Sustainability and the "Green" Site PDF
Document Details
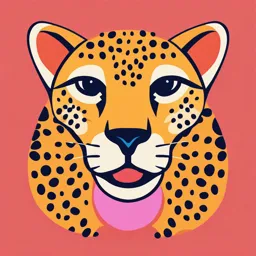
Uploaded by SwiftParadise
Tags
Summary
This chapter discusses sustainable site design, highlighting the importance of considering the environmental role of a site and its relationship with the surrounding community. It emphasizes the integration of environmental considerations with economic factors within the development process, advocating for thoughtful and intelligent development.
Full Transcript
CHAPTER 2 Sustainability and the “Green” Site T he site exists as part of a larger landscape and ecosystem, and sustainable site design must include broad considerations of the environmental role of the site as well as the program or intention of the project. Sustainable design must recognize an...
CHAPTER 2 Sustainability and the “Green” Site T he site exists as part of a larger landscape and ecosystem, and sustainable site design must include broad considerations of the environmental role of the site as well as the program or intention of the project. Sustainable design must recognize and retain as many of the functional elements of a site as possible but also consider the relationships between the site under consideration and the community at large. Change tends to come slowly to the land development process, but it does come eventually. In 2008 California became the first state to pass a statewide building code requiring water and energy conservation. A number of large cities have passed ordinances requiring green roofs, and more than 280 local governments in the United States have committed to energy conservation and greenhouse gas reductions. States are committing to green development policies for public construction, and some are offering incentives to the private sector to do the same. Many communities are requiring greener development, better street design, and more sensible use of resources and capital. These development trends embrace a sustainable environment and a robust economy, which are not mutually exclusive concepts as they are often portrayed today. Sustainability is not and cannot be antidevelopment; instead, it is the basis for thoughtful, intelligent, and rational development. Change brings many challenges. As we begin to think about sustainability and land development, it is important to note the contribution land development and construction make to our economic well-being. Nationwide about 5.5 percent of the workforce is directly employed by the construction industry, approximately 7.7 million jobs in 2005. Residential and commercial construction typically represents about 10 percent of the gross domestic product of the United States. After mortgage debt is removed, the net value of developed real estate in the United States is about $11 trillion. This does not include the value of roads or other civic infrastructure. Real estate represents the largest source of personnel wealth and savings for most Americans. Construction and development represent important economic activities. With the growth in population we can expect demand for more development: more homes and more commercial space. Studies by Woods Hole Research Center (2007) and the University of Ohio (Irwin and Bockstael, 2007) suggest that in parts of the United States development could increase by as much as 60 percent by 2030. In this same time frame, much of the infrastructure and most of the buildings in the United States will reach the end of their initial design life. Retrofitting and updating the existing “pre-green” built environment represents its own challenges and opportunities. 25 26 Chapter Two The issue is not whether we will have development but rather what that development will be. What construction methods will we employ? What materials will we use? How will the form of our designs reflect the more thoughtful and less harmful function of our projects? The impacts and issues discussed in Chap. 1 suggest that a move toward sustainable development is not only desirable but quite necessary. A plan that is concerned with sustainability cannot consider the building and the site as separate elements because they interact in important ways. This interaction is the concern of this chapter. Sustainable Development Principles Our cultural context for sustainability is in its infancy, but the dialogue is well under way. Important voices are encouraging us not to go back but forward, and to solve problems through design. Although no single set of guidelines has emerged, there is a growing recognition of the principles that lead to sustainable design and development. The groundwork for sustainable design was established by Christopher Alexander and Ian McHarg as early as the 1970s. Today the views of leaders such as William McDonough and Emory Lovins are moving into boardrooms and legislatures and are beginning to change the expectations of design professionals. This discussion of principles will necessarily be incomplete because so many efforts are occurring on so many fronts that it is impossible to capture the full range of subjects completely. The Sustainable Sites Initiative, a partnership led by the American Society of Landscape Architects, the United States Botanical Garden, and the Lady Bird Johnson Wildflower Center, is collaborating to create voluntary national guidelines and performance benchmarks for sustainable land design, construction, and maintenance practices. The initiative is a dynamic undertaking with the objective of producing a series of reports to serve the practitioner and the concerned public. Among the first of its products is the “Guidelines and Principles of Sustainable Sites.” Produced in draft form in 2008, this report provides an excellent overview and specific assessments of what should be considered first principles for site designers. The draft includes numerous case studies, summarizes measurement and analytical tools, and makes the argument for sustainable sites from a number of perspectives. These first principles are summarized in Table 2.1. The definitions of “sustainable development” are too numerous to count, and the phrase is in danger of becoming another meaningless mantra. But design professionals need to recognize the intellectual and professional challenge of finding a workable balance with nature. This is an important time for design professionals, and architects have made important advances in green buildings, although the practices are not main stream yet. Guidelines for sustainable site development practices are listed in Table 2.2, but most examples of their use are notable because they are still unusual. We must move to a marketplace of ideas and practices that make these examples unremarkable. Sustainable site planning must include considerations of the impact of development on the local ecosystem, the global ecosystem, and the future. Principles of “green site work” encourage the designer to consider the nature of the building materials, the flows of energy, and materials required for the life of the project. The designer should consider the life cycle costs of the materials being used, the ultimate disposition of the site and the materials, and ways in which these environmental impacts can be reduced or mitigated. The longer the useful life of a building or a site the longer the environment has to “amortize” the