CHM303 Main Group Chemistry Lectures 18-23 PDF
Document Details
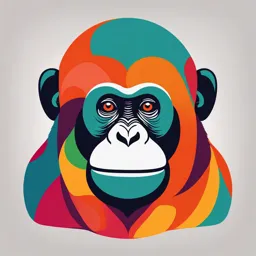
Uploaded by WealthyLaplace953
IISER Mohali
Dr. Kuduva R. Vignesh
Tags
Related
- CHM 102 (Inorganic Chemistry) 2019-2020 Notes PDF
- CY - Chemistry GATE Exam Syllabus PDF
- CHEM1910 Inorganic Lecture 1 Introduction PDF
- Main Group Lecture Notes (CSIR NET/GATE, Chemical Sciences) PDF
- JEE Main 2025 Revision Notes on Classification of Elements and Periodicity
- Inorganic Chemistry (Chemistry of Main Group Elements) PDF
Summary
These lecture notes cover main group chemistry, focusing on boron halides and heavier group 13 elements (Al, Ga, In, Tl). The provided materials include details on structures, thermal stability, reactivity, and more. The notes are presented in a lecture/study format.
Full Transcript
“CHM303” Main group chemistry Dr. Kuduva R. Vignesh Email: [email protected] Lower halides of boron: B2X4 B2X4 mp and bp in ℃ B 2 F4 -56 and -34...
“CHM303” Main group chemistry Dr. Kuduva R. Vignesh Email: [email protected] Lower halides of boron: B2X4 B2X4 mp and bp in ℃ B 2 F4 -56 and -34 B2Cl4 -92.6 and 65.5 B2Br4 Liquid (unstable at RT) B 2 I4 Pale yellow solid Planar D2h for B2F4 and solid B2Cl4 Isoelectronic to C2O42- and N2O4 staggered D2d for gaseous B2Cl4 and B2Br4 Thermal stability of these compounds follow the expected sequence of pπ-pπ bonding between the X group and B: B2(NMe2)4 > B2(OMe)4 > B2(OH)4 > B2F4 > B2Cl4 > B2Br4 Thermolysis of B2C14 and B2Br4 at moderate temperatures gives a series of closo-halogenoboranes BnXn where n = 4, 8-12 for C1, and n = 7-10 for Br. Other preparative routes include the high-yield halogenation of B9H92- to B9X92- using N- chlorosuccinimide, N-bromosuccinimide or I2. B4Cl4 is a pale-yellow-green solid, has a regular closo-tetrahedral structure. The family of BnXn (X = Cl, Br, I) molecules possess cluster structures. (a) B4Cl4 has a tetrahedral core, (b) B8Cl8 possesses a dodecahedral cluster core and (c) B9Br9 has a tricapped trigonal prismatic core. Simple trihalides of heavier group 13 elements (Al, Ga, In, and Tl) The trifluorides of Al, Ga, In and Tl are non-volatile solids, best prepared by fluorination of the metal (or one of its simple compounds) with F2. AlF3 is also prepared by the reaction below: Each trifluoride is highly melting and has an infinite structure. In AlF3, each Al centre is octahedral, surrounded by six F atoms, each of which links two Al centres. Cryolite, Na3[AlF6] occurs naturally but can also be synthesized to meet commercial needs. The solid state structure of cryolite is related to the perovskite- type structure. Compounds MX3 (M = Al, Ga or In; X = Cl, Br or I) are obtained by direct combination of the elements. They are relatively volatile and in the solid state possess layer structures or structures containing dimers M2X6. Solid AlCl3 adopts a layer structure with octahedral geometry around Al centers. The vapours consist of dimeric molecules and these are also present in solutions of the compounds in inorganic solvents. Only at high temperatures does dissociation to monomeric MX3 occur. In the monomer, the group 13 metal is trigonal planar, but in the dimer, a tetrahedral environment results from X→M coordinate bond formation involving a halogen lone pair of electrons A representation of the bonding in Al2Cl6 showing the Cl lone pair donation to Al. The structure of Al2Cl6 with bond distances determined in the vapour phase; the terminal MX bond distances are similarly shorter than the bridging distances in Al2Br6, Al2I6, Ga2Cl6, Ga2Br6, Ga2I6 and In2I6. In AlCl3 monomer, the AlCl distances are 206 pm. Part of one layer of crystalline AlCl3 viewed from above, showing Cl atoms bridging between octahedral Al atoms. The layer structure of solid AlCl3 weak van der Waals forces operate between layers. Reactivity H2O vigorously reacts with solid AlCl3, and hydrolysis occurs, but in dilute aq. solution, [Al(OH2)6]3+ and Cl- ions are present. In coordinating solvents such as Et2O, AlCl3 forms adducts such as Et2O→AlCl3. With NH3, AlX3 (X = Cl, Br, I) forms H3N→AlX3, and in the solid state intermolecular hydrogen