Microbial Growth And Control PDF
Document Details
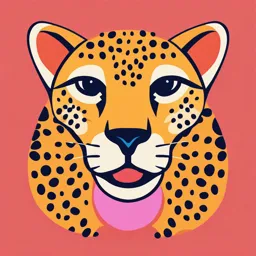
Uploaded by StunnedSwaneeWhistle
Lebanese American University
Charbel Al Khoury
Tags
Summary
This document covers microbial growth and its control, specifically focusing on temperature. It describes temperature classes, microbial life in the cold, and microbial life at high temperatures. The document is part of a microbiology lecture series.
Full Transcript
BIO 311 - Microbiology Chapter 5 Microbial Growth and Its Control Prepared by Charbel Al Khoury, Ph.D. [email protected] Lebanese American University M...
BIO 311 - Microbiology Chapter 5 Microbial Growth and Its Control Prepared by Charbel Al Khoury, Ph.D. [email protected] Lebanese American University Microbial Growth and Its Control III. Environmental Effects on Growth: Temperature 9. Temperature Classes of Microorganisms 10. Microbial Life in the Cold 11. Microbial Life at High Temperatures 9. Temperature Classes of Microorganisms Four environmental factors control microbial growth in a major way: temperature, pH, water availability, and oxygen. If any one of these factors is beyond the limits that an organism can tolerate, growth will not occur, even in an ideal culture medium. Temperature is a major environmental factor controlling microbial growth. At either too cold or too hot a temperature, microorganisms will not be able to grow and may even die. The minimum and maximum temperatures supporting growth vary greatly among different organisms and usually reflect the temperature range and average temperature of the environments the organisms inhabit. Cardinal temperatures: the minimum, optimum, and maximum temperatures at which an organism grows. The cardinal temperatures are characteristic for any given microorganism and can differ dramatically between species The temperature range (from minimum to maximum) is less than 40º C for a particular organism. Temperature affects microorganisms in two opposing ways. As temperatures rise, the rate of enzymatic reactions increases and growth becomes faster. However, above a certain temperature, proteins or other cell components may be denatured or otherwise irreversibly damaged. For every microorganism there is a minimum temperature below which growth is not possible, an optimum temperature at which growth is most rapid, and a maximum temperature above which growth is not possible. These three temperatures, called the cardinal temperatures Figure 1. The cardinal temperatures: minimum, optimum, and maximum the growth temperature optimum reflects a state in which all or most cellular components are functioning at their maximum rate and typically lies closer to the maximum than to the minimum The factors controlling an organism’s minimum growth temperature are not as clear. The maximum growth temperature of an organism reflects the However, the cytoplasmic membrane must remain in a semifluid state for nutrient temperature above which denaturation of one or more essential cell transport and bioenergetic functions to take place. That is, if an organism’s components, such as a key enzyme, occurs. cytoplasmic membrane stiffens to the point that it no longer functions properly in transport or can no longer develop or consume a proton motive force, the organism cannot grow. 9. Temperature Classes of Microorganisms Temperature Classes of Organisms Microorganisms can be classified into groups by their growth temperature optima. 4 groups low temperature optima psychrophile: low, found in cold environments midrange temperature optima mesophile: midrange, most commonly studied high temperature optima thermophile: high, found in hot environments thermophiles are found in unusually hot environments very high temperature optima hyperthermophile: very high, found in extremely hot habitats (as high as 100º C) such as hot springs and deep-sea hydrothermal vents Mesophiles are widespread in nature and are the most commonly studied microorganisms. Mesophiles are found in the intestines of endothermic (warm-blooded) animals and in terrestrial and aquatic environments in temperate and tropical latitudes. Escherichia coli is a typical mesophile 10. Microbial Life in the Cold Extremophiles organisms that grow under very hot or very cold conditions Psychrophiles Psychrophiles are found in unusually cold environments organisms with optimal growth temperature ≤ 15°C, maximum ≤ 20°C, minimum ≤ 0°C inhabit constantly cold environments are constantly cold and may even be killed by warming to moderate temperatures Psychrotolerant organisms that can grow at 0°C but have optima of 20°C to 30°C More widely distributed in nature than psychrophiles isolated from soils and water in temperate climates and food at 4°C Figure 1. Antarctic microbial habitats and microorganisms 10. Microbial Life in the Cold Molecular adaptations to life in the cold production of enzymes that function optimally in the cold (may be denatured at moderate temperatures) The behavior is linked to the protein structure more α-helices than β-sheets → greater flexibility for reaction Enzymes with more polar amino catalysis at cold temperatures Because -sheet secondary structures tend to be more rigid than -helices, the greater -helix content of cold-active enzymes allows these proteins greater flexibility for catalyzing their reactions at cold temperatures. acids tend to remain flexible and more polar and fewer hydrophobic amino acids active at lower temperatures. Polar Lesser hydrophobic amino acids contribute to this flexibility, as amino acids can interact with hydrophobic interactions tend to stabilize structures. A flexible water, which is abundant in cold structure allows cold-active enzymes to maintain activity even environments, helping to maintain when the surrounding environment is less energetic (i.e., at lower enzyme solubility and function. temperatures). fewer weak bonds (e.g., hydrogen and ionic bonds) Fewer stabilizing interactions allow the enzyme to be more flexible, which is crucial for facilitating catalysis at lower temperatures. 