CHM 102 (Inorganic Chemistry) 2019-2020 Notes PDF
Document Details
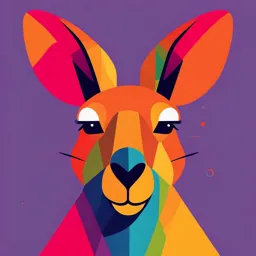
Uploaded by DefeatedKyanite
Olabisi Onabanjo University
2019
Dr. Segun A. Ogundare
Tags
Summary
These lecture notes cover inorganic chemistry topics for a CHM 102 course at Olabisi Onabanjo University. They discuss bonding and intermolecular forces, periodic trends, and the chemistry of main group elements. Questions are included at the end of each topic section.
Full Transcript
OLABISI ONABANJO UNIVERSITY FACULTY OF SCIENCE CHEMICAL SCIENCES DEPARTMENT CHM 102: GENERAL CHEMISTRY II (INORGANIC) DR. SEGUN A. OGUNDARE Content: Bonding and Intermolecular Forces The Periodic Table and Periodicity Descriptive Inorganic chemistry of Main gr...
OLABISI ONABANJO UNIVERSITY FACULTY OF SCIENCE CHEMICAL SCIENCES DEPARTMENT CHM 102: GENERAL CHEMISTRY II (INORGANIC) DR. SEGUN A. OGUNDARE Content: Bonding and Intermolecular Forces The Periodic Table and Periodicity Descriptive Inorganic chemistry of Main groups Brief introduction to transition metal Chemistry Nuclear Chemistry Bonding and Intermolecular Forces Chemical Bonding Chemical bonding is an interaction between atoms of the same or different elements with the purpose of attaining stable electronic configuration. The electronic configuration is the arrangement or distribution of electrons in the atomic orbitals. A stable electronic configuration is attained if the shells are filled with electrons. The outermost or the valence shell should have 8 electrons with the exception of the first period elements, which are H and He. Bonding and Intermolecular Forces (contd.) Chemical Bonding According to G. N. Lewis an atom is most stable if its outermost shell is either filled or contains eight electrons. An atom will lose, gain, or share electron(s) in order to achieve a stable electronic configuration of the nearest noble gas. The tendency of an atom to attain an electronic configuration, such that its valence shell contains 8 electrons is known as the octet rule. Bonding and Intermolecular Forces (contd.) Types of Chemical Bonds: The ionic (electrovalent) and the covalent bonds. The Ionic (electrovalent) Bond This is a description of a chemical bond that involves loss or gain of electron(s) by atoms. The atom that loses electron(s) will have a decrease in its number of electrons. the atom that accepts the lost electron(s) will have an increase in number of its electrons. The atom that lost electron(s) transforms to positive (cation) ion The atom that gained electron(s) transforms to negative (anion) ion. Bonding and Intermolecular Forces (contd.) The Covalent Bond (Ordinary and Dative) This is a description of a chemical bond that involves sharing of pair(s) of electrons. An Ordinary covalent bond is formed when the shared pair is equally donated by the atoms. A Dative or coordinate covalent bond is formed when the shared pair is donated by one of the atoms. Bonding and Intermolecular Forces (contd.) Other types of bonds: Metallic bond: It exists between metallic atoms of the same element or alloys. Metal valence electrons are not strongly attracted to the nucleus. The electrons move from one atom to another linking up to form conducting band on the metal surface. The bond exists in solid metals like aluminum, iron and copper. The higher the valence electrons the greater the metallic bond. Bonding and Intermolecular Forces (contd.) Other