Microbial Physiology Lecture Module 13 PDF
Document Details
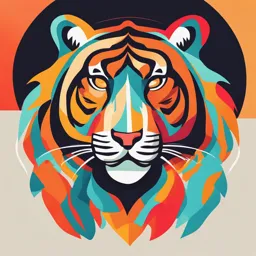
Uploaded by FertileLapisLazuli7229
Polytechnic University of the Philippines
Tags
Summary
This document is a lecture module on microbial growth and its control, focusing on environmental effects such as pH, osmolarity, and oxygen. It details how these factors impact microbial growth and provides examples to illustrate these concepts. It has a table of pH values and examples and details how this works for microbes.
Full Transcript
Microbial Growth and Its Control: Environmental Effects on Growth: pH, Osmolarity, Oxygen Module 13 Week 7 OVERVIEW: Temperature has a major effect on the growth of microorganisms. But many other environmental factors can affect microbial growth as well, including pH,...
Microbial Growth and Its Control: Environmental Effects on Growth: pH, Osmolarity, Oxygen Module 13 Week 7 OVERVIEW: Temperature has a major effect on the growth of microorganisms. But many other environmental factors can affect microbial growth as well, including pH, osmolarity, and oxygen. After successful completion of this module, you should be able to: 1.Understand the reasons why different organisms vary with regards to their environmental condition requirements; and 2.Acquainted with the with the environmental factors and their effects on the growth of microbes. Other Environmental Factors Affecting Growth Acidity and Alkalinity Osmotic Effects on Microbial Growth Oxygen and Microorganisms Toxic Forms of Oxygen © 2012 Pearson Education, Inc. Effects of pH on Microbial Growth Acidity or alkalinity of a solution is expressed by its pH on a logarithmic scale in which neutrality is pH 7. pH values less than 7 are acidic and those greater than 7 are alkaline. Figure 5.24 pH Example Moles per liter of: H OH 1 1014 Volcanic soils, waters 101 1013 Gastric fluids Acidophiles Lemon juice Acid mine drainage 102 1012 Vinegar Increasing Rhubarb 103 1011 acidity Peaches Acid soil 104 1010 Tomatoes American cheese 105 109 Cabbage Peas 106 108 Corn, salmon, shrimp Neutrality Pure water 107 107 Seawater 108 106 Very alkaline 109 105 natural soil Alkaliphiles Alkaline lakes 1010 104 Increasing Soap solutions alkalinity Household ammonia 1011 103 Extremely alkaline soda lakes 1012 102 Lime (saturated solution) 1013 101 1014 1 © 2012 Pearson Education, Inc. Effects of pH on Microbial Growth Organisms that grow optimally at a pH value in the range termed circumneutral (pH 5.5 to 7.9) are called neutrophiles. Organisms that grow best below pH 5.5 are called acidophiles. Microorganisms showing pH optima of 8 or higher are called alkaliphiles. The pH of an environment greatly affects microbial growth Some organisms have evolved to grow best at low or high pH, but most organisms grow best between pH 6 and 8 (neutrophiles) © 2012 Pearson Education, Inc. Acidophiles: organisms that grow best at low pH (9) Some have sodium motive force rather than proton motive force Cytoplasmic pH and Buffers The internal pH of a cell must stay relatively close to neutral even though the external pH is highly acidic or basic Internal pH has been found to be as low as 4.6 and as high as 9.5 in extreme acido- and alkaliphiles, respectively © 2012 Pearson Education, Inc. Acidity and Alkalinity Microbial culture media typically contain buffers to maintain constant pH © 2012 Pearson Education, Inc. Osmolarity and Microbial Growth Water is the solvent of life, and water availability is an important factor affecting the growth of microorganisms. Osmotic Effects on Microbial Growth Water activity (aw): water availability; expressed in physical terms Defined as ratio of vapor pressure of air in equilibrium with a substance or solution to the vapor pressure of pure water © 2012 Pearson Education, Inc. Osmotic Effects on Microbial Growth Typically, the cytoplasm has a higher solute concentration than the surrounding environment, thus the tendency is for water to move into the cell (positive water balance) When a cell is in an environment