impacts, but designing a site with an extended life span requires the designer to consider future uses and changes and to incorporate that thinking into the Sustainability and the “Green” Site Do no harm Make no changes to the site that will degrade the surrounding environment. Promote projects on sites where previous disturbance or development presents an opportunity to regenerate ecosystem services through sustainable design. Precautionary principle Be cautious in making decisions that could create risk to human and environmental health. Some actions can cause irreversible damage. Examine the full range alternatives— including no action—and be open to contributions from all affected parties. Design with nature and culture Create and implant designs that are responsive to economic, environmental, and cultural conditions with respect to the local, regional, and global context. Use a decision-making hierarchy of preservation, conservation, and regeneration Maximize and mimic the benefits of ecosystem services by preserving existing environmental features, conserving resources in a sustainable manner, and regenerating lost or damaged ecosystem services. Provide regenerative systems as intergenerational equity Provide future generations with a sustainable environment supported by regenerative systems and endowed with regenerative resources. Support a living process Continuously reevaluate assumptions and values and adapt to demographic and environmental change. Use a systems thinking approach Understand and value the relationships in an ecosystem and use an approach that reflects and sustains ecosystems services; reestablish the integral and essential relationship between natural processes and human activity. Use a collaborative and ethical approach Encourage direct and open communication among colleagues, clients, manufacturers, and users to link long-term sustainability with ethical responsibility. Maintain integrity in leadership and research Implement transparent and participatory leadership, develop research with technical rigor, and communicate new findings in a clear, consistent, and timely manner. Foster environmental stewardship In all aspects of land development and management, foster an ethic of environmental stewardship—an understanding that responsible management of healthy ecosystems improves the quality of life for present and future generations. Source: “Guidelines and Principles of Sustainable Sites” 2008 draft report. The Sustainable Sites Initiative, U.S. Botanic Garden, Washington, D.C. Reprinted with permission from the Sustainable Sites Initiative Guidelines and Performance Benchmarks Draft 2008. The Sustainable Sites Initiative is an interdisciplinary effort by the American Society of Landscape Architects, the Lady Bird Johnson Wildflower Center, and the United States Botanic Garden to create voluntary national guidelines and performance benchmarks for sustainable land design, construction, and maintenance practices. TABLE 2.1 Guiding Principles of a Sustainable Site 27 28 Chapter Two 1. 2. 3. 4. 5. 6. 7. 8. Minimize cooling loads through careful building location and landscaping. Utilize renewable energy resources to meet site energy demand for lighting. Install energy-efficient lighting. Use existing buildings and infrastructure instead of developing in “greenfields.” Design to create or contribute to a sense of community. Design to reduce dependence on the automobile. Reduce material use or increase the efficiency of material use. Protect and preserve the local ecosystem. Maintain the environmental function of the site. 9. Specify low-impact. 10. Design site and buildings for longevity and to be recycled. 11. Design to minimize the use and runoff of water. 12. Minimize waste. TABLE 2.2 Guidelines for Green Site Planning and Design design. The most sustainable development is redevelopment; reuse increases density and eliminates the loss of open space. Materials should be selected on the basis of durability and low environmental impact. Recycled materials are of low impact and efficient. Better than recycling materials is the reuse of entire buildings. Many construction materials have significant environmental impacts either in the manufacturing process or in their final disposition as waste material. Others contain ozone-depleting compounds that continue to volatilize and pollute even after installation. Reducing the impact of development may be possible by reducing the footprint of a building either by modifying the footprint to the most efficient shape or by building multiple stories. Reducing the surface area of a structure will reduce energy requirements as well. Sites should be designed to treat storm water as a resource, capturing runoff and encouraging infiltration, and should use water efficiently. Use native plants suited to the local climate and precipitation and practice “dryscaping” techniques where applicable (Figs. 2.1 and 2.2). Site planning should incorporate the existing environmental function of a site to the extent possible. Wetlands and important ecosystem elements such as habitat, tree masses, and stream corridors should be preserved. The ubiquitous lawn has a notoriously high environmental impact because of the requirements for pesticides, fertilizers, need for irrigation, and continual mowing. Lawns should be minimized and replaced with native species of plants selected for their aesthetic quality and drought resistance. Buildings and tree masses can be positioned to minimize cooling costs. Green development encompasses a range of design, construction, and operational approaches that reduce the environmental impacts of development and promote human health, well-being, and productivity. Variously called sustainable design, green architecture, restorative design, or ecodesign, these approaches share the following elements: • Use of regionally available, sustainably harvested, low impact, and reused/ recycled materials • Design sensitivity to energy use, the incorporation of energy conservation, and renewable energy generation Sustainability and the “Green” Site FIGURE 2.1 The headquarters of the Chesapeake Bay Foundation is a LEED Platinum Certified building and site. Note the meadow of native plants. (See also color insert.) FIGURE 2.2 The Chesapeake Bay Foundation site includes a crushed stone parking area and native vegetation. (See also color insert.) 