Week 4 SCMMA2 P1 PDF
Document Details
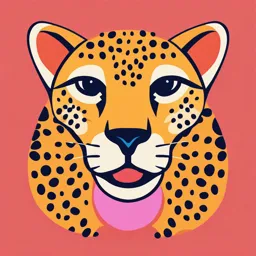
Uploaded by SuperbGermanium1022
Tags
Summary
This document discusses the use of monoclonal antibodies in various applications, including diagnostics and treatments for diseases. It explains how these antibodies are produced, their characteristics, and different types. The summary also explores the mechanism of action of various drugs that use monoclonal antibodies.
Full Transcript
Use of Monoclonal Antibodies As soon as it was determined that antibodies were produced by B cells, it was understood that if a B cell producing a single type of antibody could be isolated and cultivated, it would be able to produce the desired antibody in nearly unlimited quantities and without co...
Use of Monoclonal Antibodies As soon as it was determined that antibodies were produced by B cells, it was understood that if a B cell producing a single type of antibody could be isolated and cultivated, it would be able to produce the desired antibody in nearly unlimited quantities and without contamination by other antibodies. Unfortunately, a B cell reproduces only a few times under the normal cell culture conditions. This problem was largely solved by tapping cancerous plasma B cells for culture. These cancerous plasma B cells, known as myelomas, no longer make antibodies but can be isolated and grown indefinitely in cell culture. Combining an “immortal” cancerous B cell with an antibody-producing normal B cell creates a hybridoma that, when grown in culture, produces the type of antibody characteristic of the ancestral B cell indefinitely. This allows us to procure immense quantities of identical antibody molecules. Because all of these antibody molecules are produced by a single hybridoma clone, they are called monoclonal antibodies, or Mabs (Figure 18.2). Monoclonal antibodies are uniform, are highly specific, and can be produced in large quantities. Because of these qualities, they are enormously important diagnostic tools. Commercial kits use them to recognize several bacterial pathogens, and nonprescription pregnancy tests use monoclonal antibodies to indicate the presence of a hormone excreted only in the urine of a pregnant woman (see Figure 18.13, page 543). Monoclonal antibodies have also become a clinically important, frequently used class of drugs. Currently more than 62 have been approved for human therapy. These include treatments for multiple sclerosis, Crohn’s disease, psoriasis, cancer, asthma, and arthritis. There are hundreds more drugs of this type currently being developed worldwide for a wide range of diseases and conditions. The modes of therapeutic action of monoclonal antibodies vary. Certain inflammatory diseases such as rheumatoid arthritis require the action of tumor necrosis factor (TNF; see page 485). Mabs that neutralize TNF block the progression of the disease. One such Mab is infliximab. Other Mabs block a receptor site; an example is omalizumab. This drug treats allergic asthma by preventing the binding of IgE on mast cells and basophils (see Figure 19.1 on page 552). The Mab rituximab is used to treat inflammatory diseases that the TNF-blocking Mabs cannot. This class of Mab binds to antigen-presenting cells, depleting their supply and thereby blocking the progression of the disease. The therapeutic use of monocolonal antibodies had been limited because they were once produced only by mouse (murine) cells. The immune systems of some patients reacted against the foreign mouse proteins, leading to rashes, swelling, and even occasional kidney failure, plus the destruction of the antibodies. New generations of monoclonal antibodies aim to minimize the murine component, so they are less likely to cause side effects. Essentially, the more human the antibody, the more successful it is likely to be. Researchers are exploring several approaches. Chimeric monoclonal antibodies use genetically modified mice to make a human–murine hybrid. (A chimera is an animal or tissue made up of elements derived from genetically distinct individuals.) The variable part of the antibody molecule, which includes the antigenbinding sites (see Figure 17.4a on page 506), is murine. The constant region of the antibody molecule is derived from a human source. These monoclonal antibodies are about 66% human. An example is rituximab, which treats leukemias and some autoimmune disorders that are characterized by excessive numbers of B cells. Humanized antibodies are constructed so the murine portion is limited d to antigenbinding sites, with about 90% of the rest of the molecule being derived from human sources. An example is trastuzumab (Herceptin®), used to treat breast cancer in patients with a certain gene mutation. Even humanized antibodies can cause unwanted immune responses, which has spurred research and development of