Micro Test 2 PDF
Document Details
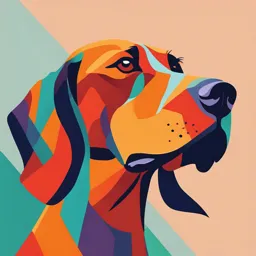
Uploaded by RenewedProtactinium
Tags
Summary
This document provides information on microbial growth and growth control, covering environmental effects such as temperature and pH. It also discusses different classes of microorganisms based on their optimal growth temperatures, such as psychrophiles, psychrotolerants, and mesophiles.
Full Transcript
Microbial Growth and Growth Control part 2 Class 10 - test 2 material starts here Environmental Effects on Growth - Physical and Chemical factors that control/affect microbial growth: - Temperature: a major environmental factor controlling microbial growth - It aff...
Microbial Growth and Growth Control part 2 Class 10 - test 2 material starts here Environmental Effects on Growth - Physical and Chemical factors that control/affect microbial growth: - Temperature: a major environmental factor controlling microbial growth - It affects in two opposing ways. – promote or inhibit growth - Temperature Classes of Microorganisms –Microorganisms can be classified into groups by their growth temperature optima - Psychrophile: low temperature – cold; found in permanently cold environments – Optima at 15 ºC - Psychrotolerant: Organisms that can grow at 0ºC but have optima of 20ºC to 40ºC - More widely distributed in nature than psychrophiles - Molecular adaptations that support psychrophily - Production of enzymes that function optimally in the cold; features that provide more flexibility (in cold membrane becomes more rigid) - More α-helices (more flexible) than β-sheets (more rigid) - More polar, less hydrophobic amino acid - Fewer weak bonds (ionic and hydrogen) - Decreased interactions between protein domains - Transport processes function optimally at low temperatures - Modified cytoplasmic membranes; High unsaturated fatty acid content (introduce kinks due to bulky double bonds) - Mesophile: midrange temperature; found in: - Warm-blooded animals, Terrestrial and aquatic environments, Temperate and tropical latitudes - Thermophile: high temperature - Prokaryotes are able to grow at higher temperatures than eukaryotes - Organisms w/ highest temperature optima are Archaea - Non Phototrophic organisms can grow at higher temperatures than phototrophic organisms - Molecular adaptations to thermophily; Enzyme and proteins function optimally at high temperatures; features that provide thermal stability: - Critical amino acid substitutions in a few locations provide more heat-tolerant folds - An increased # of (strong) ionic bonds between basic and acidic amino acids resists unfolding in the aqueous cytoplasm (more rigid) - Production of solutes (e.g. di-inositol phosphate, diglycerol phosphate) help stabilise proteins - Modifications in cytoplasmic membranes to ensure heat stability - Bacteria have lipids rich in saturated fatty acids + cholesterol (fills spaces– more rigid) - Archaea have lipid monolayer rather than bilayer - Hyperthermophile: very high temperature - Extremophiles: Organisms that grow under very hot or very cold conditions - pH - Some organisms have evolved to grow best at low or high pH, but most organisms grow best between pH 6 and 8 (neutrophiles) - Acidophiles: organisms that grow best at low pH (9) - Some have sodium motive force rather than proton m.f. (because the protons would be neutralised by the base) - The internal pH of a cell must stay relatively close to neutral even though the external pH is highly acidic or basic - However, Internal pH has been found to be as low as 4.6 and high as 9.5 in extreme acidophiles & alkaliphiles, respectively - Microbial culture media typically has buffers to maintain constant pH - Water content – osmolarity (water moves to where solute is highest) - Typically, the cytoplasm has a higher solute concentration than the surrounding environment; thus, the tendency is for water to move into the cell (osmosis process, positive water balance) - Hypotonic: salt lower outside (water gets added, burst) - When a cell is in an environment with a higher external solute concentration, water will flow out unless the cell has a mechanism to prevent this - Hypertonic: salt higher outside (water gets lost–shrivel) - Halophiles: organisms that grow best at reduced water potential; have a specific requirement for NaCl - Extreme halophiles: require high levels (15–30%) of NaCl for growth - Halotolerant: can tolerate some reduction in water activity of environment but generally grow best in absence of the added solute - Osmophiles: live in environments high in sugar as solute - Xerophiles: organisms able to grow in very dry environments - Mechanisms for combating low water activity in surrounding environment involve increasing the internal solute concentration by - Pumping inorganic ions from environment into cell - Synthesising or concentrating organic solutes - Compatible solutes: compounds used by cell to counteract low water in surrounding environment - Oxygen - 1. Aerobes: require oxygen to live - Facultative organisms: aerobes that can live w/ or w/out o2 - Microaerophiles: can use oxygen only when it is present at levels reduced from that in air - 2. Anaerobes: don’t require oxygen, may even be killed by exposure - Aerotolerant anaerobes: can tolerate oxygen and grow in its presence even though they cannot use it - Thioglycolate broth: Complex medium that separates microbes based on oxygen requirements - Reacts w/ oxygen, so it can penetrate only the top of the tube - Special techniques are needed to grow aerobic and anaerobics - Aerobic, require constant shaking of the culture - Anaerobes: Reducing agents are used–chemicals that may be added to culture media to reduce oxygen (e.g., thioglycolate) - Several toxic forms of oxygen can be formed in the cell (molecular oxygen is not toxic). Some have mechanisms to deal with this while others do not: - Single oxygen - Superoxide anion – takes an e- (can transform proteins etc) - Hydrogen peroxide (highly reactive) - Hydroxyl radical(highly reactive) - Enzymes are present to neutralise most of these toxic oxygen species - Catalase – bubbles in hydrogen peroxide - Peroxidase - Superoxide dismutase - Superoxide reductase Controlling Microbial Growth General Principles and Growth Control by Heat – physical method - Sterilisation: The killing or removal of all viable organisms within a growth medium - Inhibition: Effectively limiting microbial growth - Decontamination: The treatment of an object to make it safe to handle - Disinfection: Directly targets the removal of all pathogens, not necessarily all microorganisms - Heat sterilisation is the most widely used method of controlling microbial growth - High temperatures denature macromolecules - Amount of time required to reduce viability tenfold is called the decimal reduction time - Only guaranteed reduction of viable cells, not endospores - Some bacteria produce resistant cells called endospores: Can survive heat that would rapidly kill vegetative cells - The autoclave is a sealed device that uses steam under pressure: Allows temperature of water to get above 100ºC (usually 121) - It's not the pressure, but the high temperature, that kills the microbes - Pasteurization is the process of using precisely controlled heat to reduce the microbial load in heat-sensitive liquids - Does not kill all organisms, so it is different from sterilisation Other Physical Control Methods: Radiation and Filtration - Microwaves, UV, X-rays, gamma rays, and electrons can reduce microbial growth - UV (220-300 nm) has sufficient energy to cause modifications+breaks in DNA - UV is useful for