29 30 Chapter Two • Water conservation • Waste minimization • Incorporation of natural light, appropriate ventilation, and human scales • Design and construction techniques that evaluate and minimize environmental impacts • Site development practices that minimize and mitigate impacts More than 80 percent of all buildings in the United States were built since 1960, and the impacts of development and urbanization are well understood. As the serious environmental effects associated with greenhouse gas emissions have become known, the parts played by buildings and development have been more closely scrutinized. The Department of Energy (Battels and Burns, 2000) reports that 82 percent of the human-caused greenhouse gas emissions are energy-related carbon emissions, and of that 48 percent of the increase in emissions since 1990 is attributable to increasing emissions from the building sector. In fact, buildings (residential and commercial) are responsible for more greenhouse gas emissions than either the industrial or transportation sectors. Energy efficiency is a central concern of green development, and 50 percent of U.S. electricity is generated by burning coal, the least efficient and dirtiest of the major fuel sources. (Energy efficiency is measured as the amount of energy expended for each unit of gross domestic product.) Since the 1970s, the energy efficiency of U.S. buildings has remained flat, but the number of buildings has grown exponentially. Since the early 1990s, the European Union (EU) has sought to increase energy efficiency across the board, and in 2002 the EU passed rules requiring efficiency in commercial and government buildings. The contemporary office building in the EU is four times more energy efficient than its counterpart in the United States (Fig. 2.3). In addition to these environmental advantages, the EU building is 75 percent less expensive to operate. Energy efficiency in buildings can be improved through the use of natural light, building orientation, and material selection. Green buildings are healthier and more productive spaces than traditional construction. The increased natural light is among the commonly appreciated features found in green buildings. Careful selection of materials such as floor and wall coverings and the design of a building to passively move air can contribute to cleaner indoor air. As global energy supplies tighten due to increased demand and pressure mounts to respond to global climate change, institutions and business can take a positive step through green building. The costs of green buildings are typically no different or only nominally higher than traditional construction, and the life cycle savings and operation costs are significant. Lower energy costs and reduced water use and waste generation are the most common areas of savings. Green buildings are healthier and constitute a more desirable working and learning space. Measuring Performance Some buildings are considered “high performing” because of energy efficiencies, but these buildings are generally not “green” in a strict sense. Green buildings exist on a continuum ranging from fairly straightforward attempts to reduce the environmental footprint of a building to very sophisticated structures. Several guidelines have emerged to guide and measure the process of designing, building, and operating a green building. Sustainability and the “Green” Site FIGURE 2.3 A green roof, which reduces storm water runoff and rooftop albedo and increases energy efficiency. (Source: Image courtesy of Roofscapes, Inc. See also color insert.) The National Institute of Standards and Technology (NIST) developed the Building for Economic and Environmental Sustainability (BEES) model with support from the EPA and HUD. This approach evaluates the life cycle costs and environmental impacts of building materials in 10 areas of concern, which enables designers or builders to determine the environmental loading of a material before they specify its use. The American Society for Testing and Materials (ASTM) has also developed an approach for evaluating the life cycle costs of building materials (Standard Guide for Environmental Life Cycle Assessment of Building Materials/Products, E 1991–98). This is a broad-based approach that includes consideration of embodied energy, raw materials acquisition, and environmental impacts. The best-known approach is the U.S. Green Building Council’s Leadership in Energy and Environmental Design (LEED) program. This comprehensive approach is chief among the reasons LEED-certified buildings have been shown to have significantly lower operating costs and healthier and more productive occupants in addition to protecting the environment. Unlike BEES and the ASTM method, LEED does not certify materials or processes, although these standards might be used by designers specifying for a LEED project. The U.S. Green Building Council (GBC) created and oversees the LEED program, and in 2009 several notable changes were undertaken. First, the LEED certification process was moved from the GBC to the Green Building Certification Institute, which was created for this purpose and gives the certification process a degree of third-party credibility. The second significant change was adoption of the LEED 2009 update in November 2008, which provides for regional credits that reflect issues that may be of 31 32 Chapter Two greater concern in a given area. The program provides greater weight for credits that reflect concern with climate change and energy efficiency. The LEED process is a consensus standard, and changes reflect a process of collecting input from across the building industry, reiterative comment periods, and a final vote by about 18,000 member organizations. LEED certified professionals are employed in the planning, design, and construction processes. Projects are evaluated in five areas of concern: • Site design and planning • Energy use • Water management • Materials resources and waste • Indoor environmental quality Specific guidelines are published for each area, and a Green Building Rating System describes how design choices will be rated in terms of LEED points. A detailed review of the rating system is beyond the scope of this book, but a LEED certified building generally will utilize the following considerations: • Consideration of building position for solar access and wind direction, with regard to energy impacts • Materials selection subject to environmental and life cycle considerations • Design for energy efficiency and performance • Design and select materials and equipment with consideration for high-quality indoor air • Minimize waste in construction and operations • Weigh capital costs savings against operation cost savings • Use of published measurement strategies such as LEED Sustainable site concerns include the following: • Landscaping for energy efficiency • Rethinking grounds maintenance to eliminate toxins • Reducing water consumption through design, plant selection, and maintenance practices • Being aware of site specific concerns (e.g., soil limitations) • Accommodating access, possibly including public transportation • Using site features to contribute to building performance • Locating buildings with regard to solar and seasonal influence • Maximizing local energy alternatives Benefits to green buildings extend beyond the environmental performance of the building. Green buildings have been found to increase worker and student productivity as much as 6 to 12 percent. Such significant improvements have been made in Sustainability and the “Green” Site worker productivity in these high-performing buildings that companies almost cannot afford not to build green! Similar improvements have been seen in less worker turnover (Fahet, 2005). The nature of the activities in buildings is so variable that it is difficult to draw meaning from broad claims. By looking at a narrower type of building, we may be able to cautiously draw some general conclusions regarding some advantages of green buildings and sites. A sufficient number of green school buildings have been constructed to provide a basis of study. Simply put, performance improves in green schools. Studies have found that indoor air quality and classroom lighting have direct effects on both teacher and student performance (Heschong Mahone Group, 1999). Green buildings commonly feature significant day lighting, and the character of day lighting is carefully considered and controlled. The quality of air and the acoustics within the classroom also have a direct influence on student learning. The available studies focused on elementary and secondary schools, and the results are notable. For example, improvements in lighting alone were correlated with 7 to 18 percent higher scores in the end-of-year examinations over classrooms without such improvements. Students in the improved classrooms also did 20 percent better on math exams and 26 percent better on reading tests. This suggests that a deeper understanding of building environments is important. Capital Cost Implications The data on the cost of green buildings are difficult to synthesize or reduce to any specific building project. In general, it is more cost-effective to build green ideas into a new building or site, although retrofitting offers advantages in some cases. Cost comparisons are difficult to assess because true or life cycle costs of traditional construction are rarely accounted for. For example, green materials tend to be more durable than traditional construction. This may result in higher construction or development costs but a much longer life cycle and fewer maintenance costs. Since capital costs and operational costs are not usually considered together, the life cycle costs cannot be compared. In general, green construction is cost competitive for capital projects and is more cost-efficient from the operations standpoint. Figures routinely used in the literature suggest that green architecture increases construction costs 2 to 11 percent (Kats et al., 2003). Among the most comprehensive studies of the cost of green buildings was the 2004 Davis Langdon study, which compared the costs of green architecture to traditional architecture from two perspectives: (1) what would the cost difference be between a traditional building and the same building as a green building, and (2) a direct comparison of 45 buildings seeking LEED certification and 93 buildings not seeking any green certification. LEED uses a point system to guide choices that range from building location and orientation, building materials, landscaping, water and energy conservation, and light pollution to transportation. Eligible buildings may apply for and be recognized as Certified or Silver, Gold, or Platinum Certified, depending on the number of points they achieve (see Fig. 2.1). In general the Davis Langdon study drew the following conclusions: • Most green buildings can meet the goals of LEED within or nearly within the original program budget. • Many non-LEED buildings included green elements for purposes of operational efficiency without specific regard to environmental performance or certification. 33 34 Chapter Two • The key to the cost performance of green buildings lies in the program planning phase, the expertise of the project team, and project management/oversight (Davis Langdon, 2004). The costs of green buildings have also been evaluated in a study commissioned by the U.S. General Services Administration (GSA). The 2004 study evaluated the costs for buildings seeking Certified, Silver, and Gold LEED ratings. The study only considered courthouses and building modernization but concluded costs may range widely depending on the rating being sought. In cases where low or no cost features are used, the study found “the overall cost premium [to be] surprisingly limited, even at higher rating levels. Under certain conditions it is possible to show a slight decrease overall” (Steven Winter Associates, 2004). These and other studies demonstrate that the capital costs of green buildings are often no more or only nominally more than those for traditional buildings. The Davis Langdon (2004) study strongly suggests that cost performance can be related to management of the process from planning through construction. Other studies have indicated that although costs may rise slightly as greater efficiency is designed into buildings, the rate of savings increases more dramatically. Operating Costs Operation cost data are highly variable, but green building savings are most often associated with lower energy costs. Electricity cost savings are in the range of 45 to 55 percent. Some sophisticated energy conservation systems have a payback period of 7 to 9 years, but more common energy efficiency features have a payback of less than 2 years. Kats et al. (2003) looked specifically at public buildings and found that the median payback period for increased capital cost was 2.6 years or less. High-performing buildings saved, on average, $0.87 per square foot per year in lower energy costs, and 3 percent of the savings was derived from more efficient lighting and load responsive HVAC equipment. Water use can be reduced by as much as 90 percent, but savings of around 50 percent are more common. Among the chief advantages to green buildings are the excellent life cycle costs. It is estimated that the life cycle savings of the typical green building is 10 times the initial investment. That is, for every $100,000 in initial capital investment, a savings of $1 million is achieved over the life of the building (Weidt Group, 2005). Green Building Materials The choice of building materials is as important as the site design or choice of construction methods. Designers have significant influence over the materials used through the specifications made in the design and planning. Many designers are not aware of the implications of choosing one material over another beyond cost and specific performance criteria. Site designers have fewer material choices than architects, but awareness of site materials is just as important. As a matter of practice, materials should be selected in part because of their durability. The process of manufacturing materials is energy and material intensive, and durable materials usually require less maintenance over a longer service life. Materials that require less maintenance or maintenance that has a lower environmental impact are preferred. Heavily processed or manufactured Sustainability and the “Green” Site materials have a higher embodied energy; that is, greater energy inputs are required to manufacture the product than a material with a lower embodied energy. Locally produced products require less transportation energy and produce less pollution. Designers should seek durable, locally produced, low-maintenance products with low embodied energy ratings. For example, local hardwoods are preferable to tropical woods, and local stone to imported stone. The best choice for materials may be recycled materials. Using recycled materials reduces solid waste, eliminates the energy of manufacturing, and reduces the impact on natural resources. Some ways of using recycled materials in site work include specifying the use of fly ash in concrete, furniture made from recycled plastic, and pavement containing ground tires. Avoid materials with toxins such as pressure treated lumber by specifying alternatives such as recycled plastic lumber. Choosing a green building material involves consideration of the entire life cycle of the material: the manufacture of the material, the impacts of its use, its distribution and service life, and finally