with a higher external solute concentration, water will flow out unless the cell has a mechanism to prevent this © 2012 Pearson Education, Inc. Osmotic Effects on Microbial Growth Halophiles: organisms that grow best at reduced water potential; have a specific requirement for NaCl (Figure 5.25) Extreme halophiles: organisms that require high levels (15–30%) of NaCl for growth Halotolerant: organisms that can tolerate some reduction in water activity of environment but generally grow best in the absence of the added solute © 2012 Pearson Education, Inc. Figure 5.25 Halotolerant Halophile Extreme Example: Example: halophile Staphylococcus Aliivibrio fischeri Example: aureus Halobacterium salinarum Growth rate Nonhalophile Example: Escherichia coli 0 5 10 15 20 NaCl (%) © 2012 Pearson Education, Inc. Osmotic Effects on Microbial Growth Osmophiles: organisms that live in environments high in sugar as solute Xerophiles: organisms able to grow in very dry environments © 2012 Pearson Education, Inc. Osmotic Effects on Microbial Growth Mechanisms for combating low water activity in surrounding environment involve increasing the internal solute concentration by Pumping inorganic ions from environment into cell Synthesis or concentration of organic solutes compatible solutes: compounds used by cell to counteract low water activity in surrounding environment © 2012 Pearson Education, Inc. Oxygen and Microbial Growth Oxygen (O2) is an essential nutrient for many microbes; they are unable to metabolize or grow without it. Other microbes, by contrast, cannot grow in the presence of O2 and may even be killed by it. There are different classes of microorganisms based on their needs or tolerance of O2. Oxygen and Microorganisms Aerobes: require oxygen to live Anaerobes: do not require oxygen and may even be killed by exposure Facultative organisms: can live with or without oxygen Aerotolerant anaerobes: can tolerate oxygen and grow in its presence even though they cannot use it Microaerophiles: can use oxygen only when it is present at levels reduced from that in air © 2012 Pearson Education, Inc. Culture Techniques for Aerobes For the growth of aerobes, it is necessary to provide extensive aeration. This is because the O2 that is consumed by the organisms during growth is not replaced fast enough by diffusion from the air. Therefore, forced aeration of liquid cultures is needed and can be achieved by either vigorously shaking the flask or tube on a shaker or by bubbling sterilized air into the medium through a fine glass tube or porous glass disc. Culture Techniques for Anaerobes For the culture of anaerobes, the problem is not to provide O2 but to exclude it. Bottles or tubes filled completely to the top with culture medium and fitted with leakproof closures provide suitably anoxic conditions for organisms that are not overly sensitive to small amounts of O2. A chemical called a reducing agent may be added to such vessels to remove traces of O2 by reducing it to water (H2O). An example is thioglycolate, which is present in thioglycolate broth, a medium commonly used to test an organism’s requirements for O2. Read: Brock Biology of Microorganisms 15th Edition (2019) pp. 196-200 Oxygen and Microorganisms Special techniques are needed to grow aerobic and anaerobic microorganisms (Figure 5.27) Reducing agents: chemicals that may be added to culture media to reduce oxygen (e.g., thioglycolate) © 2012 Pearson Education, Inc. Oxygen and Microorganisms Thioglycolate broth Complex medium that separates microbes based on oxygen requirements Reacts with oxygen so oxygen can only penetrate the top of the tube © 2012 Pearson Education, Inc. Growth versus o concentration. From left to right, aerobic, anaerobic, facultative, Oxic zone microaerophilic, and aerotolerant anaerobe growth, Anoxic zone as revealed by the position of microbial colonies (depicted here as black dots) within tubes of thioglycolate broth culture medium. Figure 5.27 © 2012 Pearson Education, Inc. Toxic Forms of Oxygen Several toxic