decontaminating surfaces - Surface needs to be already clean for it to penetrate - Cannot penetrate solid, opaque, or light-absorbing surfaces - Ionising radiation - Electromagnetic radiation that produces ions and other reactive molecules - Generates electrons, hydroxyl radicals, and hydride radicals - Some microorganisms (eg endospores) are more resistant to radiation than others - Sources of radiation include cathode ray tubes, X-rays, and radioactive nuclides - Radiation is used for sterilisation in the medical field and food industry - Radiation is approved by WHO and is used in the USA for decontaminating foods particularly susceptible to microbial contamination (burgers, chicken, spices etc) - Filtration avoids the use of heat on sensitive liquids and gases - Pores of filter are too small for organisms to pass through - Pores allow liquid or gas to pass through - Depth filters: HEPA filters - Membrane filters: Function more like a sieve - Filtration can be accomplished by syringe, pump, or vacuum - A type of membrane filter is the nucleation track (nucleopore) filter Chemical Control of Microbial Growth - Antimicrobial agents can be classified as: - Bacteriostatic - prevents growth but once removed they grow again - bacteriocidal - (intact but can not grow) - bacteriolytic - (dead) - Minimum inhibitory concentration (MIC) is the smallest amount of an agent needed to inhibit growth of a microorganism - Varies with the organism used, inoculum size, temperature, pH, etc. - Disc diffusion assay uses solid media - Antimicrobial agent added to filter paper disc - MIC is reached at some distance - Zone of inhibition: Area of no growth around disc - Products designed to prevent growth of human pathogens in inanimate environments and on external body surfaces - Sterilants, disinfectants, sanitizers, and antiseptics - Sterilants destroy all microorganisms, including endospores. - Disinfectant: used on surfaces to kill bacteria but not necessarily endospores. - Sanitizers reduce microbial numbers but do not sterilise. - Antiseptics (germicides) kill or inhibit microbial growth but are nontoxic enough to be applied to living tissues. Class 11 Microbial Regulatory Systems Overview of Regulation - A myriad of reactions occur at particular points of growth in cells. To maximise their resources, cells must regulate the types, amounts, and activities of proteins and other macromolecules. - Biological information flows from DNA to RNA to Protein. The process of gene transcription into mRNA followed by translation of mRNA into protein is referred to as gene expression. - Regulation occurs at all molecular levels in the cells: DNA, RNA and proteins. - Microbial genomes encode many proteins that are not needed all the time. - Regulation helps conserve energy and resources. - Proteins and RNA molecules that are needed in the cell under all growth conditions are said to be constitutive. - Gene expression and regulation of protein activity: Gene expression: process of gene transcription into RNA followed by translation of mRNA into proteins Proteins and RNA that are needed for all cell growth conditions are constitutive There are checkpoints in expression – can only proceed to next step if previous is done Major Modes of Regulation - Two major levels of regulation in the cell: - One controls the amount of an enzyme - Regulates level of transcription - Regulates translation - Slower process (minutes) - One controls the activity of preexisting enzymes - Post-translational regulation - Very rapid process (seconds) DNA-Binding Proteins and Transcriptional Regulation: down and upstream DNA binding proteins (DBP) - Protein amount can be regulated at the transcription level, or by protein degradation. - Regulation of transcription requires proteins that can bind to DNA (DBP) - Small molecules (metabolites) influence the binding of regulatory proteins to DNA - The domain of a DBP contains protein–protein contacts, holding protein dimer together and a DNA-binding domain that fits in major grooves (each major groove it binds has the same (inverted) sequence) and along sugar–phosphate backbone - Major groove of DNA is the main site of protein binding - They are homodimeric: proteins composed of two identical polypeptides - Protein dimers interact with inverted repeats on DNA - Each of the polypeptides binds to one inverted repeat - Most DNA-binding proteins interact with DNA in a sequence-specific manner - Specificity is provided by interactions between amino acid side chains and chemical groups on the bases and sugar–phosphate backbone of DNA - Inverted repeats frequently are the binding sites for regulatory proteins - Several classes of protein domains are critical for proper binding of proteins to DNA - Helix-turn-helix – very common, found in bacteria and eukaryotes - First helix is the recognition helix which interacts with 1 major groove - Second helix is the stabilising helix which interacts with the other - Many different DNA-binding proteins from Bacteria contain helix-turn-helix: eg. lac and trp repressors of E. coli - Zinc finger - Protein structure that binds a zinc ion - Eukaryotic regulatory proteins use zinc fingers for DNA binding - Globular, 2 cys in a beta sheet and 2 his in an alpha helix tetrahedrally holding a zn ion - Leucine zipper - Contains regularly spaced leucine residues – creates hydrophobic patch which creates a very stable homodimer - Function is to hold two recognition helices in the correct orientation Two outcomes, after DNA binding: 1. The binding event can block transcription (negative regulation) 2. The binding event can activate transcription (positive regulation) 1. Negative control of transcription: block transcription - There are two major mechanisms that can BLOCK enzyme (protein) production based on the cells needs: - Enzymes are not synthesized if the product is present. This is called enzyme repression. - prevents the synthesis of an enzyme in response to a signal - Enzymes affected by repression make up a small fraction of proteins - If we have enough product we do not need to make more - Typically affects anabolic enzymes (e.g., arginine biosynthesis) - Enzymes are not synthesized if the substrate is absent. This is called enzyme induction. - production of an enzyme in response to a signal - Typically affects catabolic enzymes (e.g., lac operon) - Enzymes are synthesized only when they are needed, if substrate is not there, why would we synthesise the enzymes? No wasted energy. - Eg of repression in the arginine operon Eg of induction in the lactose operon 2. Positive control of transcription: activate transcription - Positive control: regulator protein activates the binding of RNA polymerase to DNA - Eg. Maltose catabolism in E. coli - Maltose activator protein can’t bind to DNA unless it 1st binds maltose -- acts as a anchor for polymerase - Activator proteins bind specifically to certain DNA sequence - Called activator-binding site, not operator - Promoters of positively controlled operons only weakly bind RNA polymerase - Activator protein helps RNA polymerase recognize promoter - May cause a change in DNA structure - May interact directly with RNA polymerase - Activator-binding site may be close to the promoter or several 100 base pairs away - Many E. coli genes have promoters under positive or negative control. Many operons have promoters w/ multiple types of control, some have more than one promoter. - Sometimes, the genes that encode proteins involved in a biosynthetic pathway, e.g. maltose genes, are spread out over the chromosome in several operons, unlike the genes that are involved in