its disposal. Every stage of the material’s life involves energy use and environmental impacts. The BEES life cycle assessment model evaluates 10 impacts of building materials: 1. Global warming potential 2. Acidification potential 3. Eutrophication potential 4. Natural resource depletion 5. Indoor air quality impacts 6. Solid waste impacts 7. Smog 8. Ecological toxicity 9. Human toxicity 10. Ozone depletion Each of the calculations involves converting impacts to a known and given reference point provided in the BEES documentation. The program then calculates the environmental loading of the product to allow designers to compare alternative materials. BEES software is available from the National Institute of Standards and Technology (NIST) along with a manual that describes the use of the software, explains the algorithms, and provides examples of material and product data already evaluated using BEES. ASTM Standard Guide for Environmental Life Cycle Assessment of Building Materials/Products (E 1991–98) describes a four-step process for conducting a Life Cycle Assessment (LCA): definition of goals, analysis of inventory, impact assessment, and interpretation of findings. The LCA is comprehensive and includes considerations of embodied energy, raw materials acquisition, environmental impacts from cradle to grave, and performance. Other more approachable methods have also emerged through public and private green building initiatives throughout the world. Many of these organizations have established standards or thresholds that products must meet to be listed as “green.” Most of the work has been done on materials used in buildings, but materials used in site development are not without their environmental “signature.” The general requirements for green building materials are summarized in Table 2.3. 35 36 Chapter Two 1. Products made from recycled or salvaged materials. 2. Products made from wood harvested from Forest Stewardship Council certified forests. 3. Products made from materials that are renewable in the short term (10 years or less). 4. Products that do not contain toxics or environmentally damaging materials. 5. Products (or methods) that reduce the material volume required. 6. Products that reduce environmental impacts during the manufacturing process, construction, renovation, or demolition. 7. Products (or methods) that are energy efficient or that reduce the heating and cooling loads on a building. 8. Products that are reusable or recyclable. 9. Local products rather than products from far away. TABLE 2.3 Green Building Material Requirements The American Society for Testing and Materials (ASTM) Subcommittee on Sustainability has developed a Standard Practice for Data Collection for Sustainability Assessment of Building Products (E-2129). This standard includes a checklist to guide the process of evaluating the environmental character of products. Most of the processed or manufactured materials specified in site work are related to paving, utility, or storm water pipes. Even with these few categories of materials, a wide range of choices is available for designers to consider. Pipe Materials Pipes are selected primarily for storm water drainage, sanitary sewage conveyance, or the distribution of water. Water systems commonly required ductile iron or steel pipe, but there are many choices of material for storm water and sewage collection. Selecting pipe materials might be a matter of complying with local ordinance or preference, but selecting materials should also involve an understanding of the costs and benefits of the possible choices. ABS ABS (acrylonitrile-butadienne-styrene) is used primarily as waste and storm water pipe. ABS is lighter than PVC but is more than twice as expensive. ABS has almost twice the thermal expansion of PVC, but the resin material from which it is made is expensive to manufacture. ABS manufacturing involves a number of toxic materials and environmental impacts, and instances of off-quality material in the marketplace have resulted in failures in the field. Cast Iron Many building codes still require cast iron pipe, but these tend to be more a reflection of political and economic pressures than the value of the material itself. Cast iron is durable and has a low thermal expansion coefficient, but its great weight and associated labor costs would seem to offset those values. Cast iron is no more durable than PVC, for example, and the energy and environmental impacts of cast iron pipe manufacture are quite high. Sustainability and the “Green” Site Concrete Pipe Although very durable and resistant to wear, concrete pipe is heavy and expensive to install. It is still required in some local and state codes because of its durability. The strength of concrete makes it useful in applications in which there is minimal cover or where significant loads are expected. HDPE HDPE (high-density polyethylene) is the lowest cost, lightest, and most flexible of the pipe materials. HDPE is relatively simple to manufacture, and it is the most easily recycled pipe material. It is manufactured in long sections familiar as the coils of pipe material often used to reline old pipelines and sewers. For all of its positive characteristics, HDPE suffers from the greatest expansion coefficient of any of the popular pipe materials— more than twice the thermal expansion of PVC—which limits is usefulness for many applications. PVC PVC (polyvinyl chloride) is widely used because it is high strength, lightweight, low cost, easy to work with, and durable. It is used in a wide array of products, but for site concerns it is used primarily as pipe or site furniture. About 60 percent of the PVC in the United States is used in the construction industry. Available pipe diameters in PVC range from 1/8 inch to 36 inch. Nearly all wastewater sewers constructed in the United States today are built of PVC pipe. Manufacturing PVC has some environmental costs. Vinyl chloride is a carcinogen produced from ethylene and chlorine. PVC manufacturing produces about 4.6 million pounds of vinyl chloride emissions each year. PVC manufacturing has been associated with the presence of dioxin in the environment, but research has not established a clear risk associated with the quantities observed. More dioxin, one of the most toxic substances known, is produced when PVC is burned, however. Some concerns associated with the decomposition of PVC are associated primarily with architectural or electrical uses of plasticized PVC and do not appear to be relevant to the exterior site applications of the material. PVC is difficult to recycle; a wide range of formulations are used in different products, making it difficult to use the material in the manufacture of postconsumer goods. Incinerating PVC is problematic because it has low fuel value and it turns into hydrochloric acid as it burns, increasing the wear on incinerators. Many products made of PVC have formulations that include lead and other toxins. Although these products are not usually associated with site development applications, there is noteworthy concern about the environmental costs and impacts of PVC manufacturing, use, and disposal. Calls for stopping the manufacture of PVC because of these concerns have been raised. Vitrified Clay Pipe Vitrified clay pipe (VCP) has been largely replaced by the use of PVC, but