fully human antibodies. One approach has been to genetically modify mice so they contain human antibody genes. An early fully human example is adalimumab, used to treat rheumatoid arthritis and psoriatic arthritis. Most types of monoclonal antibodies end in mab; the letter(s) directly before that indicate derivation. Umab means human-derived, omab are from mice, ximab are chimera, and zumab are humanized. A name may also reference the particular disease or tumor being treated. For instance, biciromab are monoclonal antibodies derived from mice that treat a cardiovascular condition (cir being short for circulatory system). Mabs are used in the diagnostic tests described throughout the rest of this chapter Precipitation reactions involve the reaction of soluble antigens with IgG or IgM antibodies. Precipitation reactions occur in two stages. Within seconds, the antigens and antibodies rapidly form small antigen–antibody complexes. Next, over the course of minutes or hours, the antigen– antibody complexes form larger, interlocking molecular aggregates called lattices that precipitate from the solution. Precipitation reactions normally occur only when the ratio of antigen to antibody is optimal. Figure 18.3 shows that no visible precipitate forms when either component is in excess. The optimal ratio can be achieved when separate solutions of antigen and antibody are placed adjacent to each other in agar gel or in solution. If they are allowed to diffuse together, an immunodiffusion test, or precipitin ring test Figure 18.4), will show a cloudy line of precipitation in the area in which the optimal ratio has been reached (the zone of equivalence). Other tests use electrophoresis to speed up the movement of antigen and antibody in a gel, sometimes in less than an hour, with this method. The techniques of immunodiffusion and electrophoresis can be combined in a procedure called immunoelectrophoresis. The procedure is used in research to separate proteins in human serum and is the basis of certain diagnostic tests. It is an essential part of the Western blot test used in AIDS testing Whereas precipitation reactions involve soluble antigens, agglutination reactions involve either particulate antigens (particles such as cells that carry antigenic molecules) or soluble antigens adhering to particles. These antigens can be linked together by antibodies to form visible aggregates, a reaction called agglutination (Figure 18.5). Agglutination reactions are very sensitive, relatively easy to read (see Figure 10.11, page 319), and available in great variety. Agglutination tests are classified as either direct or indirect. Direct Agglutination Tests Direct agglutination tests detect antibodies against relatively large cellular antigens, such as those on red blood cells, bacteria, and fungi. They are usually done in plastic microtiter plates that contain many shallow wells. The amount of particulate antigen in each well is the same, but the amount of serum that contains antibodies is diluted, so that each successive well has half the antibodies of the previous well. These tests are used, for example, to test for brucellosis and to separate Salmonella isolates into serov ovars, types defined by serological means. For infectious diseases in general, the higher the serum antibody titer, the greater the immunity to the disease. However, the titer alone is of limited use in diagnosing an existing illness. There is no way to know whether the measured antibodies were generated in response to the immediate infection or to an earlier illness. For diagnostic purposes, a rise in titer is significant; that is, the titer is higher later in the course of the disease than at its outset. Also, if it can be demonstrated that the person’s blood had no antibody titer before the illness but has a significant titer while the disease is progressing, this change, called seroconversion, is also diagnostic. This situation is frequently encountered with HIV infections. Some diagnostic tests specifically identify IgM antibodies. Short-lived IgM is more likely to reflect a response to a current disease condition (see Chapter 17). Specific immunodeficiency diseases, such as the inability to make IgG antibodies, can be identified by notable titer differences from a healthy patient exposed to the same antigen. Indirect (Passive) Agglutination Tests Antibodies against soluble antigens can be detected by agglutination tests if the antigens are attached d onto particles such as bentonite clay or, most often, extremely small latex beads. Such tests, known as latex agglutination tests, are commonly used for the rapid detection of serum antibodies against bacterial and viral diseases. In such indirect (passive) agglutination tests, the antibody reacts with the attached antigen or in reverse by using particles coated with antibodies to