bonding involving NH‧‧‧‧X interactions are present. Gallium and indium trichlorides and tribromides also form adducts, but with coordination numbers of 4, 5 or 6: [MCl6]3-, [MBr6]3-, [MCl5]2-, [MCl4]- and [MBr4]- (M = Ga or In) and L→GaX3 or L3→InX3 (L = neutral Lewis base). The square-based pyramidal structure of [Et4N]+[InCl5]2- has been confirmed by X-ray diffraction. This is not expected by VSEPR arguments, but one must bear in mind that energy differences between 5- coordinate geometries are often small and preferences can be tipped by, for example, crystal packing forces. The Tl(III) halides are less stable than those of the earlier group 13 elements. TlCl3 and TlBr3 are very unstable with respect to conversion to the Tl(I) halides. Lower oxidation state Al, Ga, In and Tl halides: Aluminum(I) halides are formed in reactions of Al(III) halides with Al at 1270 K followed by rapid cooling. Red AlCl is also formed by treating the metal with HCl at 1170 K. However, the monohalides are unstable with respect to disproportionation Gallium(I) chloride forms when GaCl3 is heated at 1370 K. It is formed in the gas phase when HCl and Ga are heated under vacuum at 1200 K, and has been characterized by IR spectroscopy as a matrix isolated species. Above 273 K, GaCl disproportionate, but solutions of GaCl in toluene and Et2O are metastable and may be used as starting materials in a similar way to solutions containing GaBr. Gallium(I) bromide can also be formed at high temperatures. A pale green, insoluble powder, ‘GaI’, can be prepared from Ga metal and I2 in toluene under ultrasonic conditions. InCl can also be isolated from the InCl3/In reaction mixture and has a deformed NaCl structure, and is practically insoluble in most organic solvents. Thallium(I) halides, TlX, are stable compounds which in some ways resemble Ag(I) halides. Thallium(I) fluoride is very soluble in water, but TlCl, TlBr and TlI are sparingly soluble. The trend in solubilities can be rationalized in terms of the increased covalent contributions in the ‘ionic’ lattices for the larger halides, a situation that parallels the trend for the Ag(I) halides. Self-study: Sulfides and oxides of Group 13 elements. Molecular species containing B–N or B–P bonds We are familiar with the formation of B-N single bonds in adducts R3N → BH3, via symmetric cleavage of B2H6 with soft Lewis bases. Molecular compounds with boron–nitrogen multiple bonds also exist in stable forms. The thermodynamically stable phase of boron nitride, BN, consists of planar sheets of atoms like those in graphite The hexagonal B3N3-motif in the layered form of boron nitride appears in a group of compounds called borazines. The parent compound (HBNH)3, is isoelectronic and isostructural with benzene Borazine is a colourless liquid (mp 215 K, bp 328 K) with an aromatic odour and physical properties that resemble those of benzene. It is generally prepared by the following reactions: The use of an alkylammonium chloride in place of NH4Cl in reaction leads to the formation of an N-alkyl derivative (ClBNR)3 which can be converted to (HBNR)3 by treatment with Na[BH4]. The BN distances in the planar B3N3 ring are equal (144 pm) and close to those in the layered form of BN. This involves delocalization of the N lone pairs around the ring as shown in one resonance form of borazine, analogous to a Kekule´ structure for benzene. Electronic structures of benzene (top) and borazine (bottom) Contour map of the charge density in the molecular plane of benzene (left) and borazine (right) Despite the formal charge distribution, a consideration of the relative electronegativities of B (χP = 2.0) and N (χP = 3.0) indicates that B is susceptible to attack by nucleophiles while N attracts electrophiles. Thus, the reactivity of borazine contrasts sharply with that of benzene, although it must be remembered that C6H6 is kinetically inert towards the addition of, for example, HCl and H2O. The products of these reactions with four coordinated B and N centers possesses a chair conformation (compare cyclohexane). Treatment of (ClHBNH2)3 with Na[BH4] leads to the formation of (H2BNH2)3 It is known that the less-reactive hexamethyl derivative B3N3Me6 (which can be heated to 460 ℃ for 3 h without significant decomposition) reacts with [Cr(CO)3(MeCN)3] to give the complex [Cr(η6-B3N3Me6)(CO)3] (Figure below) which closely resembles the corresponding hexamethylbenzene complex [Cr(η6-C6Me6)(CO)3]. The electronic differences between benzene and borazine: The bonding of Cr(CO)3 with benzene is nearly twice as strong as that for borazine. Measured from metal to ring dissociation energy. 