it still has some applications. Many VCP installations have been in use well over a hundred years. It is durable, resistant to chemical corrosion, and has the lowest thermal expansion coefficient of any pipe material. The weight of VCP (8.9 lbs per ft for a 4-in. VCP versus 2.0 lbs. per ft for a 4-in. Schedule 40 PVC) leads to more handling and greater labor 37 38 Chapter Two costs for installation. As PVC has become the material of choice, the availability of VCP has dropped in some areas. Recycled Plastic Pipe Recycled plastic pipe (RPP) is available as a stock item in Canada, Australia, and Europe but usually requires a custom order in the United States. Early attempts at RPP were reported to suffer from a number of quality issues ranging from variations in material hardness to load bearing capacity. As with many products, quality has improved with experience. RPP offers an opportunity to reuse plastics that might otherwise be dumped in a landfill or burned. The pipe is manufactured in corrugated and smooth surfaces for use as storm water and sewer pipe. Cement and Concrete Concrete is widely used in all types of construction because of its ability to be cast in a desired form and its durability once it is cured. Cement manufacturing and concrete mixing is a large business sector involving about 210 cement plants and almost 5000 ready-mix plants in the United States. Most ready-mix concrete for residential purposes is approximately 12 percent cement. The most common cement used is Portland cement. Manufacturing cement involves mixing a source of calcium (usually limestone) with finely ground additives (such as bauxite or iron ore) in a rotary kiln heated to about 2700°F (1480°C). As the kiln mixes the heated materials, a series of chemical reactions occurs. The materials form a molten mass that is cooled and then ground to a powder, which is mixed with some gypsum to become cement. The cement is mixed with sand, aggregate, water, and possible admixtures specified to control setting time or plasticity of the final material. Environmental Considerations The raw materials of cement are common enough. It takes about 3400 pounds of raw material to produce 2000 pounds of finished concrete. The most significant environmental impacts of cement manufacturing and concrete use are the amount of energy consumed, the energy-associated emissions of carbon dioxide and other greenhouse and acid-forming pollutants, the dust that results from the manufacturing process, the pollution impact on surface waters from run-off, and “washout water.” Manufacturing cement is an energy-intensive process involving burning fossil fuels to generate the high temperatures of the rotary kiln. Some cement plants have been converted to burn hazardous wastes or other solid waste to extract the energy value. The high temperature of the kiln provides fairly complete combustion with low levels of residual air pollution. According to the Portland Cement Association, a single cement kiln can consume more than a million tires each year. Other elements of concrete do not require the substantial energy inputs of cement manufacturing, and the use of fly ash in concrete reduces the energy load even more (Cockram, 2006). In addition to the energy costs, there are environmental impacts associated with fugitive dusts. The EPA estimates that for every ton of cement manufactured 360 pounds of alkaline dust is generated. Much of this dust is generated during the manufacturing process, but some occurs in handling and transporting the cement and in the mixing Sustainability and the “Green” Site process. At the cement manufacturing plant, much of the dust is captured in baghouses or by other pollution control equipment. Some of the dust is used for agricultural soil amendments, but much of it is used for landfill. Dust generated at ready-mix facilities and construction sites generally is not controlled. The alkaline character of cement may result in runoff or washout water with a pH as high as 12. High alkalinity is particularly harmful to aquatic life. Runoff from most concrete and ready-mix sites requires a surface water discharge permit. Washout on construction sites should be properly collected and managed on site. Fly Ash Concrete Fly ash is a residual by-product of burning coal that has become a common substitute for Portland cement in concrete. Fly ash is produced in the generation of electricity and industrial processes. In the past, fly ash was used for a variety of purposes but most commonly as landfill. Replacing Portland cement with fly ash, or using the two in combination, reduces the amount of Portland cement required, offsetting the environmental costs to some degree. The advantages of using fly ash are well documented. Fly ash concrete is stronger, although it may take longer for strength to develop. Fly ash concrete tends to increase the time it takes for concrete to set. An advantage in the summer, allowing longer working times, this may be a disadvantage in the winter. Concrete mixes can be adjusted for weather conditions. Local ready-mix plants can provide mixtures that are seasonally adjusted to a given area. The time for strength to develop can be reduced to be comparable to Portland cement if a fly ash/Portland cement mixture is used (15 to 30 percent fly ash). Fly ash concrete requires less water per unit of volume, which reduces shrinking and cracking. Fly ash concrete may not accept color dies or acid finishes with the same results as Portland cement concrete. Environmental Strategies for Concrete Use The key to the wise use of concrete begins with proper specification of materials and estimates of volumes. Alternative designs or products that minimize the amount of material necessary may be possible. Precast products, for example, may use less material than cast-in-place alternatives and reduces on-site waste. Specifying fly ash concrete or fly ash/Portland mixtures can improve strength and reduce the amount of material required. Solid waste that may be produced can be crushed and used as fill. Arrangements should be made to collect washout water and to control runoff from such areas. Recycling Asphalt Paving Recycling paving involves milling the top surface of a roadway or parking lot and removing as little as three-quarters of an inch to as much as three or four inches of the pavement. There are several methods for recycling the removed material. Recycling may be done in place, crushing the milled materials and mixing them with new asphalt emulsion and perhaps new asphalt materials and repaving the surface. This is often done using a “train” of equipment to mill, crush and mix, and repave the road in a continuous “ribbon.” In other cases the material is transported off-site to a plant where remixing occurs. In some cases the pavement is heated during repaving to soften the 39 40 Chapter Two material and bond the new surface. Recycling paving is a very cost-efficient approach. Other recycled materials may be used as road base material. For more information on paving, see discussions in Chaps. 