lactose metabolism, which are grouped in a single operon. - Each operon has an activator-binding site - Multiple operons controlled by same regulatory protein are called a regulon - Regulons also exist for negatively controlled systems Global control of transcription: - Global control systems: regulate expression of many different, and unrelated, genes simultaneously - Catabolite repression is an example of global control - Ensures that the "best" carbon and energy source is used first - Synthesis of unrelated catabolic enzymes is repressed if glucose is present in growth medium (glucose used first– it's the best) - lac operon is under control of catabolite repression -- plateau is expression of lactose enzymes+shutdown of glucose ones - Dozens of catabolic operons are affected by catabolite repression - Enzymes for degrading lactose, maltose, and other common carbon sources - Flagellar genes are also controlled by catabolite repression - No need to swim in search of nutrients when there is abundance Sensing and Signal Transduction Two-Component Regulatory Systems - Prokaryotes regulate cellular metabolism in response to environmental fluctuations - External signal is detected by a sensor and transmitted to regulatory machinery (signal transduction) - Most signal transductions are two-component regulatory systems - Two-component regulatory systems - Made up of two different proteins: - Sensor his kinase (membrane): detects environment signal - Kinase: adds phosphate groups - Spans inside to outside of cell across membrane - Response regulator (cytoplasm): DNA-binding protein that regulates transcription (either neg or pos regulation) based on the sensor - Also has feedback loop which terminates the response - Almost 50 different two-component systems in E. coli - Examples include phosphate assimilation, nitrogen metabolism, osmotic pressure response, and antibiotic resistance - Some signal transduction systems have multiple regulatory elements - Some Archaea also have two-component regulatory systems - HIS kinase binds a phosphate to itself from ATP, as a response to signals, which gets transferred to a DBP, and now it either binds more or less to a particular gene Class 12 Quorum Sensing: counting themselves. Often utilises two component systems - Prokaryotes can respond to the presence of other cells of the same species - Quorum sensing: mechanism by which bacteria assess their population density - Ensures that a sufficient number of cells are present before initiating a response that, to be effective, requires a certain cell density (e.g., toxin production in pathogenic bacterium) - Virulence factors: pathogens use quorum sensing to make sure they have reached a critical mass, before they start damaging the host, so they are strong enough to overwhelm the host's defences - Each species of bacterium produces a specific autoinducer molecule - Made in a cell, and diffuses freely across the cell envelope to other cells to tell them ‘im here!’ Reaches high concentration inside cell only if many cells near - Binds to specific activator protein and triggers transcription of specific genes - Several different classes of autoinducers - Gram neg: Acyl homoserine lactone (AHL): first autoinducer to be identified - Quorum sensing was first discovered as a mechanism regulating light production in bacteria including Aliivibrio fischeri - Examples of quorum sensing - 1. Virulence factors - Eg. Escherichia coli O57:H7 (gram neg) - Shiga toxin–producing strain - Produces AHL AI-3 - Epinephrine plus norepinephrine plus AI-3 bind to sensor molecules in plasma membrane - Activates motility, enterotoxin production, and production of virulence proteins - - Eg. Staphylococcus aureus (gram pos) - Secretes small peptides that damage host cells or alter host's immune system - Under control of autoinducing peptide (AIP) - Activates several proteins that lead to production of virulence proteins - - 2. Switching from free-living to growing as a biofilm - Eg. Pseudomonas aeruginosa - Produces polysaccharides that increase pathogenicity and antibiotic resistance - Two quorum-sensing systems - Produces AHLs and cyclic di-guanosine monophosphate (c-di-GMP) - Leads to exopolysaccharide production and flagella synthesis - - Quorum sensing is present in some microbial eukaryotes - Quorum sensing likely exists in Archaea Translational regulation: RNA-based regulation Regulatory RNAs: small and antisense (non coding) - Regulatory RNA molecules exert their effects by base pairing with complementary mRNA. There are four distinct mechanisms of altering translation process: - Block a ribosome-binding site (RBS): may be complementary to RBS, blocks. - Open up a blocked RBS - Increase degradation of mRNA - Decrease degradation of mRNA Riboswitches: - Riboswitches: RNA domains in an mRNA molecule that can bind small molecules to control translation of mRNA - Located at 5′ end of mRNA - Binding results in folding of RNA into a 3-D structure - Similar to a protein recognizing a substrate - Riboswitch control is analogous to negative control - Found in some bacteria, fungi, and plants analogous to arginine operon regulation Attenuation: - Transcriptional control that functions by premature termination of mRNA synthesis - Control exerted after the initiation of transcription, but before its completion - The first transcribed RNA sequence forms two alternative secondary structures, which control the fate of transcription completion. - First example was the tryptophan operon in E. coli - mRNA stem–loop structure and synthesis of leader peptide are determining factors in attenuation - Genomic evidence suggests attenuation exists in Archaea a) Hairpin Loop causes RNA pol to be unable to bind b) RNA poly can bind Post-translational regulation: Regulation of enzymes and other proteins. - Faster then regulation at the DNA and mRNA level Feedback inhibition - Feedback inhibition: mechanism for turning off reactions in a biosynthetic pathway - End product (allosteric effector) of the pathway binds to the first enzyme in the pathway, thus inhibiting its activity - Inhibited enzyme is an allosteric enzyme w/ 2 binding sites: active, allosteric - Reversible reaction - Some pathways controlled by feedback inhibition use isoenzymes: Different enzymes that catalyse the same reaction but are subject to different regulatory controls - final products do not bind covalent (not permanent) Post translational modification: - Biosynthetic enzymes can also be regulated by covalent modifications (not permanent) - Regulation involves a small molecule attached to or removed from the protein - No issues with this at all - Results in conformational change that inhibits activity - Common modifiers include adenosine monophosphate (AMP), adenosine diphosphate (ADP), inorganic phosphate (PO42-), and methyl groups (CH3) - Eg. Regulation of glutamine synthetase by covalent modification Class 13 Microbial Genetics Mutation Mutants: - DNA damage can cause mutations, but are only considered mutations if heritable - Mutation: Heritable change in DNA sequence that may lead to a change in phenotype (observable properties of an organism) - Mutant: A strain of any cell or virus differing from parental strain in genotype (nucleotide sequence of genome) - Wild-type strain: Typically refers to a strain isolated from nature - The observable properties of the mutant, referred to as phenotype, may be altered relative to the parent strain. - A genotype is designated by: abcK and a number - A phenotype is designated by Abc+/- (+=mutant, - =wild type) - Selectable mutations - Those that give the mutant a growth