2nd evidence is that borazine molecule is puckered in these complexes. The actual structure appears to be intermediate between a true π-complex and the extreme σ-only model. Heating borazine at 70 °C expels hydrogen with formation of a borazinyl polymer or polyborazylene, in which the monomer units are coupled in a para fashion by new boron-nitrogen bonds. Borazine and its derivatives are potential precursors to boron nitride ceramics. Boron nitride can be prepared by heating polyborazylene to 1000 °C. Dewar benzene Dewar benzene or bicyclo[2.2.0]hexa-2,5-diene is a bicyclic isomer of benzene with the molecular formula C6H6. Unlike benzene, Dewar benzene is not flat because the carbons where the rings join are bonded to four atoms (3 C and 1H). These carbons show a distorted tetrahedral geometry, and the two cyclobutene rings make an angle where they are cis-fused to each other. The compound has nevertheless considerable strain energy and reverts to benzene with a chemical half-life of two days. This thermal conversion is relatively slow because it is symmetry forbidden based on orbital symmetry arguments. Synthesized by photolysis of the cis-1,2-dihydro derivative of phthalic anhydride followed by oxidation with lead tetraacetate Hexamethyl Dewar benzene has been prepared by bicyclotrimerization of dimethylacetylene with aluminium chloride The Dewar borazine derivatives: are prepared by cyclotrimerization of iminoboranes These iminoboranes have short B-N bonds with triple bond character. Dewar borazines are prepared by cyclotrimerization of iminoboranes R-B≡N-R’. A family of R-B≡N-R’ compounds is known, and can be rendered kinetically stable with respect to oligomerization by the introduction of bulky substituents and/or maintaining low temperatures. For example, tBuB≡NtBu (with sterically demanding tert-butyl groups) has a half-life of 3 days at 323 K. The ‘open-book’ conformation of the B3N3 framework mimics that of the C6-unit in Dewar benzene. The bond distances in the central BN bond in Dewar borazine is longer than a typical B- N single bond, the four distances of 155 pm are close to those expected for single bonds, and the two remaining B-N bond lengths correspond to double bonds. N,N’,N’’-tBu3-B,B’,B’’-Ph3B3N3 Boroxine, H3B3O3 is isoelectronic with benzene. Boroxine is prepared by explosive oxidation of B2H6 or B5H9. Simple boroxine is less stable and decomposes at room temperature to diborane and boron oxide. However, substituted boroxines are stable molecules and are solid at room temperature. Boroxine is planar but has even less π-delocalization than borazine. Electronegativity values F 3.98 R Cl 3.16 B Br 2.96 O O I 2.66 B B B 2.04 R O R N 3.04 R=Me, Phetc. O 3.44 P 2.19 Boroxine derivatives such as trimethylboroxine and triphenylboroxine are solids that are usually in equilibrium with their respective boronic acids at room temperature. Boroxines are produced from their corresponding boronic acids by dehydration using a drying agent or by heating under a high vacuum. R B O O HO dehydration B R B B HO R O R R = Me, Ph etc. The synthesis of trimethylboroxine involves the reaction of carbon monoxide with B2H6 and lithium borohydride (LiBH4) as a catalyst. Boron single-bonds on boroxine compounds are mostly σ-character. Et3B3O3 boroxine has B-O bond lengths of 1.384 Å and B-C bond lengths of 1.565 Å. Phenyl-substituted boroxine shows bond lengths of 1.386 Å and 1.546 Å respectively, showing that the substituent has little effect on the boroxine ring size. Phosphorus analogue of borazine The parent compound B3P3H6 deviates slightly from planarity and exhibits some aromaticity. R t B u P t B u R P R