types of bonds; Intermolecular bonds which include are; Hydrogen bond: It exists between molecules containing H bonded to F, O or N. Dipole-Dipole force: It exists between heteronuclear molecules that are polar such as: H2S, CO and SO2. London dispersion force: It exists between homo- and heteronuclear molecules which are non-polar. I2, CCl4, H2. Bonding and Intermolecular Forces (contd.) Non polar Hydrides of group IVA: Effect of Hydrogen bonding in group V-VIIA London forces effect on boiling point. London forces Bonding and Intermolecular Forces (Questions) Which of the following contain(s) both ionic and covalent bonds? NH3, NH4Cl, MgCl2, CO2, Mg, F2, Which of the following contain(s) only ordinary covalent bond? NH3, NH4Cl, MgCl2, CO2, Mg, F2 Which of the following contain(s) both Dative and ordinary covalent bonds? NH3, NH4Cl, MgCl2, CO2, Mg, F2 Which of the following contain(s) ionic bond? NH3, NH4Cl, MgCl2, CO2, Mg, F2 Which of the following will have hydrogen bond? NH3, NH4Cl, MgCl2, CO2, Mg, F2 Which of the following will have metallic bond? NH3, NH4Cl, MgCl2, CO2, Mg, F2 Which of the following will have London forces? NH3, NH4Cl, MgCl2, CO2, Mg, F2 Which of the following will have dipole-dipole forces bond? NH3, NH4Cl, MgCl2, CO2, Mg, F2 The Periodic Table and Periodicity The Periodic Table: This consists elements arranged in order of atomic number into 18 groups and 7 periods. The groups are divided into main (representative) groups, transition groups The main groups are labelled IA-VIIIA while the transition groups are labelled IB-VIIIB. The elements are distributed into different blocks. The block of any element is determined by the location of its last valence electron when at ground state. The transition elements are located in d-block while the inner transition are located in f-block The Periodic Table and Periodicity (contd.) Periodicity: This is the recurrent variation in properties of elements, when arranged in order of atomic number. Physical properties such as atomic radius, first ionization energy, electron affinity, ionic radius and electronegativity show variation within the groups and across the periods. Atomic radii of elements increase down the groups due to additional shell and decrease across the period as the valence shell shrinks with increase in nuclear charge. Atomic radius theoretically is the average distance between the center of the nucleus and the valence shell. The Periodic Table and Periodicity (contd.) The Ionic radii of cations (positively charged ions) are smaller than the corresponding atomic radii. The ionic radii of anions (negatively charged ions) are smaller than the corresponding atomic radii. Examples: Na+ (116 pm)<Na (154 pm) Mg2+ (86 pm)<Mg (130 pm), O2- (126 pm) >O (73 pm) F- (119 pm) >F (71 pm) The Periodic Table and Periodicity (contd.) First ionization energy is the minimum amount of energy required to remove the most loosely bound electron from the valence shell of one mole of isolated gaseous atoms to form ions with a unit positive charge. Be (1s2 2s2) → Be+ (1s2 2s1) + e- First ionization energy Be+ (1s2 2s1) → Be2+ (1s2) + e- Second ionization energy, Ionization energy decreases down the group. but increases across the period. The Periodic Table and Periodicity (contd.) The electron affinity is the energy change that occurs when an electron is added to a neutral atom in the gaseous state to form a negative ion. Cl + e- → Cl- It increases down the group but decreases across the period, though not in a regular pattern. The negative energy implies energy is released when electron is added. The higher the energy released the greater the stability of the anion formed. The Periodic Table and Periodicity (contd.) Electronegativity is a measure of the relative ability of an atom in a molecule to attract the bonding electrons (shared pair) to itself. Electronegativity increases across the period and decreases down the group. Pauling electronegativity is based on bond energies, using the empirical observation that bonds between atoms with a large electronegativity difference tend to be stronger than those where the difference is small. The Periodic Table and Periodicity (contd.) The Periodic Table and Periodicity (Questions) 1. Which of the following has the lowest first ionization energy (a) Ca (b) Mg (c) Al (d) O 2. Which of the following has the largest ionic radius (a) Ca2+ (b) S2- (c) Al3+ (d) Mg2+ 3. Which of the following has the smallest ionic radius (a) Ca2+ (b) S2- (c) Al3+ (d) Mg2+ State the reason for the decrease in ionization energy of O with respect to N. Descriptive Inorganic chemistry of Main groups The chemical and physical properties of the elements in the main groups show periodic trends. The elements in groups IA, IIA and IIIA are metallic while group IVA contains elements with nonmetallic, metalloid and metallic characters. Moving farther left to groups V-VIIA , elements show strong nonmetallic character. The variants of metallic–nonmetallic character are related to variations in the ionization energies the corresponding atoms. Those with low ionization energy have strong metallic characteristics, those with high ionization energy show stronger tendency for nonmetallic character. Descriptive Inorganic chemistry of Main groups (contd.) Group IA elements are also known as the alkali metals. They are very reactive and soft. They exist as compounds such as chloride, silicate, carbonate and nitrate. Elements in the group are: 3Li ([He] 2s1), 11Na ([Ne] 3s1), 19K ([Ar] 4s1), 37Rb ([Kr] 5s1), 55Cs ([Xe] 6s1) and 87Fr ([Rn] 7s1). Sources: The elements occur in nature as salts, examples: Lithium (Li) is found Spodumene (LiAl(SiO3)2), sodium (Na) is found in Rock salt (NaCl), Saltpetre (NaNO3), Borax (Na2B4O7.10H2O) and Trona (Na2CO3.NaHCO3.2H2O). Potassium (K) is found in Sylvite (KCl), Sylvinite (KCl/NaCl) and Carnalite (KCl.MgCl2.6H2O). Rubidium (Rb) and Caesium (Cs) have no known suitable natural sources of commercial value. Francium is radioactive in nature Descriptive Inorganic chemistry of Main groups (contd.) Extraction: Lithium and sodium are obtained by electrolysis of their fused halide salts. 2LiAl(SiO3)2 + 4CaO → Li2O + Al2O3 + 4CaSiO3 Li2O + H2O → 2LiOH LiOH + HCl → LiCl + H2O For the extraction of sodium, the electrolyte is a mixture of 40% sodium chloride and 60% calcium chloride (as impurity) in a Down cell. 2Cl-(l) → Cl2(g) + 2e- Na+ + e- → Na(l) Potassium is obtained by reduction of KCl with sodium vapour. Rubidium and Caesium are produced in a similar manner using calcium under reduced pressure. Fr is obtained by radioactivity KCl + Na → NaCl + K 2RbCl + Ca → CaCl2 + 2Rb 227 223 2CsCl + Ca → CaCl2 + 2Cs 89𝐴𝑐 → 87𝐹𝑟 + 42𝐻𝑒. Descriptive Inorganic chemistry of Main groups (contd.) Physical Properties of Group IA Metals They are silvery-white in appearance except caesium, which