4 and 6. Treated Lumber Wood is widely used in landscaping, and in most contemporary applications treated wood is specified because it lasts up to 30 times longer than untreated lumber. It could be argued that the extended service life helps to save trees that would otherwise be harvested and that this offsets the environmental problems associated with treated wood. In the past wood was treated primarily with creosote, essentially a coal tar distillate, but creosote treated wood is less common today. The remaining wood preservatives fall into two categories: oil-based preservatives and water-based preservatives (Table 2.4). Some concerns with using treated wood include whether the material will come into contact with people or animals or any water body, including groundwater. Alternative materials should be considered if the treated wood is to come into direct contact with food supplies. Treated lumber should not be used where it will come into direct contact with drinking water, although federal guidelines allow for incidental uses such as for docks and bridges. The type of treated lumber should be carefully considered for use in playground equipment or picnic facilities; creosote and penta should not be used for these purposes. Disposing of treated wood is difficult; it is, after all, treated to resist decomposition. Ideally waste wood should be recycled, but it should not be composted. Some states prohibit burning treated wood. Ultimately, using treated wood is a commitment to using toxic materials in the site. The belief that pressure treated wood will last a very long time reflects a successful marketing strategy more than a fact. A technical report (McQueen and Stevens, 1998) indicates that the average life of a pressure treated deck is only about 9 years. Treated wood may be insect resistant and perhaps rot resistant, but it is not moisture resistant. Repeated cycles of wetting and drying inevitably begin to warp and split the wood, and the structure slowly tears itself apart. As the structure is replaced or rebuilt, the treated lumber cannot be recycled, composted, or burned. The specifier should be at least as concerned with moisture resistance if durability is a concern. Redwood, cedar, and cypress are commonly Preservative Type Character Creosote Oil Restricted use only Pentachlorophenol (penta) Oil Teratogenic properties; restricted use only Copper napthenate Oil Chromated copper arsenate (CCA) Water Pressure treatment only; contains arsenic and chromium Ammoniacal copper quaternary compound (ACQ) water Pressure treatment only; does not use toxics arsenic and chromium TABLE 2.4 Types of Common Wood Preservatives Sustainability and the “Green” Site thought of as rot resistant woods, but in fact only the heartwoods of these trees are demonstrably rot and moisture resistant. Even heartwood does not generally outperform treated lumber. If treated wood is to be used, the best option for the environment is ACQ, but consideration should be given to specifying rot resistant species from native trees or recycled plastic lumber. Deconstruction With interest in sustainability has come an emerging marketplace for reusable or recycled materials and the infrastructure for finding and selling such materials. This, in turn, has led to a revitalized deconstruction industry. The industry is still at the early stages of developing the methods and practices of building deconstruction, materials salvage, and management of abandoned and vacant property. This may be a natural extension of the 1990s brownfield movement that focused on finding effective uses for abandoned and environmentally unsafe properties, but it points to the potential for a marketplace for recycled materials. Abandoned or condemned homes may contribute to a loss of local property values and reduce the desirability of otherwise strong neighborhoods (Fig. 2.4). In many cities such structures are routinely razed. Removing valuable materials such as flooring, windows, doors, trim, and fixtures allow these items to live again in new or remodeled buildings. Deconstructing buildings saves useful materials for reuse in new construction and rehabilitation projects and creates more jobs and business opportunities than demolition (Fig. 2.5). Even buildings with minimal potential for rehabilitation may be valuable sources of useful building materials. The process of deconstruction may have more value to architects than to site designers, but many repositories of recovered material include pavers, stone stairs and curbs, planters, exterior lighting, exterior metal work, and other materials that may be useful (Fig. 2.6). The challenge is that the designer must become familiar with the deconstruction practitioners in her marketplace. Confirming that materials are recycled or contain recycled materials may be a necessary element in establishing a site or building that is sustainably designed. A number of organizations provide certifications of one sort or another. For example, Scientific Certification Systems (SCS) rose out of the need for such a system created by the U.S. Green Building Council’s LEED program. The focus is largely on finishes and building elements as opposed to site elements, but SCS does certify wood, cement, rock, and other materials used in site work. Paving operations have been using alternative and recycled materials for years. Specifications for the use of recycled material as aggregate in paving are available from most state highway departments. The use of recycled materials for LEED certification may require some life cycle information or documentation of origin. SCS does certify manufactured products that contain a minimum percentage of recycled materials to qualify for LEED; however, there is no formal process for documenting or certifying building materials recovered by deconstruction for reuse. As interest in green building grows and more owners become interested in LEED certifications, such a system may be developed. Individuals engaged in deconstruction confirm that no system is currently in place nationwide. A notable exception to this is “The Reuse People” (TRP), with headquarters in Oakland, California. TRP typically completes an inventory of materials prior to 41 42 Chapter Two FIGURE 2.4 The presence of abandoned houses may lead to unwanted activities and reduce both the value and livability of neighborhoods. deconstruction. To comply with Internal Revenue Service (IRS) regulations, they require an independent appraisal to determine the value of donated materials. On donations of $5,000 or more, their system tracks material from deconstruction to the point of sale or reuse to meet IRS requirements. Items are labeled with a unique job and piece number as the deconstruction proceeds. Materials of many pieces such as bricks or lumber are gathered in lots and inventoried, then given a unique lot number. These identifiers are maintained in an inventory database. Each lot can be traced to a specific project or source, providing a chain of custody and perhaps the needed documentation for certification. The cost of the inventory and tracking system for a typical residential deconstruction is estimated to be $1.25 to $1.50 per square foot of building. As building size increases—for example, in commercial buildings—the cost per foot decreases (Ted Reiff, personal communication). The additional cost of this Sustainability and the “Green” Site FIGURE 2.5 Site deconstruction. FIGURE 2.6 Workers engaged in the deconstruction of a house. The reuse of valuable building materials is an important aspect of sustainable architecture. (Source: Image provided by and used with the permission of Second Chance, Inc., Baltimore, MD. See also color insert.) 