electronegativity B B B 2.04 P P N 3.04 P P R B R O 3.44 t B u P 2.19 R R = Ph 2,4,6-tri-tert-butyl-1,3,5-triphosphabenzene. The B3P3R6 rings can form η6-complexes with transition metal carbonyl fragments example Cr(CO)3. R R P R B B P P R B R R B-P bond distance 1.84 Å The B3P3 ring is planar and has equal B-P bond lengths and shortened B-P bonds, suggesting significant aromaticity. Polyhedral borane cluster compounds Types of bonds found in complex/higher boranes: B-H terminal bond (2c2e bond) B-H-B bridge bond (3c2e bond) B-B (2c2e bond) B-B-B (3c2e bond) formed from overlap of 3-orbitals from 3-corners of an equilateral triangle of B atoms. styx Rule/ Number: The styx number is used to understand the types of bonds in boranes. W.N. Lipscomb developed a method for determining what combinations of bonding features (types of bonds) are possible for a specific formula of boron hydride. The each boron in neutral boron hydrides, hydroborate anions and carboranes cations each boron has at least one H (or,other substituent ) attached by a normal two electron sigma bond (2c-2e). It can be assumed that one B-H bond is present per boron atom. styx number s = number of B-H-B bonds t = number of 3 Center BBB bonds (2002) y = number of 2 C BB bonds B2H6 x = number of BH2 units H H H B H H B B H H B H H H B4H10 (4012) B5H9 (4120) B5H11 (3203) B6H10 (4220) (4620) Nomenclature: Boranes are named by indicating the number of B atoms with a latin prefix and the number of H atoms by an Arabic number in parentheses, e.g. B5H9, pentaborane(9); B5H11, pentaborane(11). Names for anions end in “ate” rather than “ane” and specify both the number of H and B atoms and the charge, e.g. B5H8- , octahydropentaborate(1-). Further information can be provided by the optional inclusion of the italicized descriptors closo-, nido-, arachno-, hypho- and conjuncto-, e.g.: B6H62- : hexahydro-closo-hexaborate(2-) B5H11: arachno-pentaborane(11) B4H10: arachno-tetraborane(10) B7H72-: heptahydro-closo-heptaborate(2-) B10H102- : decahydro-closo-decaborate(2-) B10H14: nido-decaborane(14) B10H142-: tetradecahydro-arachno-decaborate(2-) B10H16: 1,1’-conjuncto-decaborane(16) i.e., 1,1’-bi(nido-pentaboranyl)] Naming polyhedral boranes: Closo-, Nido-, Arachno-, Hypho-, and Conjuncto- The deltahedral cages with five to 12 vertices which are the parent cages used in conjunction with Wade’s rules to rationalize borane cluster structures. As a general (but not foolproof) scheme, when removing vertices from these cages to generate nido-frameworks, remove a vertex of connectivity three from the trigonal bipyramid, any vertex from the octahedron or icosahedron, a ‘cap’ from the tricapped trigonal prism or bicapped square-antiprism, and a vertex of highest connectivity from the remaining deltahedra. Naming polyhedral boranes: Closo-, Nido-, Arachno-, Hypho-, and Conjuncto- Depicting family of polyhedral boranes: Closo boranes are considered as parent clusters and other boranes are hypothetically derived from it via removal of B vertexes. Likely structure for [B5H8]⎯ ❖ There are five {BH}-units and three additional H atoms. ❖ Each {BH}-unit provides two valence electrons. ❖ There is one electron from the 1 –ve charge. ❖ Total number of cage-bonding electrons available ❖ = (5 x 2) + 3 + 1 = 14 electrons =7 pairs ❖ Seven pairs of electrons are consistent with the parent deltahedron having six vertices, i.e. (n + 1) = 7, and so n = 6. ❖ The parent deltahedron is an octahedron and the B5-core of [B5H8]⎯ will be derived from an octahedron with one vertex left vacant: The three extra H atoms form B-H-B bridges along three of the four B-B edges of the open (square) face of the B5- cage. The predicted structure of [B5H8]⎯ is: Polyhedral electron pair theory; Wade’s Rules or Wade / Mingo’s rule: A systematic classification scheme for polyhedral brane clusters These classification rules are based on a set of rules formulated by Prof. Kenneth Wade in 1971. Classification of structural types are often done based on the valence electron counts. The formulation helps to rationalize bonding and topology in borane clusters They were originally formulated by K. Wade