has a golden- yellow appearance. The atomic and ionic radii of elements in this group increases down the group. All the metals in the group are soft with lithium being the hardest. The metals have very low density They have the lowest first ionization energy and electronegativity The heat of hydration (ΔHhydr) varies with size. It is the heat released following infinite dilution of mole of a gaseous ion. Descriptive Inorganic chemistry of Main groups (contd.) Chemical Properties of Group IA Metals Reaction with water: All the metals in the group react with H2O. 2M(s) + 2H2O(l) → 2MOH(aq) + H2(g), M: Li, Na, K, Rb, Cs Reaction with air: The metals in this group tarnish in air due to their high reactivity. 4Li + O2 → 2Li2O (monoxide) 2Li + O2 → Li2O2 (very small peroxide) 6Li + N2 → 2Li3N (nitride) 2Li3N → 6Li + N2 (decomposition) Li3N + 3H2O → 3LiOH + NH3 (reaction with H2O) Sodium forms higher proportion of peroxide than monoxide in air. K forms superoxide in addition to peroxide Monoxide: 4Na + O2 → 2Na2O (oxidation state of O is -2) 2Na2O2 → 2Na2O + O2 2KNO3 + 10K → 6K2O + N2 4KO2 → 2K2O + 3O2 2KNO3 + 10K → 6K2O + N2 Peroxide: 2Na + O2 → Na2O2 (oxidation state of O is -1) Superoxide: K + O2 → KO2 (oxidation state of O is -1/2) Descriptive Inorganic chemistry of Main groups (contd.) Chemical Properties of Group IA Metals The hydroxides have very strong basic character with high solubility in H2O with exception of LiOH, which is sparingly soluble. NaOH + HCl → NaCl + H2O (reaction with acid) 2NaOH + CO2 → Na2CO3 + H2O (reaction with acid anhydride) NaOH + NH4Cl → NaCl + NH3 + H2O (reaction with NH4Cl) 2NaOH + H2S → Na2S + 2H2O (reaction with H2S) The peroxides and superoxides also react with other compounds and elements. 3Na2O2 + 4Al → 2Al2O3 + 6Na (violent reaction) Na2O2 + CO → Na2CO3 2Na2O2 + 2CO2 → 2Na2CO3 + O2 (This is used to produce O2 in submarine) 4KO2 + CO2 → 2K2CO3 + 3O2 4KO2 + 4CO2 + 2H2O → 4KHCO3 + 3O2 (This is used to produce O2 in breathing apparatus) Descriptive Inorganic chemistry of Main groups (contd.) Compounds of Group IA Metals Carbonates and bicarbonates are formed by reaction of the metal hydroxides with CO2, when CO2 is in excess, bicarbonates are formed. 2NaOH + CO2 → Na2CO3 + H2O Na2CO3 + H2O + CO2 → 2NaHCO3 Water hardness treatment: Na2CO3 + CaSO4 (soluble) → Na2SO4 + CaCO3 (insoluble) Uses of NaHCO3 : It is used as source of CO2 in; baking powder and fire extinguisher. NaHCO3 also has medicinal value as antacid for treatment of indigestion, heartburn and stomach upset. Large fraction of sodium carbonate is still produced by the Solvay process. The Solvay process is an industrial method for obtaining sodium carbonate from sodium chloride and limestone. CaCO3(s) → CaO(s) + CO2 (g) (generation of CO2) NH3(g) + H2O(l) + CO2(g) + NaCl(aq) → NaHCO3 (s) + NH4Cl(aq) (formation of bicarbonate) 2NaHCO3 (s) → Na2CO3 (s) + CO2(g) + H2O(l) (Decomposition to obtain carbonate) Na2CO3 (s) + 10H2O(l) → Na2CO3.10H2O(s) (hydration of carbonate) CaO(s) + H2O(l) → Ca(OH)2(aq) (slaking of lime (CaO)) Ca(OH)2(aq) + 2NH4Cl(aq) → CaCl2(aq) + 2NH3(g) + 2H2O(l) (Recovery of NH3) Descriptive Inorganic chemistry of Main groups (contd.) Compounds of Group IA Metals Nitrates (NO3-) and Nitrites (NO2-): The nitrates are formed by reaction of the group IA metal hydroxides or carbonates with nitric acid. The nitrites are obtained by thermal decomposition of the nitrates or by