forms of oxygen can be formed in the cell (Figure 5.28): Single oxygen Superoxide anion Hydrogen peroxide Hydroxyl radical © 2012 Pearson Education, Inc. Figure 5.28 Reactants Products (superoxide) (hydrogen peroxide) (hydroxyl radical) (water) Outcome: © 2012 Pearson Education, Inc. Toxic Forms of Oxygen Enzymes are present to neutralize most of these toxic oxygen species (Figure 5.29) Catalase (Figure 5.30) Peroxidase Superoxide dismutase Superoxide reductase © 2012 Pearson Education, Inc. Figure 5.29 Catalase Peroxidase Superoxide dismutase Superoxide dismutase/catalase in combination Superoxide reductase © 2012 Pearson Education, Inc. Figure 5.30 © 2012 Pearson Education, Inc. ACTIVITIES/ASSESSMENTS (40 pts.): Answer the following questions in not more than 50 words. Write your answers in a yellow paper. 1.What terms are used to describe organisms whose growth pH optimum is very high? Very low? 2.What are compatible solutes, and when and why are they needed by the cell? What is the compatible solute of Halobacterium? 3.How does an obligate anaerobe differ from a facultative anaerobe? 4.How does superoxide dismutase or superoxide reductase protect a cell? Microbial Growth and Its Control: Control of Microbial Growth Module 14 Week 8 OVERVIEW: Many aspects of microbial growth control have significant practical applications. For example, washing of hands remove attached microorganisms and inhibit microbial growth on body surfaces. However, neither of these processes kills or removes all microorganisms. Only sterilization—the killing or removal of all microorganisms (including viruses)—ensures that this is the case. LEARNING OUTCOMES: After successful completion of this module, you should be able to: 1.Identify the general principles of controlling microbial growth; and 2.Describe different techniques of microbial growth control. Terminology Sterilization: Removal of all microbial life Commercial sterilization: Killing C. botulinum endospores Disinfection: Removal of pathogens Antisepsis: Removal of pathogens from living tissue Degerming: Removal of microbes from a limited area Sanitization: Lower microbial counts on eating utensils Biocide/Germicide: Kills microbes General Principles and Growth Control by Heat The effects of microorganisms can often be controlled by simply limiting or inhibiting growth. Methods for inhibiting microbial growth include decontamination, the treatment of an object or surface to make it safe to handle, and disinfection, a process that directly targets pathogens although it may not eliminate all microorganisms. Physical methods of microbial growth control such as heat, radiation, and filtration are used extensively in industry, medicine, and the home Heat Sterilization Heat Sterilization The effectiveness of heat as a sterilant is quantified by the time required for a 10- fold reduction in the viability of a microbial population at a given temperature. This is called the decimal reduction time (D). Decimal Reduction Time (DRT) Minutes to kill 90% of a population at a given temperature Table 7.2 Heat Thermal death point (TDP): Lowest temperature at which all cells in a culture are killed in 10 min. Thermal death time (TDT): Time to kill all cells in a culture General Principles and Growth Control by Heat Of these three, heat is the most widely used method of physically treating an object or substance to render it sterile. The autoclave is a sealed heating device that uses steam under pressure to kill microorganisms. Killing of heat-resistant endospores requires heating at temperatures above the boiling point of water at 1 atm. The autoclave places steam under a pressure of 1.1 kg/cm2 (15 lb/in2), which yields a temperature of 121°C. At 121°C, the time to achieve sterilization of small amounts of endospore- containing material is about 15 min Heat Moist heat denatures proteins Autoclave: Steam under pressure Figure 7.2 Steam Sterilization Steam must contact item’s surface. Figure 7.3 General Principles and Growth Control by Heat Pasteurization, a