advantage under certain conditions, e.g. antibiotic resistance. Are useful in genetic research - Non Selectable mutations - Those that usually have neither an advantage nor a disadvantage (usually to do with growth on selective media) over the parent, e.g. loss of pigmentation - Detecting such mutations requires examining a large number of colonies and looking for differences (screening; auxotrophs) Molecular basis of mutation: - Point mutations: Mutations that change only one base pair - Can lead to single amino acid change in a protein, an incomplete protein, or no change at all - Transitions are purines (A/G) substituted for other purines; Transversions are purines substituted for pyrimidines (C/T) or vice versa. - Possible effects of base-pair substitution in a gene encoding a protein: - Missense (new AA), Nonsense (premature stop AA), Silent (Same AA) - Deletions and insertions cause more dramatic changes in DNA. They are referred to as Frameshift mutations: they result in a shift in the reading frame. - Often result in complete loss of gene function only after the deletion/insertion = new AA sequence Reversions and mutation rates: - Point mutations are typically reversible - Reversion - Alteration in DNA that reverses (restores the activity) the effects of a prior mutation - Revertant: Strain in which original phenotype is restored - Two types - Same-site revertant: mutation is at the same site as original mutation. If the point site mutation is reverted then strain is a true revertant. - Second-site revertant: mutation is at a different site in the DNA. - Suppressor: mutation that compensates for the effect of the original mutation - Suppression of nonsense mutations - Several classes of suppressors in second site reversion are known: (a) a mutation in the same gene, but different site (b) a mutation in another gene that restores the activity of the mutated gene, (c ) a mutation in another gene that assumes the function of the mutated gene * suppressor allowed tRNA to still bring gln, despite improper codon sequence - For most microorganisms, errors in DNA replication occur at a frequency of 10^-6 to 10^-7 per kilobase - DNA viruses have error rates 100–1000x greater (they rely on mutations) - The mutation rate in RNA genomes is 1000-fold higher than in DNA genomes - Point mutations are more likely to lead to missense mutations, and silent mutations (second most common outcome) than nonsense mutations. Mutagenesis: - Spontaneous mutations: Those that occur without external intervention - Induced mutations: Those made environmentally or deliberately (physical of chemical factors), e.g. can result from exposure to natural radiation or oxygen radicals - Mutagens: Chemical, or physical, biological factors that increase mutations - 1. Several classes of chemical mutagens exist: - Nucleotide base analogs: resemble nucleotides - Chemical mutagens that induce chemical modifications - EX: alkylating agents such as nitrosoguanidine - Chemical mutagens that cause frameshift mutations - For example, intercalating agents such as acridines - *make dna poly make mistakes - 2. Several forms of radiation - Two main categories of mutagenic electromagnetic radiation - Non Ionizing (i.e., UV radiation) - Purines and pyrimidines strongly absorb UV - Pyrimidine dimer is one effect of UV radiation - Ionising (i.e., X-rays, cosmic rays, and gamma rays) - Ionize water and produce free radicals - Free radicals damage macromolecules in cell - - Three types of DNA repair systems - Direct reversal: mutated base is still recognizable and can be repaired without referring to other strand (repairs to what it was originally) - Repair of single-strand damage: damaged DNA is removed and repaired using opposite strand as template (repairs to what it was originally) - Repair of double-strand damage: a break in the DNA - Requires more error-prone repair mechanisms. Just attaches the 2 strands together, which can still=mutation (cell only wants to replicate) - When DNA damage is of a large scale, the cell may use a different type of repair system (i.e., damage interferes with DNA replication) - Mechanism called the SOS regulatory system (SOS regulon) - This system is more error-prone - Allows replication to proceed+cell to replicate, but errors more likely - Translesion synthesis allows DNA to be synthesized with no template - LexA is a repressor of SOS regulon, its repression= released by RecA Gene transfer in bacteria Genetic recombination - Recombination: Physical exchange of DNA between genetic elements - Homologous recombination: Process that results in genetic exchange between homologous DNA sequences from two different sources - homologous recombination: SSBs prevent religation of the DNA Helicase separates the strands This can be done with extracellular DNA as well - new DNA needs to share a sequence with the host DNA, so it can base pair together Can result in patches or splices - To generate new genotypes, the homologous sequences must be related but distinct (e.g., diploid eukaryotic cell). - In bacteria, genetic recombination occurs after transformation, transduction, or conjugation. - To detect the exchange of DNA, recombinant cells must phenotypically differ from both parents. - usually use recipient strains lacking a selectable characteristic that recombinants gain Transformation: Acquisition of extracellular DNA - Transformation: Genetic transfer process by which DNA is incorporated into a recipient cell and brings about genetic change - Not all prokaryotes are naturally transferable - We can use heat shock (chemical) or electroporation to make cells artificially competent - The typical DNA transferable size is ~10 kbp (about 10 genes). - A single cell may incorporate one or a few DNA fragments in a single transformation event. - Competent: cells are capable of taking up DNA and being transformed - In naturally transformable bacteria, competence is regulated - In other strains, specific procedures are necessary to make cells competent (chemical transformation) - Electricity can be used to force cells to take up DNA (electroporation) - They digest the double strand into single strand - The single strand must share a sequence with the host DNA - This sequence will get incorporated via recombination - If it does not share a sequence with the host DNA, the cell can digest it and use the strand as a source of carbon Transduction: Acquisition of extracellular DNA - Transduction: Transfer of DNA from one cell to another by a bacteriophage - Two modes - Generalized transduction: DNA from any portion of the host genome is packaged inside the virion (random) - - Specialized transduction: DNA from a specific region of the host chromosome is integrated directly into the virus genome (specific) Does not fully digest the host chromosome, unlike generalised transduction Conjugation: Acquisition of extracellular DNA - Bacterial conjugation (mating): mechanism of genetic transfer that involves cell-to-cell contact (eg with pilli) - Highly regulated process; or else it would gain so much new genes it wouldn't be the same bacteria - Plasmid-encoded mechanism - Donor: has conjugative plasmid - Recipient: doesn’t contain plasmid - Conjugative plasmid: F (fertility) plasmid - Circular DNA molecule; ~100 kbp - Has genes that regulate DNA replication - Has several TEs that allow the plasmid to integrate into the host chromosome - Contains tra genes>code transfer function - - The Formation of Hfr Strains and Chromosome Mobilisation - F plasmid is an episome; which means it can integrate into the host's chromosome - Cells possessing a nonintegrated F plasmid