43 44 Chapter Two inventory may be offset by an increased value for reuse materials whose origin can be documented. Sustainable Site Planning Among the first considerations for the site planner is to determine how to minimize the disturbed area necessary for the project. Key aspects of minimizing the disturbed area are the degree to which habitat and open space are preserved and created. Not all open space is equal in this concern: athletic fields, for example, are not considered to be habitat or open space for purposes of sustainability, but a green roof might be. Strategies for minimizing the disturbed area include clustering buildings, increasing density, and encouraging smaller building footprints. Clustering buildings reduces the disturbed area and allows the designer to minimize road length and paving. Recognizing that the disturbance of the site affects the landscape well beyond the property line, the designer looks for ways to maintain or reestablish links to other parts of the landscape ecosystem through the building location decision. Care should be taken to protect stream corridors, wetlands, and other unique or important landscape features. Storm water management is a key component of sustainable site design. This is discussed more fully in Chap. 8. Building Location The location of a building on the site is a critical element of site planning. The building should be located to minimize the impact on the site while maximizing the function and quality of the designed space. Selecting a location is a combination of managing the solar influences of the site, balancing the earthwork, and maximizing the utility and aesthetics of the site. Locating proposed structures offers the designer the first opportunity to focus the design of the site in a sustainable direction. The building location fixes the limits and extent of disturbance. Building location has important implications for the energy costs of heating and cooling. For northern areas, buildings should be located on the portion of the site that receives the most light during the hours of greatest sunshine, particularly in the winter months—about 9:00 a.m. to 3:00 p.m. The building should be located in the northern most part of this area, but allowances should be made for adequate distance from neighboring properties to allow for possible shading due to future development. Open space should be located on the southern side of the building. Open space with a southern exposure is preferred over open space with a northern exposure. Building shape may be more important than orientation, but site designers may not have influence over the shape of the building. Square buildings are inefficient shapes for heating and cooling, although they tend to be more efficient than a long, narrow building on a north-south axis. The best combination of shape and orientation is an elongated building on an east-west axis. In northern latitudes in winter, buildings orientated on an east-west axis receive almost three times as much solar radiation on the south side of the building as on the east or west. This situation is reversed in the summer. The location and shape of buildings can be managed to reduce the site albedo and the heat island effect created by development. The use of vegetated roofs can further reduce these effects. Sustainability and the “Green” Site Site Maintenance Considerations The operating and maintenance costs of a site are a reflection of the design. Reducing the need for additional watering and reducing the chemical inputs needed to maintain a landscape are critical concerns. Site designers are well versed in the elements of design needed to facilitate traffic, pedestrian movement, and the functional and aesthetic aspects of a site; but sustainability adds to these elements. Sustainability may require smaller turf areas and more reliance on native species of plants. The designer should have some local climate knowledge and an understanding of the microclimates that will be created in the site. It is likely that a working knowledge of local soils will need to be supplemented with specific soil tests to ascertain the right plant selection to minimize reoccurring inputs. The choice of plant species and materials used on the site can make important contributions to the environmental performance of the site. From trees providing cooling shade to buildings and parking lots, reducing site albedo and heat generation and retention help reduce energy demand. Reduced turf area and increased use of meadows and natural vegetation increase biodiversity on the site. Selecting plants that form natural communities and have supporting interactions will reduce the need for fertilizer and pesticides. The use of design to capture and retain precipitation will lower the costs of infrastructure and reduce the need for additional watering. Designers might consider the use of nonpotable water for landscape watering either by using on-site retention ponds or innovative wastewater systems. More information on these issues is provided in subsequent chapters. Moving to Sustainability Sustainability concerns go beyond the selection of materials. The layout of a site, the types and character of groundcover, and the management of the various landscape functions are critical issues. All of these issues have implications for site planners and designers. First, what is the role of site development in contributing to these effects, and how might those effects best be mitigated? Second, given that these decisions will influence the use and function of a site, how do we account for these changes in planning and design? Site planning, design, and development professionals are moving toward including sustainability as a matter of practice. It is up to the planner and the designer to synthesize all of the issues and interests and then educate the parties about the value of the plan and the design. To include issues of sustainability, the planner and designer should become students of those subjects as much as the other subjects that are incorporated in a site plan. The site planning and design professions are embracing the concerns with sustainability as we grow more aware of issues of climate change, energy, and the anticipated pressures of continued population growth. More to the point, communities and clients are also requiring more sensitive and intelligent design. The opportunities to address these concerns are expected to grow in years to come. Although much has been done to improve our understanding of our relationship with the environment, much more remains to be done; the design paradigms of the future are just now being formed. Begin to consider issues for site and community design that go beyond mitigating the impacts of site disturbance and the disruption of environmental services. As 45 46 Chapter Two concern with energy conservation and efficiency grow, transit planning and local sources of energy are likely to become important to communities. Likewise, concern over quality of life issues may lead to a greater premium on communities that provide more manageable commutes or to walkable communities. In addition, access to local food and other resources may become important elements in the sustainable community of the near future.