process named for Louis Pasteur, is not the same as sterilization. Pasteurization uses heat to significantly reduce rather than totally eliminate the microorganisms found in liquids, such as milk. Pasteurization reduces spoilage organisms and pathogens Equivalent treatments 63°C for 30 min High temperature, short time: 71°C for 15 min Ultra high temperature: 135°C for 1-2 sec Thermoduric organisms survive At the temperatures and times standardized for the pasteurization of food products, all known pathogenic bacteria are killed. In addition, however, by decreasing the overall microbial load, pasteurization increases the shelf life of perishable liquids. Other Physical Control Methods: Radiation In addition to heat, radiation, in particular ultraviolet (UV) light, X- rays, and gamma rays, are also effective microbial killing agents. However, each type of energy has a different mode of action and killing efficacy and thus their applications vary widely. Radiation damages DNA Ionizing radiation (X rays, gamma rays, electron beams) Nonionizing radiation (UV) (Microwaves kill by heat; not especially antimicrobial) Figure 7.5 On the other hand, heat is an effective way to decontaminate most liquids, but heat-sensitive liquids not subject to ionizing radiation are typically sterilized by filtration. For sterilization, a filter with pores of average size 0.2 μm is a minimum requirement; however, even such tiny holes will not trap most viruses. Filtration removes microbes Low temperature inhibits microbial growth Refrigeration, Deep freezing, Lyophilization High pressure denatures proteins Desiccation prevents metabolism Osmotic pressure causes plasmolysis Chemical Control of Microbial Growth Chemicals are routinely used to control microbial growth, and an antimicrobial agent is a natural or synthetic chemical that kills or inhibits the growth of microorganisms. Agents that actually kill are called -cidal agents, with a prefix indicating the type of micro- organism killed. Thus, bactericidal, fungicidal, and viricidal agents kill bacteria, fungi, and viruses, respectively. Agents that do not kill but only inhibit growth are called -static agents, and include bacteriostatic, fungistatic, and viristatic compounds. Chemical Control of Microbial Growth Antibacterial agents are classified as -static, -cidal, or -lytic (cell lysing). Bacteriostatic agents are typically inhibitors of some important biochemical process, such as protein synthesis, and bind relatively weakly; if the agent is removed, the cells can resume growing. Bactericidal agents bind tightly to their cellular targets and by definition kill the cell. However, the dead cells are not lysed, and total cell numbers, reflected in the tur- bidity of the culture, remain constant. Bacteriolytic agents kill cells by lysing them, and this affects both viable and total cell numbers. Chemical Control of Microbial Growth Antimicrobial activity can be measured by determining the smallest amount of the agent needed to inhibit the growth of a test organism, a value called the minimum inhibitory concentration (MIC). One way to determine the MIC of a given agent is to inoculate a series of tubes of liquid growth medium containing a test organism and dilutions of the agent. Chemical Control of Microbial Growth Antimicrobial activity can also be assessed using solid media. Known amounts of an antimicrobial agent are added to filter-paper discs and the discs are arranged on the sur- face of a uniformly inoculated agar plate. During incubation, the agent diffuses from the disc into the agar, establishing a gradient; the farther a chemical diffuses away from a disc, the lower its concentration. Following an incubation period, a zone of growth inhibition forms around discs that released effective chemicals;. Chemical Methods of Microbial Control Evaluating a disinfectant Disk-diffusion method Figure 7.6 Chemical Antimicrobial Agents Sterilants destroy all microorganisms, including endospores. Chemical sterilants are used for decontamination or sterilization