are called F+ - Cells possessing an integrated F plasmid are called Hfr (high frequency of recombination) - High rates of recombination between genes on the donor chromosome and those of the recipient - Happens when Insertion sequences (mobile elements) are present in both the F plasmid + chromosome - Facilitate homologous recombination - Plasmid is now part of chromosome - Chromosomal genes transferred with plasmid - Type IV pilli: - Also used to inject things into other cells–DNA transfer (conjugation) - extends through the outer membrane into another cell during conjugation. It makes contact with a receptor on the host where the DNA is recognised by an inner membrane protein of the donor and is then transferred using ATP facilitated transport - Not only can it transfer from bacteria > bacteria, but from the bacteria to other cell types (eg plant cells) - Summary Class 14 Transposable elements (TEs) - Mobile, jumping DNA - Discrete segments of DNA that move as a unit from one location to another within or other DNA molecules are transposable elements - Transposable elements can be found in all three domains of life - Move by a process called transposition - Frequency of transposition is 1 in 1000 to 1 in 10,000,000 per generation - Usually very rare (can cause issues in small cells) - First observed by Barbara McClintock - Beneficial when cells are under extreme stress - 2 main types of TEs in Bacteria are transposons and insertion sequences - Both carry genes encoding transposase - Both have inverted repeats at their ends - Insertion sequences are the simplest transposable element - In general they do not carry extra genes (like antibiotic resistance) - ~1000 nucleotides long, and are 10–50 base pairs - Only gene is for the transposase - Found in plasmids and chromosomes of Bacteria, Archaea, and some bacteriophages - Transposons are larger than insertion sequences - Transposase moves any DNA between inverted repeats - Insertion of a TE generates a duplicate target sequence - May include antibiotic resistance - Examples are Tn5 and Tn10 - Mechanisms of transposition: two types - Conservative: transposon is excised from one location and reinserted at a second location (e.g., Tn5) - Number of transposons stays constant - Can be problematic for donor - Replicative: a new copy of transposon is produced+inserted at a 2nd location - Number of transposons present doubles Spacer sequences: - Preserving Genome Integrity: CRISPR Interference - CRISPR: Clustered Regulatory Interspaced Short Palindromic Repeats - Type of prokaryotic "immune system" - Region of bacterial chromosome containing DNA sequences similar to foreign DNA (spacers) alternating with identical repeated sequences - CRISPR-associated proteins (Cas proteins) - Obtain and store segments of foreign DNA as spacers - Recognize and destroy foreign DNA - Operation of the CRISPR system: Microbial omics How to find animal origins of virus’ like covid - Genomics, the sequence of the RNA of this virus, and then comparing it with other coronaviruses found in bats and other animals. - Found that the bat-borne virus lacks the hardware to latch onto human cell receptors. - We still do not know where covid came from – could be pangolin – covid RNA from them is 99% similar to ours – this is still not sufficient - To conclusively identify the culprit, researchers would need to test each species that was on sale at the “wet market in Wuhan” – Almost impossible!! Genomics Intro - Genome: Entire complement of genetic information - Includes genes, regulatory sequences, and noncoding DNA - Genomics: discipline of mapping, sequencing, analysing, and comparing genome - Genomics: then and now - First genomes sequenced were small viruses - first bacterial genome published in 1995 - Today, DNA sequences from 125,000+ Bacteria, Archaea, and viruses are available publicly (e.g., Genomes Online Database [GOLD]) - many eukaryotic genomes sequenced, including human genome - Utility of microbial genome sequences. - Mapping, modelling, monitoring outbreaks, diagnosing pathogens, identifying drug targets, insight into how pathogens work - What can genomes tell us? - heat-stable enzymes to virulence factors - studying gene expression, detecting horizontal transfer, monitoring disease outbreaks, discovering CRISPRs, understanding metabolism, and determining growth requirements - solving medical mysteries (e.g., cause of “Black Death”) - identifying new microbial phyla - Found Remnants of ancient viral pandemics in the form of viral DNA sequences embedded in our genomes are still active in healthy people – called HERVs (human endogenous retroviruses); make up 8% of our genome - Found that Among the traits with the strongest Neanderthal DNA contribution were smoking habits, alcohol consumption and sleeping patterns - Also found that those with certain neanderthal DNA had more serious reaction to covid Sequencing and annotating genomes - Genome assembly consists of connecting the DNA fragments in the correct order, eliminating overlaps - Occasionally assembly is not possible due to gaps - Closure can be pursued using PCR to target areas of the genome - Closed vs. draft genome - Closed genome relies on manpower - More expensive - More information - Annotation: converting raw sequence data into a list of genes present in the genome, and what they encode for - Annotation is "bottleneck" in genomics - Bioinformatics: science that applies powerful computational tools to DNA and protein sequences for the purpose of analysing, storing, and accessing the sequences for comparative purposes - Functional ORF: an open reading frame that encodes a protein - Computer algorithms used to search for ORFs - Look for start/stop codons and Shine–Dalgarno sequences - ORFs can be compared to ORFs in other organisms genomes - Majority of genes encode proteins * each organism has unique codon bias, the computer tests codon bias, if it matches with the organisms bias, its likely to be genuine - Number of genes with role that can be clearly identified in a given genome is 70% or less of total ORFs detected - Hypothetical proteins: uncharacterized ORFs; proteins that likely exist but whose function is currently unknown - Likely encode nonessential genes - In E. coli, many predicted to encode regulatory or redundant proteins - Noncoding RNA: RNA that does not code for protein - Usually are not conserved, so they are harder to find - Lack start codons and have multiple stop codons - Ex: (tRNA) (rRNA) Non Coding regulatory RNA molecules Class 15 Microbial genomes –genomics Genome Size and Content - Prokaryotic genomes range in size from those of large viruses to those of eukaryotic microbes - Unlike prokaryotes, eukaryotes contain a large fraction of noncoding DNA - Correlation between genome size and ORFs - As genome size increases, gene content proportionally increases in prokaryotes (linear positive relationship) not in eukaryotes - On average, a prokaryotic gene is 1,000 bp long - Smallest cellular genomes belong to parasitic or endosymbiotic prokaryotes - Obligate parasites range from 490 kbp - 4,400 kbp - Endosymbionts can be smaller (eg 160 kbp) - Estimates suggest the minimum # of genes for a viable cell is 250–300 genes - Largest prokaryotic genomes comparable to those of some eukaryotes - S. cellulosum (Bacteria)= Largest prokaryotic genome 12.3 Mbp - Largest archaeal genomes tend to be smaller (~5 Mbp) - Complement of genes in a particular organism defines its biology, but genomes are also moulded by an organism's lifestyle - Large genome /=/ complexity (some trees have larger than us!) - Gene distribution in