and further developed by Mingos and others, and are sometimes known as Wade's Rules or Wade / Mingos rules. Polyhedral skeletal electron pair theory The polyhedral skeletal electron pair theory provides electron counting rules used to predict the structure of electron deficient clusters. The rules were originally developed to aid the prediction of the structures of borane and carborane cluster compounds and are based on a molecular orbital treatment of the bonding. The rules only apply to clusters that have the same deltahedral geometry as the boranes and carboranes. That is to say their structures must be based on polyhedra where every face is triangular. The deltahedral boranes and carboranes are classified as closo-, nido-, arachno- or hypho-, based on whether they represent a complete (closo-) Deltahedron, or a Deltahedron that is missing one (nido-), two (arachno-) or three (hypho) vertices. Classification and electron count of boron hydrides Summary of Wade’s rule Closo ---> clovos Nido ---> nidus Arachno ---> Spider’s web Hypho ---> hyphe; a net Conjucto ---> conjuncto; I join together The rules for boranes and carboranes The skeletal orbital (electron pair) and electron counts for the four types of deltahedral clusters are:- n vertex closo (n+1) orbitals; (2n+2)electrons n vertex nido (n+2) orbitals; (2n+4)electrons n vertex arachno (n+3) orbitals; (2n+6)electrons n vertex hypho (n+4) orbitals; (2n+8)electrons The skeletal electron counts are determined by totaling the following:- 2 from each BH unit 3 from each CH unit 1 from each additional H atom (over and above the ones on the BH and CH units) the anionic charge electrons Example of B6H62− with 6 BH units and ionic charge of 2− Framework electron count: 2x6+2 (12 + 2) = 14 As the number of vertices is 6 the prediction is closo (14 = 2(6+1)) Example of B10H14 with 10 BH units Framework electron count: 2x10+4 = 24 As the number of vertices is 10 the prediction is nido (24 = 2(10+2)) Example of B10H142- with 10 BH units and anionic charge of 2- Framework electron count: 2x10+4+2 = 26 As the number of vertices is 10 the prediction is arachno (26 = 2(10+3)) Example of C2B7H13 with 7 BH units, 2CH units and 4 additional H Framework electron count: 14 + 6 + 4 = 24 As the number of vertices is 9 the prediction is arachno (24 = 2(9+3)) Heteroboranes: boranes containing vertices with one or more atoms other than B {BH} Contributes 2e to the cluster {CH} Contributes 3e to the cluster {NH} or {PH} Contributes 4e to the cluster {SH} Contributes 5e to the cluster {S} Contributes 4e to the cluster plus a lone pair as exo- polyhedral to the cluster Total electron count (TEC) formulation for boranes and carboranes The more straightforward version of the rules that uses a total valence electron count. For boranes and carboranes the total valence electrons are counted plus any charge electrons, the rules are simply: ❖ n vertex closo (4n+2) electrons ❖ n vertex nido (4n+4) electrons Polyhedral electron count (PEC) ❑ n vertex closo (n+1) orbitals; (2n+2)electrons ❖ n vertex arachno (4n+6) electrons ❑ n vertex nido (n+2) orbitals; (2n+4)electrons ❑ n vertex arachno (n+3) orbitals; (2n+6)electrons ❖ n vertex hypho (4n+8) electrons ❑ n vertex hypho (n+4) orbitals; (2n+8)electrons Theoretical basis for the rules for boranes A brief insight into the theory behind the rules through a consideration of the anionic closo cluster B6H62−. The boron atoms sit at the vertices of an octahedron, each using one sp hybrid, that points radially out of the polyhedron, to form a σ-bond to a hydrogen atom. Each boron atom therefore has 3 orbitals remaining to contribute to framework molecular orbitals i.e.: 1 sp hybrid orbital pointing radially into the center of the cluster 2 p orbitals tangential to the surface 18 framework molecular orbitals, (MOs) derived from the 18 boron atomic orbitals are: 1 bonding MO at the center of the cluster and 5 antibonding MOs from the 6 sp radial hybrid orbitals 6 bonding MOs and 6 antibonding MOs from the 12 tangential p orbitals. The total skeletal bonding orbitals is therefore 7 i.e., (n+1). Bonding in [B6H6]2– Closo-boranes