in situations where it is impractical or impossible to use heat or radiation. Disinfectants are chemicals that kill microorganisms but not necessarily endospores and are primarily used on surfaces. Sanitizers, by contrast, are less harsh than disinfectants and reduce microbial numbers but do not sterilize. Antiseptics, often called germicides, are chemicals that kill or inhibit the growth of micro- organisms but are sufficiently nontoxic to animals to be applied to living tissues. Most germicides are used for hand washing or for treating surface wounds Table 7.7 Figure 7.11 ACTIVITIES/ASSESSMENTS (50 pts.): Answer the following questions in not more than 50 words. Write your answers in a yellow paper. 1.Why is heat an effective sterilizing agent? 2.What steps are necessary to ensure the sterility of material contaminated with bacterial endospores? 3.Why is ionizing radiation more effective than UV radiation for sterilization of food products? 4.Distinguish between the antimicrobial effects of -static, -cidal, and -lytic agents. 5.Describe how the minimum inhibitory concentration of an antibacterial agent is determined. Antimicrobial Drugs Chemotherapy The use of drugs to treat a disease Antimicrobial drugs Interfere with the growth of microbes within a host Antibiotic Substance produced by a microbe that, in small amounts, inhibits another microbe Selective toxicity A drug that kills harmful microbes without damaging the host 1928 – Fleming discovered penicillin, produced by Penicillium. 1940 – Howard Florey and Ernst Chain performed first clinical trials of penicillin. Figure 20.1 Table 20.1 Table 20.2 The Action of Antimicrobial Drugs Broad-spectrum Superinfection Bactericidal Bacteriostatic The Action of Antimicrobial Drugs Figure 20.2 The Action of Antimicrobial Drugs Figure 20.4 Antibacterial Antibiotics Inhibitors of Cell Wall Synthesis Penicillin Natural penicillins Semisynthetic penicillins Penicillins Figure 20.6 Antibacterial Antibiotics Inhibitors of Cell Wall Synthesis Penicillin Penicilinase-resistant penicillins Extended-spectrum penicillins Penicillins + -lactamase inhibitors Carbapenems Monobactam Antibacterial Antibiotics Inhibitors of Cell Wall Synthesis Figure 20.8 Antibacterial Antibiotics Inhibitors of Cell Wall Synthesis Cephalosporins 2nd, 3rd, and 4th generations more effective against gram-negatives Figure 20.9 Antibacterial Antibiotics Inhibitors of Cell Wall Synthesis Polypeptide antibiotics Bacitracin Topical application Against gram-positives Vancomycin Glycopeptide Important "last line" against antibiotic resistant S. aureus Antibacterial Antibiotics Inhibitors of Cell Wall Synthesis Antimycobacterium antibiotics Isoniazid (INH) Inhibits mycolic acid synthesis Ethambutol Inhibits incorporation of mycolic acid Antibacterial Antibiotics Inhibitors of Protein Synthesis Chloramphenicol Broad spectrum Binds 50S subunit, inhibits peptide bond formation Aminoglycosides Streptomycin, neomycin, gentamycin Broad spectrum Changes shape of 30S subunit Antibacterial Antibiotics Inhibitors of Protein Synthesis Tetracyclines Broad spectrum Interferes with tRNA attachment Macrolides Gram-positives Binds 50S, prevents translocation Erythromycin Gram-positives Binds 50S, prevents translocation Antibacterial Antibiotics Inhibitors of Protein Synthesis Streptogramins Gram-positives Binds 50S subunit, inhibits translation Synercid Gram-positives Binds 50S subunit, inhibits translation Oxazolidinones Linezolid Gram-positives Binds 50S subunit, prevents formation of 70S ribosome Antibacterial Antibiotics Injury to the Plasma Membrane Polymyxin B Topical Combined with bacitracin and neomycin in over- the-counter preparation Antibacterial Antibiotics Inhibitors of Nucleic Acid Synthesis Rifamycin Inhibits RNA synthesis Antituberculosis Quinolones and fluoroquinolones Ciprofloxacin Inhibits DNA gyrase Urinary tract infections Antibacterial Antibiotics Competitive Inhibitors Sulfonamides (Sulfa drugs) Inhibit folic acid synthesis Broad spectrum Figure 5.7 Figure 20.13