reacting metal hydroxide with NO. NaOH + HNO3 → NaNO3 + H2O (NaNO3 is used as nitrogenous fertilizer in Chile) K2CO3 + 2HNO3 → 2KNO3 + H2O + CO2 (KNO3 is used as gun powder) 2NaNO3 → 2NaNO2 + O2 (thermal decomposition at 500°C) 4NaNO3 → 2Na2O + 5O2 + 2N2 (thermal decomposition at 800°C) 2NaNO3 + C → 2NaNO2 + CO2 (reduction) KNO3 + Zn → KNO2 + ZnO (reduction) 2KOH + 4NO → 2KNO2 + N2O + H2O (reaction with NO) 2KOH + 6NO → 4KNO2 + N2 + 2H2O (reaction with NO) Hydrides (H-): Group IA metals react with H2 gas to give hydrides 2M + H2 → 2MH MH + H2O → MOH + H2 (LiH + H2O is used for supply of H2) Lithium and sodium are known to form complex hydrides, which are strong reducing agents: 4LiH + AlCl3 → LiAlH4 + 3LiCl (formation of lithium aluminum tetrahydride) 4NaH + B(OCH3)3 → NaBH4 + 3NaOCH3 (formation of sodium boron tetrahydride) Descriptive Inorganic chemistry of Main groups (contd.) Compounds of Group IA Metals Amides (NH2-): Amides of group IA metals are formed by reaction of the metals with ammonia in the presence of Fe catalyst. 2M + 2NH3 → 2MNH2 + H2 Carbides (C22-): Lithium reacts directly with carbon to form carbide but others form carbides by reacting with ethyne (C2H2). 2Li + 2C → Li2C2 2Na + C2H2 → Na2C2 + H2 Sulphates (SO42-) and bisulphates (HSO4-): sulphates are formed by complete neutralization of sulphuric acid with corresponding metal hydroxides, while bisulphates are formed by partial neutralization. 2NaOH + H2SO4 → Na2SO4 + 2H2O (complete neutralization) NaOH + H2SO4 → NaHSO4 + H2O (partial neutralization) 2NaHSO4 → Na2S2O7 + H2O (thermal decomposition) Na2S2O7 → Na2SO4 + SO3 (at higher temperature) Organic salts: 2CH3COOH + 2Na → 2CH3COO-Na+ + H2 Descriptive Inorganic chemistry of Main groups (contd.) Diagonal relationship of lithium with Magnesium: Lithium exhibits anomalous behaviour comparing with other group IA metal. The properties of lithium resemble that of magnesium more than that of other group IA metals. This is an example of diagonal relationship. The anomaly is mainly due to the exceptional high charge density and polarizing power of lithium ion comparing with other group IA metal ions. Properties Lithium Magnesium Sodium Burning in air Formation of normal Formation of normal Formation of peroxide on oxide oxide top of normal oxide Heating of carbonate Decompose into oxide Decompose into oxide Stable to heat and carbon dioxide and carbon dioxide Heating of hydroxide Decompose into oxide Decompose into oxide Stable to heat and water and water Heating of nitrate(V) Decompose into oxide, Decompose into oxide, Decompose into nitrogen dioxide and nitrogen dioxide and nitrate(III) and oxygen oxygen oxygen only Formation of Do not form stable solid Do not form stable solid Form stable solid hydrogencarbonate hydrogencarbonate hydrogencarbonate hydrogencarbonate Formation of Form organometallic Form organometallic Do not form any stable organometallic compound e.g. RLi compound e.g. MgR2 organometallic compound compound at all Solubility of salts Insoluble phosphate, Insoluble phosphate, Soluble phosphate, fluoride and carbonate fluoride and carbonate fluoride and carbonate Stability of compound Form stable nitride Form stable nitride Do not form stable nitride, Descriptive Inorganic chemistry of Main groups (contd.) Group IIA metals are also known as the alkaline earth metals. They are very reactive but less reactive than the group IA metals. Elements in the group