prokaryotes - Metabolic genes typically are the most abundant class of genes; - Genes for protein synthesis also abundant; - Smaller genomes encode more translational processes; - Larger genomes encode more transcriptional regulation and signal transduction - DNA replication and translation genes make up minor fraction of genome - Evolution of Genomes - Gene Families, Duplications, and Deletions - Homologs: genes w/ related sequence due to common ancestry (homologous genes) - Gene families: groups of gene homologs - Paralogs: genes whose similarity is the result of gene duplication at some time in the evolution of an organism - Orthologs: genes found in one organism that are similar to those in another organism but differ because of speciation (evolution) (were derived from the same ancestor). Horizontal Gene Transfer and Genome Stability - Horizontal gene transfer - The transfer of genetic information between organisms, as opposed to vertical inheritance from parental organism(s) - May be extensive in nature - Genes are not native to the host – has been acquired - May cross phylogenetic domain boundaries - Uses transformation. Transduction, conjugation - Detecting horizontal gene flow - Presence of genes typically found only in distantly related species - Presence of a DNA with GC content or codon bias that differs significantly from remainder of genome - Gram positives are GC rich, gram negatives are AT rich - If a gram positive has more ATs (and vise versa) we can assume horizontal gene transfer - Horizontally transferred genes typically do not encode core molecular processes (they are not essential processes, are usually extra, nice ones) - The mobilome consists of all mobile genetic elements in a genome: - plasmids, prophages (integrated virus genomes), integrons, insertion sequences, transposons - They function to shuffle genes between species - transposons: mobile genetic elements that move between chromosomes, plasmids, and viruses via transposase - strong driver of genome evolution - may mediate large-scale chromosomal changes - Insertion sequences: Simple transposable elements whose genes encode only transposition - Organisms tend to lose these over time, as they are risky – cause genomic instability - Mobile elements promote genome evolution - Transposons may transfer DNA between different organisms - Transposons may also mediate large-scale chromosomal changes within a single organism - Presence of multiple insertion sequences (IS) - Recombination among identical IS can result in chromosomal rearrangements Examples: deletions, inversions, or translocations Core Genome versus Pan Genome - The "pan"/"core" concept: genomes of bacterial species consist of two components - Core genome: intrinsically shared by all strains of the species - Pan genome: includes all the optional, extrinsically gained extras present in some but not all strains of the species - Chromosomal islands - Region of bacterial chromosome of foreign origin that contains clustered genes for some extra property such as virulence or symbiosis - Pathogenicity islands: chromosomal islands containing genes for virulence - Chromosomal islands believed to have a "foreign" origin because: - Extra regions often flanked by inverted repeats - Base composition and codon usage in chromosomal islands often differ from rest of genome - Often found in some strains of a species but not others - Chromosomal islands contribute specialized functions not essential to growth - Virulence - Biodegradation of recalcitrant compounds - For example, hydrocarbons and herbicides - Symbiosis Functional Omics - The entire complement of RNA (transcriptome), proteins (translatome), or metabolites (metabolome) Metagenomics: - Metagenome (environmental genomics) - The total gene content of the organisms present in an environment (microbial community) - Several environments have been surveyed by large scale metagenomic projects - Examples: acid mine runoff waters, deep-sea sediments, fertile soils - Found that - Most genes from natural habitats are viral. - Most of the cellular DNA in natural habitats does not reside in living cells. Around 50-60% of the DNA in oceans is extracellular DNA. Marine sediment DNA is thought to be a major component of the global phosphorus cycle! Not inorganic phosphate like we thought! - “Biome” studies - The collection of prokaryotic cells that inhabit humans are called the human microbiome - the size of human microbiome is 10 times more than the size of human cells in a single person - Majority of these prokaryotes inhabit gut and majority belong to Bacteroidetes and Firmicutes - Microbiome correlates to the well being of the person, e.g. higher proportion of Firmicutes correlates to obesity - Fungi (60+) also are part of the microbiome, residing in the oral cavity and in the skin. Transcriptome - Transcriptome - The entire complement of RNA produced under a given set of conditions - To study transcriptome: Microarrays and DNA Gene chip - Small solid-state support to which genes or portions of genes are fixed and arrayed spatially in a known pattern - Hybridization techniques can be used in conjunction with fluorescence probes to measure gene expression - - What can be learned from microarray experiments? - Global gene expression - Expression of specific groups of genes under different conditions - Expression of genes with unknown function; can yield clues to possible roles - Comparison of gene content in closely related organisms; microbial diversity (e.g., PhyloChips) - Identification of specific organisms - To study transcriptome: RNA sequence analysis (RNA-seq) - All RNA molecules from a cell are sequenced - Measures: - expressed mRNA and How many copies of each RNA are made - Used with the DNA sequence, you can determine: - Which genes are transcribed - Discovers noncoding RNA regions - Identifies long untranslated regions - method of choice for global studies of gene expression - Can look at transcript abundance under different culture conditions - culturing previously uncultured bacteria by identifying highly transcribed genes and deducing usable nutrients (allows us to give it correct nutrients so we can culture it) Proteomics and the Interactome - Proteomics - Genome-wide study of the structure, function, and regulation of an organism's complement of proteins - In its wider sense proteome refers to all the proteins encoded by an organism’s genome - In its narrower sense, it refers to those proteins present in the cell at a given time (translatome) - Why look at proteomics when we already know the transcriptome (mRNA which makes these proteins)? - mRNA degrades quick, so sometimes when using transcriptome, we may be missing some possible protein products - Two-dimensional (2-D) polyacrylamide gel electrophoresis - Technique for separating, identifying, and measuring all proteins in a sample - May need to degrade some proteins with proteases, as its hard to sequence all proteins at once - In first (horizontal) dimension, proteins are separated by differences in isoelectric points - In second (vertical) dimension, proteins are separated by size - How is the Proteomics information used - Proteins with >50% sequence similarity typically have similar functions - Proteins with >70% sequence similarity almost certainly have similar functions - Protein domains - Distinct structural modules within proteins - Have characteristic functions that can reveal much about a protein's role, even in the absence of complete sequence homology - Utility of proteomics: an environmental case study - Metaproteomics of environmental samples shows collective