detect the antigens against which they are specific (Figure 18.7). The particles then agglutinate with one another, much as particles do in the direct agglutination tests. This approach is especially common in tests for the streptococci that cause sore throats. A diagnosis can be completed in about 10 minutes. Hemagglutination When agglutination reactions involve the clumping of red blood cells, the reaction is called hemagglutination. These reactions involve red blood cell surface antigens and their complementary antibodies. They are used routinely in blood typing (see Table 19.2, page 557) and in the diagnosis of infectious mononucleosis. CHECK YOUR UNDERSTANDING ✓ 18-11 Why wouldn’t a direct agglutination test work very well with viruses? ✓ 18-12 Which test detects soluble antigens: agglutination or precipitation? ✓ 18- 13 What type of test requires red blood cells to clump visibly? Neutralization Reactions Certain viruses, such as those causing mumps, measles, and influenza, can agglutinate red blood cells without an antigen– antibody reaction—a process called viral hemagglutination (Figure 18.8). Neutralization is an antigen–antibody reaction in which antibodies block the harmful effects of a bacterial exotoxin or a virus. These reactions were first described in 1890, when investigators observed that immune serum could neutralize the toxic substances produced by the diphtheria pathogen, Corynebacterium diphtheriae. Such a neutralizing substance, which is called an antitoxin, is a specific antibody produced by a host as it responds to a bacterial exotoxin or its corresponding toxoid (inactivated toxin). The antitoxin combines with the exotoxin to neutralize it (Figure 18.9a). This reaction has led to their use as diagnostic tests. Viruses that exhibit their cytopathic effects in cell culture or embryonated eggs can be used to detect the presence of neutralizing viral antibodies (see pages 462–463). If the serum to be tested contains antibodies against the particular virus, the antibodies will prevent that virus from infecting cells in the cell culture or eggs, and no cytopathic effects will be seen. Such tests, known as in vitro neutralization tests, can be used both to identify a virus and to ascertain the viral antibody titer. A neutralization test used mostly for the serological typing of viruses is the viral hemagglutination inhibition test. Certain viruses, such as those causing influenza, mumps, and measles, have surface proteins that will cause the agglutination of red blood cells. This test is most commonly used for subtyping of influenza viruses, although more laboratories are likely to be familiar with ELISA tests for this purpose. If a person’s serum contains antibodies against these viruses, these antibodies will react with the viruses and neutralize them (compare Figure 18.8 and Figure 18.9b). For example, if hemagglutination occurs in a mixture of measles virus and red blood cells but does not occur when the patient’s serum is added to the mixture, this result indicates that the serum contains antibodies that have bound to and neutralized the measles virus. CHECK YOUR UNDERSTANDING ✓ 18-14 What is the connection between hemagglutination and certain viruses? ✓ 18-15 Which test is an antigen–antibody reaction: precipitation or viral hemagglutination inhibition? Complement-Fixation Reactions In Chapter 16 (pages 489– 493), we discussed a group of serum proteins collectively called complement. During most antigen–antibody reactions, a complement serum protein binds to the antigen–antibody complex and is used up, or fixed. This process of complement fixation can be used to detect very small amounts of antibody. Complement fixation was once used to diagnose syphilis (Wassermann test) and is still used to diagnose certain viral, fungal, and rickettsial diseases. The complement-fixation test requires great care and good controls. This is one reason why newer, simpler tests such as ELISA and PCR-based tests are increasingly replacing it. The test is performed in two stages: complement fixation and indicator. Fluorescent-antibody (FA) techniques can identify microorganisms in clinical specimens and can detect the presence of a specific antibody in serum (Figure 18.11). These techniques combine fluorescent dyes such as fluorescein isothiocyanate (FITC) with antibodies that fluoresce when exposed to ultraviolet light (see Figure 3.6, page 83). These procedures are quick, sensitive, and very specific; the FA test for rabies can be performed in a few hours and has an accuracy rate close to 100%. Fluorescent-antibody tests are of two types. Direct fluorescentantibody (FA) tests are usually used to identify a microorganism in a clinical specimen (Figure 18.11a). During this procedure, the specimen containing the antigen to be identified is fixed onto a slide. Fluorescein-labeled