10. Microbial Life in the Cold Molecular adaptations to life in the cold Cytoplasmic membranes function at low temperatures. Shorter fatty acid chains have fewer carbons, which reduces the overall hydrophobic interactions in the membrane. This results in a lower melting point, meaning the membrane remains fluid and functional at lower temperatures. high unsaturated and shorter-chain fatty acid content this helps the membrane remain in a semifluid state at low temperatures to carry out important transport and bioenergetic functions. Unsaturated fatty acids contain one or more double bonds, which create kinks in the fatty acid chains. This prevents the molecules from packing closely together, maintaining membrane fluidity even at low temperatures. some polyunsaturated fatty acids, which remain flexible at very low temperatures unlike monounsaturated or fully saturated fatty acids that tend to stiffen at low temperatures, polyunsaturated fatty acids remain flexible even at very cold temperatures. cold shock proteins (chaperones) have several functions that include maintaining cold-sensitive proteins in an active form or binding specific mRNAs and facilitating their translation under cold conditions. Cryoprotectants (e.g., antifreeze proteins) prevent formation such as glycerol or other sugars of ice crystals. that can puncture the cytoplasmic membrane. exopolysaccharide cell surface slime (cryoprotection) Highly psychrophilic bacteria often produce abundant levels of exopolysaccharide cell surface slime, and these slime layers confer cryoprotection as well. 11 Microbial Life at High Temperatures Thermophiles: organisms with growth temperature optima between 45°C and 80°C Hyperthermophiles: organisms with optima greater than 80°C inhabit hot environments, including boiling hot springs and seafloor hydrothermal vents, that can experience temperatures in excess of 100°C Above 65°C, only prokaryotic life forms thrive, but extensive diversity present (Table 1) Table 1. Presently known upper temperature limits for growth of living organisms 11. Microbial Life at High Temperatures Hyperthermophiles in hot springs (Figure 1) generation times (g) as low as one hour high prokaryotic diversity (both Archaea and Bacteria represented) Some hyperthermophilic Archaea have growth-temperature optima above 100°C, while no species of Bacteria have yet been discovered that grow above 95°C Figure 1. Growth of hyperthermophiles in boiling water 11. Microbial Life at High Temperatures Thermophiles inhabit moderately hot or intermittently hot environments. Thermal gradients form along the edges of hot environments. Distribution of microbial species along the gradient is dictated by organism's biology. As boiling water leaves a hot spring, it gradually cools, setting up a thermal gradient. Along this gradient, microorganisms become established, with different species growing in the different temperature ranges 11. Microbial Life at High Temperatures Protein and membrane stability at high temperatures Enzymes and proteins function optimally at high temperatures, features that provide thermal stability. Critical amino acid substitutions in a few locations provide more heat-tolerant folds. The heat stability of an enzyme from a hyperthermophile is often due to subtle changes in amino acid sequence from the corresponding enzyme from a mesophile, and these changes affect protein structure and function to resist heat denaturation. Increased number of ionic bonds between basic and acidic amino acids resists unfolding. highly hydrophobic interiors. factors that prefer unfolding Production of solutes (e.g., di-inositol phosphate, diglycerol phosphate) helps stabilize proteins. from denaturation. enzymes commercially useful prolong shelf life, (e.g., Taq polymerase for polymerase chain reaction [PCR]) 11. Microbial Life at High Temperatures Protein and membrane stability at high temperatures modifications in cytoplasmic membranes to ensure heat stability Heat naturally works to peel apart the lipid bilayer that makes up the cytoplasmic membrane Bacteria have lipids rich in long-chain and saturated fatty acids, Saturated fatty acids form a stronger hydrophobic environment than do unsaturated fatty acids, and longer-chain fatty acids fewer unsaturated fatty acids. have a higher melting point than shorter-chain fatty acids; collectively, these properties increase membrane stability Hyperthermophiles, most of which are Archaea, do not contain fatty acids in their membranes but instead have C40 hydrocarbons composed of repeating units of isoprene bonded by ether linkage to glycerol phosphate ( Archaea have C40 hydrocarbons made of repeating isoprene units bonded to glycerol phosphate, and membrane forms lipid monolayer rather than bilayer. The membrane forms a lipid monolayer rather than a lipid bilayer. The monolayer structure covalently links both halves of the membrane and prevents it from melting at the high growth temperatures of hyperthermophiles. THE END