metabolic potential of a community. - example: permafrost microbial communities and climate change Metabolomics - Metabolome - the complete set of metabolic intermediates and other small molecules produced in an organism - reflects enzymatic pathways - confirms reactions occurred - Advances in techniques: NIMS (Nanostructure-initiator mass spectrometry) - technically challenging due to immense chemical diversity - NIMS can directly analyse samples. - Utility of metabolomics - Plant biochemistry: plants produce several thousand metabolites. - secondary metabolites: scents, flavours, alkaloids, pigments - microbial cultures and communities (e.g., biofilms) - human microbiome Class 16 Human Microbiome Beneficial Human–Microbial Interactions - Humans have an extensive population of microorganisms: they outnumber the host cells by a factor of 10 to 100 (higher end is usually in gut) - including large populations of bacteria and fungi - Most microorganisms are not harmful - A number of them are beneficial and even necessary to health - Eg provide vital metabolites, and these metabolites may even control over microorganisms - They provide beneficial microbial products and inhibit growth of dangerous microorganisms. The host provides microenvironments for growth. - Due to competition. Normal flora have established growth, and can ‘win out’ against new, dangerous bacteria. - Normal microbial flora (“human microbiome”, “human microbiota”) - Microbiome: collective genomes of the microorganisms in an environment - Microbiota: the community of microorganisms themselves. - Why Study the Human Microbiome? - studies show that there are complex interactions between host and microbiota - People were concerned about bacteria in our bodies– tried to eliminate them. - But realised most were beneficial, and we can not eliminate them - Human microbiome studies focus on: - Do individuals share a core human microbiome? - If we share them, they are likely more important - Is there a correlation between the composition of microbiota colonising a body site and host genotype? - Do differences in our microbiome correlate w/ differences in health? - Are differences in the relative abundance of specific bacterial populations important to either health or disease? - Challenges studying human microbiota - Many microorganisms of human microbiota remain unculturable, but advances in sequencing have enabled metagenomic studies - We do not know their metabolic needs. We have cultured very few species in our microbiome (1-5%) - Metagenomic studies have enabled identification of nutrient requirements and possible culturing - Sequencing of 16s rRNA is used to identify microbial cell species of microbiota, in which >97% sequence similarity identifies 1 species. - However, not everyone follows similar sample treatment or sequence analysis for species identification. - Benefits of Knowing the Human Microbiome - development of biomarkers for predicting predisposition to diseases - Associated between our microbiome + predispositions - design targeted therapies, personalised drug therapies and probiotics - What do we know thus far about microbiota? - They contribute to: - Metabolic functions - We can use them to synthesise necessary vitamins, so why should we have a gene to synthesise it when we can use them to do it? - Protect against pathogens via competition (they are established and new dangerous bacteria are not, so our flora wins out) - Educate the immune system: helps mature our immune system - Through these basic functions microbiome affects directly or indirectly our physiology, both in heath and in disease - Do we share a core microbiome? - Each individual has their own microbial fingerprint at the species level (more so at strain level), but at larger taxonomic levels (phyla, families and genera), particular microbial groups typically dominate in all. - Microbiota matures within the first 3 yrs of the individual’s life, and it is retained (species composition) for the rest of one’s life. - Microbiota is very dynamic (high plasticity), many factors alter its composition (life style, diet, pets, antibiotics, etc) - Allows us to adjust easier. Since our genomes are rigid, and theres are constantly evolving, they can adapt for us! - Microbiota is resilient— when a disturbance factor stops the original microbiota emerges. - Taxonomic levels of Bacteria - Human microbiome – is there a correlation between composition of microbiota colonising a body site, and hosts geno/pheno type? - Human body offers a variety of ecosystems, each with unique physical parameters (pH, temp, and osmotic pressure, moisture) and nutrients - Eg skin, respiratory, GI tract have different microenvironments - Each body part provides a selective environment that favours growth of certain microorganisms and prevents growth of others. - Eg skin, respiratory, GI tract have different microorganisms Microbiota of the Skin - Scientists use the term microbiota to describe all the microbes in a microhabitat (e.g., skin microbiota). - The skin surface varies greatly in chemical composition and moisture content - Three microenvironments - Dry skin – mostly betaproteobacteria and corynebacteria - Moist skin – mostly corynebacteria and staphylococci - Sebaceous skin – mostly propionibacteria - Each microenvironment shows a unique microbiota - May be different in males and females due to differential gene expression - Normal skin microbiota - Skin microflora examined by molecular ecology methods: 19 bacterial phyla, about 200 genera and 850 species are detected. Eukaryotic microbes and Archaea are also present. - Most are gram positive (75%) actinobacteria and firmicutes, with 25% gram negatives, being proteobacteria and bacteroidetes Microbiota of the Oral Cavity - Mucous membrane lines the oral cavity and prevent the attachment of microbial cells - Saliva contains antimicrobial enzymes - But high concentrations of nutrients near surfaces in the mouth promote localized microbial growth - Bacteria colonise tooth surfaces by first attaching to acidic glycoproteins deposited there by saliva. - The oral cavity is a complex, heterogeneous microbial habitat - The tooth consists of a mineral matrix (enamel) surrounding living tissue (dentin and pulp) - Metagenomic analysis of human microflora shows a complex microbial community - Most microorganisms are facultatively aerobic - Some are obligately anaerobic - Some are obligately aerobic - The oral microbiome is as diverse as the gut, but humans share a greater proportion of common taxa for the mouth than for the gut. 750 species are identified in the oral cavity, with 7 major phyla - siliva - Mostly firmicutes, bacteroidetes, proteobacteria - Subgingival plaque - Bacteroidetes, actinobacteria, firmicutes, proteobacteria - Each part of the mouth interestingly has a different microbiome ! Microbiota of respiratory tract - A restricted group of organisms colonises the upper respiratory tract - Examples: staphylococci, streptococci, diphtheroid bacilli, and gram-negative cocci - Bacteria continually enter the upper respiratory tract from the air during breathing, from the nose. - Most are trapped in the mucus of the nasal and oral passages and expelled w/ nasal secretions or swallowed, then killed in the stomach. - The lower respiratory tract lacks naturally resident microbiota in healthy individuals - Is mostly sterile, sometimes have prevotella, streptococcus - Ciliated mucosal cells move particles up and out of the lungs. Microbiota of the Gastrointestinal Tract - The human gastrointestinal (GI) tract Consists of stomach (acidic), small intestine (basic), and