antibodies are then added, incubated briefly, and washed to remove any antibody not bound to antigen. Yellow-green fluorescence under the fluorescence microscope from the bound antibody will be visible even if the antigen, such as a virus, is submicroscopic in size. Indirect fluorescent-antibody (FA) tests are used to detect the presence of a specific antibody in serum following exposure to a microorganism (Figure 18.11b). They are often more sensitive than direct tests. A known antigen is fixed onto a slide, and then the test serum added. If antibody that is specific to that microbe is present, it reacts with the antigen to form a bound complex. To make the antigen–antibody complex visible, fluorescein-labeled anti-human immune serum globulin (anti-HISG), an antibody that reacts specifically with any human antibody, is added to the slide. Anti-HISG will be present only if the specific antibody has reacted with its antigen and is therefore present as well. After the slide has been incubated and washed (to remove unbound antibody), it is examined under a fluorescence microscope. If the known antigen fixed to the slide appears fluorescent, the antibody specific to the test antigen is present. One adaptation of fluorescent antibodies is the fluorescenceactivated cell sorter (FACS). In Chapter 17, we learned that T cells carry antigenically specific molecules such as CD4 and CD8 on their surface, and these are characteristic of certain groups of T cells. The depletion of CD4+ T cells is used to follow the progression of AIDS; their populations can be determined with a FACS. The FACS is a modification of a flow cytometer, in which a suspension of cells leaves a nozzle as droplets containing no more than one cell per drop. A laser beam strikes each cell-containing droplet and is then received by a detector that determines certain characteristics, such as size (Figure 18.12). For example, if the cells have FA-bound antibody markers to identify them as CD4+ or CD8+ T cells, the detector can measure this fluorescence and cell size. As the laser beam detects a cell of a preselected size or fluorescence, an electrical charge, either positive or negative, can be imparted to it. As the charged droplet falls between electrically charged plates, it is attracted to one receiving tube or another, effectively separating cells of different types. Millions of cells can be separated in an hour with this process, all under sterile conditions, which allows them to be used in experimental work. An interesting application of the flow cytometer is sorting sperm cells to separate male (Y-carrying) and female (X-carrying) sperm. The female sperm (meaning that it will result in a female embryo when it fertilizes the egg) contains more DNA, 2.8% more in humans, 4% in animals. When the sperm is stained with a fluorescent dye specific for DNA, the female sperm glows more brightly when illuminated by the laser beam and can be separated out. The technique was developed for agricultural purposes. However, it has received medical approval for use in human couples who carry genes for inherited diseases that affect only boys. The enzyme-linked immunosorbent assay (ELISA) is the most widely used of a group of tests known as enzyme immunoassay (EIA). There are two basic methods. The direct ELISA detects antigens, and the indirect ELISA detects antibodies. A microtiter plate with numerous shallow wells is used in both procedures (see Figure 10.12a, page 309). ELISA procedures are popular primarily because they are sensitive and require little interpretive skill to read. Because the procedure and results are highly automated, the results tend to be clearly positive or clearly negative. Many ELISA tests are available for clinical use in the form of commercially prepared kits. Some tests based on this principle are also available for use by the public, including a commonly available home pregnancy test (Figure 18.13). Direct ELISA The direct ELISA method is shown in Figure 18.14a. A common use of the direct ELISA test is to detect the presence of drugs in urine. For these tests, antibodies specific for the drug are attached to a well of the microtiter plate. (The availability of monoclonal antibodies has been essential to the widespread use of the ELISA test.) When the patient’s urine sample is added to the well, any of the drug that it contained would bind to the antibody and is captured. The well is rinsed to remove any unbound drug. To make a visible test, more antibodies specific to the drug are now added (these antibodies have an enzyme attached to them—therefore, the term enzyme-linked) and will react with the already-captured drug, forming a “sandwich” of antibody/drug/enzyme-linked antibody. This positive test can be detected by adding a substrate for the linked enzyme; a visible color is produced by the enzyme reacting