BnHn2– where (n = 6-12) The neutral boranes BnHn+2 are not known Nido-boranes (1) BnHn+4 e.g., B2H6, B5H9, B6H10, B10H14 B2H6 B6H10 B5H9 B8H12 B10H14 B8H12 can be visualized as being formed from B10H14 by removal of B(9) and B(10) Nido-boranes (2) BnHn+3– formed by the removal of 1 bridged proton from BnHn+4 e.g., B5H8–, B10H13–. Nido-boranes (3) BnHn+22– e.g., B10H122–, B11H132– Arachno-boranes: (1) BnHn+6 e.g., B4H10, B5H11, B6H12, B8H14, B9H15 (2) BnHn+5– e.g., B2H7–, B3H8–, B9H14- (3) BnHn+42– e.g., B10H142– B5H11 B6H12 B9H15 [B3H8]- [B2H7 ]- B4H10 [B9H14]- [B10H14]2- Hypho-boranes: BnHn+8 No neutral borane has been definitely established in this series but the known compounds B8H16, and B10H18 may prove to be hypho-boranes and several adducts are known to have hypho-structures. Hetero-boranes: Inclusion of Other Atoms in the Hydroborane Cage Many derivatives of boranes containing other main group atoms are also known. These heteroboranes may be classified by formally converting the heteroatom to a BHx group having the same number of valence electrons. Carboranes are also known in closo-, nido-, arachno- and hypho- structures and conform to the Wade’s rule Examples of monocarboranes CB5H7 and CB5H9 Considering Other Atoms in the Context of Wade’s Rules: Classification of Heteroborane Clusters These represent the most common main group heteroatoms incorporated into hydroborane clusters. Nido-B6H10 and examples of structurally related isoelectonic carboranes Nido-B6H10 provides the basic cluster structure for the four carboranes: CB5H9, C2B4H8, C3B3H7, C4B2H6, each successive replacement of a basal B atom by C being compensated by the removal of one Hµ Pyrolysis of alkyl boranes at 500-600 C is a related route which is particularly useful to monocarbaboranes though the yields are often low, e.g.: Some generalizations concerning carboranes Although closo-carboranes are stable to high temperatures and to most common reagents, M. F. Hawthorne showed (1964) that they can be specifically degraded to nido-carborane anions by the reaction of strong bases in the presence of protonic solvents, e.g.: Figure 6.20 indicates that, in both cases, the BH vertex removed is the one adjacent to the two CH vertices: since the C atoms tend to remove electronic charge preferentially from contiguous B atoms, the reaction can be described as a nucleophilic attack by EtO- on the most positive (most electron deficient) B atom in the cluster. Deprotonation of the monoanions by NaH removes the bridge proton to give the nido-dianions 7,8-C2B9H112- (80) and 7,9-C2B9H112- (81). Metallocarboranes: Deprotonation of the monoanions by NaH removes the bridge proton to give the nido-dianions 7,8- C2B9H112– (80) and 7,9-C2B9H112– (81). It was the perceptive recognition that the open pentagonal faces of these dianions were structurally and electronically equivalent to the pentahapto cyclopentadienide anion (η5-C5H5)– (Fig. below) that led to the discovery of the metallocarboranes and the development of some of the most intriguing and far-reaching reactions of the carboranes. Metallocarboranes: There are now at least a dozen synthetic routes to metallocarboranes including: (i) coordination using nido-carborane anions as ligands, (ii) Polyhedral expansion reactions, (iii) polyhedral contraction reactions, (iv) polyhedral subrogation and (v) thermal metal transfer reactions. These first five routes were all devised by M. F. Hawthorne and his group in the period 1965-74 and have since been extensively exploited and extended by several groups. (i) (ii) (iii) (iv) (v) (a) Dicarbollyl species; M = Fe, n = 2 and M = Co, n = 1 are isoelectronic with ferrocene and cobaltocenium ion; M = Fe, n = 1 is isoelectronic with ferrocenium ion (b) Mixed carbollyl-cyclopentadienyl analogue of ferrocene, n = 1 (c) Mixed carbollyl-carbonyl compound, M = Mn, Re. The number of vertices in the n vertex closo (n+1) orbitals; (2n+2)electrons deltahedron will be one less than the n vertex nido (n+2) orbitals; (2n+4)electrons number of bonding pairs in the n vertex arachno (n+3) orbitals; (2n+6)electrons framework. n vertex hypho (n+4) orbitals; (2n+8)electrons