large intestine (neutral) - Less microbiota in stomach (due to acid) and more in large intestine (neutral) - But still have diverse types which thrive in stomach - Responsible for digestion of food, absorption of nutrients, and production of nutrients by the indigenous microbiota, a surface of 400 m^2 - Contains 10^13 to 10^14 microbial cells with a low number of phyla - Mostly bacteroidaceae - Less are firmicutes - 2 anaerobic species=essential in digestion of plant based food - Lachnospiraceae and ruminococcaceae - Even less are Proteobacteria - Is called our second brain - The gut microbiota can impact our brain activity - Functions and products of intestinal microbiota - Intestinal microorganisms carry out a variety of essential metabolic reactions that produce various compounds (depending on the diet): - Vitamins, amino acids we can’t make - Gas, organic acids, and odor - Enzymes - The vast majority (~98 percent) of all human gut phylotypes fall into one of three major bacterial phyla: Firmicutes, Bacteroidetes, and Proteobacteria. - Individuals may have mostly Firmicutes, mostly Bacteroidetes, or a mix of the two. This may regulate metabolism and the host’s propensity for obesity. - The species diversity in the human gut is enormous (~35,000 species) - Obesity is linked to the ratio of firmicutes and bacteroidetes - Most organisms are restricted to the lumen of the large intestine, while others are in the mucosal layers. - Facultative aerobes, e.g. E. coli consume the remaining O2, rendering lumen anoxic, hence anaerobes will thrive, e.g. Clostridium and Bacteroides spp. - It takes 24 h for the passage of food through GI, during which the bacteria doubles once or twice - Gut Enterotypes (gut communities) – how much microbiota do humans share ? - While individuals vary in their gut microbiota, each individual has a relatively stable gut microbiota. - There are three basic enterotypes currently being studied: - #1 is enriched in Bacteroides (complex polysaccharides digestion) - #2 is enriched in Prevotella (cellulose digestion) - #3 is enriched in Ruminococcus (plant digestion) - Early studies indicate that each enterotype is functionally as well as phylogenetically distinct, and transcends national borders, ethnicity & nutrition - Products of Intestinal Microbiota and “Educating” the Immune System - Many microbial metabolites or transformation products that can be generated in the gut have significant influence on host physiology. - vitamin production - modification of steroids - Aa synthesis (and modifiers eg. tryptamine and 4-ethylphenylsulphate) - Production of polysaccharides as symbiosis factor - GI microbiota train the immune system - In early days of birth, are important in immune maturation - Excessive hygiene leads to issues (allergies, asthma, IBD etc) - Polysaccharide A production by Bacillus fragilis enables the gut to be tolerant towards species, and prevents colonisation of helicobacter hepaticus (cause of colitis) - Clostridium species also suppress inflammation and promote tolerance Development of the Human Microbiome From Birth to Death Human Study Groups - These studies are challenged by complexity of sampling - Contribution of host’s genetic background - Challenges in controlling diet and contributing lifestyle factors Human Study Groups and Animal Models - Human study groups form most of our knowledge of our microbiome - HMP determined baseline for healthy microbiome - But not diverse (all subjects were from USA) and lacked diet differences - Mouse models - Significant differences between us and mice, but have good use in studying human gut microbiome interactions - Mice have a larger cecum than humans. - Most fermentation is completed in the mouse cecum, rather than the human large intestine. - Good to use because Mice have a short life cycle and well-defined genetic lines; they can be raised in a germ-free environment. - antibiotic therapy - strict dietary control - faecal transplants (what does this do? We can check!) - germ-free environment (we can make sterile mice!) Colonisation, Succession, and Stability of the Gut Microbiota - Microbial Activities in the First Year of Life - Colonization begins at birth, with transfer from mother to infant. - In womb the baby is thought to be sterile - Early colonising microbes are a source of vitamins and tend to be facultative rather than obligate anaerobes. - Variables determine the nature of the gut microbiome. - Vaginally born infants have a microbiome more similar to that of their mothers than those born via c-section. - C sections have a microbiome similar to the nurse - Breastfed infants have more commensal bacteria, as breast milk has oligosaccharides that promote their colonization. - Stability of the Adult Microbiome and Transitions with Age - Early experiences determine gut microbiome. - Ageing and frailty are associated with decreased microbial diversity. - Firmicutes and proteobacteria are less stable members - Firmicutes abundance goes down w/ age - Living in communities increases species diversity – more contact with microbiome of others Disorders linked to human microbiota Disorders Attributed to the Gut Microbiota – do differences in biota contribute to health? - Inflammatory Bowel Disease (IBD) - chronic inflammation of the gut and disruption of homeostasis (dysbiosis). - Antibiotic use increases the risk of developing IBD. - Individuals with IBD have lower gut microbiome diversity. - Once developed, IBD may be transmissible between family members - The Role of the Gut Microbiota in Obesity: - Mouse Models - Normal mice have 40 percent more fat than germ-free mice with the same diet. When germ-free mice were given normal mouse microbiota, they started gaining weight. - Mice that are genetically obese have different microbiota than normal mice. Obese mice have more Firmicutes - We can take microbiome of obese people, put it into mice, and the mice will become obese, even with a low fat diet - Like the mouse model, obese humans have more Prevotella and methanogenic Archaea than non-obese humans, which seem to play a similar role as the Firmicutes and methanogenic Archaea in obese mice. - Not everything can be explained by the above rationale, e.g. lean mice digest more plant fiber, and produce certain volatile fatty acids in large amounts. - The nature and transferability of gut microbiota is dependent on diet as well genetics. - The host’s physiology can also modulate the gut microbiota Modulators of human microbiota Antibiotics and the Human Microbiome - Oral antibiotics decrease ALL microbes in the human gut (both target and non-target). - Use of antibiotics during the first few months of life increases the risk of developing IBD and other disorders related to dysbiosis. - Clostridium difficile infections are associated with antibiotic use. - Clostridium difficile is a spore-former and generally antibiotic resistant. - A newer therapy for Clostridium difficile infection is a faecal transplant. - Since antibiotics kill healthy microbiota, and c diff becomes resistant to antibiotics FAST, so when the antibiotic is removed, it is actually in higher amounts – it is very resilient Probiotics and Prebiotics - Probiotics are live organisms that confer a health benefit to the host. - Species of Bifidobacterium and Lactobacillus bacteria are commonly used probiotics found in yoghurt and probiotic drinks. - They may work by taking up space or nutrients, limiting the ability of pathogens to colonise the gut. - Prebiotics are typically carbohydrates that are indigestible by human hosts, but provide nutrition for fermentative gut bacteria.