with its substrate. Indirect ELISA The indirect ELISA test, illustrated in Figure 18.14b, detects antibodies in a patient’s sample rather than an antigen such as a drug. Indirect ELISA tests are used, for example, to screen blood for antibodies to HIV (see page 576). For such a purpose, the microtiter wells contain antigens, such as the inactivated virus that causes the disease the test is designed to diagnose. A sample of the patient’s serum is added to the well; if it contains antibodies against the virus, they will bind the antigen. The well is rinsed to remove unbound antibodies. If antibodies in the serum and the virus in the well have attached to each other, they will remain in the well—a positive test. To make a positive test visible, some anti-HISG (an immunoglobulin that will attach to any antibody, including the one in the patient’s serum that has attached to the virus in the well; see page 540) is added. The anti-HISG is linked to an enzyme. A positive test consists of a “sandwich” of a virus/antibody/enzyme- linked-anti-HISG. At this point, the substrate for the enzyme is added, and a positive test is detected by the color change caused by the enzyme linked to the anti-HISG. Western Blotting (Immunoblotting) Western blotting, often simply called immunoblotting, can identify a specific protein in a mixture (such as proteins extracted from a blood sample). The components of the mixture are separated by electrophoresis in a gel and then transferred to a protein-binding sheet (blot). There the blot is flooded with an enzyme- linked antibody specific for the antigen. The location of the antigen and the enzyme-linked antibody reactant can be visualized, usually with a color-reacting label similar to an ELISA test reaction (see Figure 18.14). Figure 10.13, page 310, shows the steps of the Western blot for diagnosing Lyme disease. The most frequent application is in a confirmatory test for HIV infection (see page 576). The Future of Diagnostic and Therapeutic Immunology The introduction of monoclonal antibodies has revolutionized diagnostic immunology by making available large, economical amounts of specific antibodies. This has led to many newer diagnostic tests that are more sensitive, specific, rapid, and simpler to use. For example, tests to diagnose sexually transmitted chlamydial infections and certain protozoan-caused intestinal parasitic diseases are coming into common use. These tests had previously required relatively difficult culture or microscopic methods for diagnosis. At the same time, the use of many of the classic serological tests, such as complement- fixation tests, is declining. The use of certain nonimmunological tests, such as nucleic acid amplification tests (NAATs) that were discussed in Chapter 10 (page 312), is increasing. NAATs are becoming automated to a significant degree. For example, a DNA chip (see Figure 10.18, page 314) containing over 50,000 single-stranded DNA probes can identify genetic information, including that of possible pathogens in a test sample. This chip is scanned and its data automatically analyzed. Most of the diagnostic tests described in this chapter are those used in the developed world. In many less-developed countries, the money available for diagnosis and treatment alike is tragically small. The diseases that most of these diagnostic methods target are also those that are more likely to be found in developed countries. In many parts of the world, especially tropical Africa and tropical Asia, there is an urgent need for diagnostic tests for diseases endemic in those areas, such as malaria, leishmaniasis, AIDS, Chagas’ disease, and tuberculosis. These tests will need to be inexpensive and simple enough to be carried out by personnel with minimal training. The tests described in this chapter are most often used to detect existing disease. In the future, diagnostic testing could be directed at preventing disease. In the United States we regularly see reports of outbreaks of foodborne disease. Fresh produce does not carry the identifying marks that facilitate the tracking of packaged food products. Thus, sampling methods that would allow complete identification (including specific pathogenic serovars) within a few hours, or even minutes, would save valuable time in tracking outbreaks of infectious disease carried by fruits and vegetables. This saving in time would be translated into immense economic savings for growers and retailers. It would also lead to less human illness. Not every topic discussed in this chapter is necessarily directed at detecting and preventing disease. As was mentioned on pages 534–535, Mabs have applications in the therapy of disease as well. These are already in use to treat certain cancers such as breast cancer and non-Hodgkin’s lymphoma, as well as inflammatory diseases such as rheumatoid arthritis.