are: 4Be ([He] 2s2), 12Mg ([Ne] 3s2), 20Ca ([Ar] 4s2), 38Sr ([Kr] 5s2), 56Ba ([Xe] 6s2) and 88Ra ([Rn] 7s2). Sources: The elements occur in nature as salts, examples: Beryllium: beryl (Be3Al2Si6O18) and phenacite (Be2SiO4) Magnesium: dolomite (MgCO3.CaCO3), Magnesite (MgCO3) and talc (Mg3(OH)2Si4O10) Calcium is found in limestone (CaCO3) and fluorite (CaF2) Strontium is found in strontianite (SrCO3) and celestite (SrSO4) Barium is found in barite BaSO4; Radium is radioactive in nature, it is found in small quantity (1g/tonne) in uranium mineral called pitchblende (UO2). beryl limestone dolomite Descriptive Inorganic chemistry of Main groups (contd.) Extraction: Beryllium is extracted at 350°C by electrolysis of beryllium chloride (BeCl2), which is produced from beryl. It can also be obtained by reduction of beryllium fluoride (BeF2) using magnesium. BeF2 + Mg → Be + MgF2. Magnesium can be extracted by several methods; the dominant process is the electrolysis of a fused halide mixture of MgCl2 + CaCl2 + NaCl. From Sea water rich in Mg2+ and Ca2+ ions: Ca(OH)2(aq) + Mg2+(aq) → Mg(OH)2(s) + Ca2+(aq) (Precipitation) Mg(OH)2 + 2HCl → MgCl2 + 2H2O (Neutralization) Electrolysis Mg2+ + 2e- → Mg (at the iron cathode) 2Cl- → Cl2 + 2e- (at the graphite anode) Reduction MgO + C → Mg +CO (at 200°C) CaCO3.MgCO3 → CaO.MgO + 2CO2 (decomposition) 2CaO.MgO + Si → 2Mg + Ca2SiO4 Calcium, strontium and barium are extracted by electrolysis of their respective halides Descriptive Inorganic chemistry of Main groups (contd.) Physical Properties of Group IIA Metals They are mostly silvery in appearance. The atomic and ionic radii of metals in this group are large but smaller than those of corresponding group IA metal. They are harder than those in group IA. The metals have higher density relative to Group IA They have higher first ionization energy relative to Group IA Descriptive Inorganic chemistry of Main groups (contd.) Chemical Properties of Group IIA Metals Reaction with water: Beryllium does not react with water but other metals in the group do. M + 2H2O → M(OH)2 + H2 (M: Mg, Ca, Sr and Ba) Mg + H2O → MgO + H2 Reaction with air: The metals in this group burn in air to produce a mixture of oxide and nitrides. 2Mg + O2 → 2MgO 3Mg + N2 → Mg3N2 2Be + O2 → 2BeO CaCO3 → CaO + CO2 All the oxides except beryllium oxide react with water to give hydroxides. Beryllium oxide is insoluble in water. Sodium forms higher proportion of peroxide than monoxide in air. K forms superoxide in addition to peroxide. MO + H2O → M(OH)2 (M: Mg, Ca, Sr, Ba) Beryllium oxide is amphoteric but other group IIA oxides are basic. CaO + 2HCl → CaCl2 H2O BeO + 2HCl → BeCl2 +H2O (BeO as an acid) BeO + NaOH + H2O → NaBe(OH)3 (BeO as a base) Descriptive Inorganic chemistry of Main groups (contd.) Compounds of Group IIA Metals Peroxides: BeO2 is unknown MgCO3 + H2O2 → MgO2 + H2O + CO2 Ca(OH)2 + H2O2 + 6H2O → CaO2.8H2O CaO2.8H2O → CaO2 + 8H2O (Dehydration) CaO2 + 2HCl → CaCl2 + H2O2 Sulphides: 8M + S8 → 8MS Carbonates and bicarbonates: Ca(NO3)2(aq) + Na2CO3(aq) → CaCO3(s) + 2NaNO3(aq) Mg(NO3)2(aq) + 2NaHCO3(aq) → MgCO3(s) + CO2(g) + H2O(l) + 2NaNO3(aq) CaCO3 + H2O + CO2 → Ca(HCO3)2 Sulphates: CaSO4.2H2O → CaSO4.1/2H2O (at 150°C) (Plaster of Paris) MSO4 → MO + SO3 MSO4 + 4C → MS + 4CO Nitrates: MgCO3 + 2HNO3 → Mg(NO3)2 + CO2 + H2O Hydrides: M + H2 → MH2 (M: Mg, Ca, Sr, Ba) BeCl2 + 2LiBH4 → BeB2H8 + 2LiCl BeB2H8 + 2PPh3 → BeH2 + 2Ph3PBH3 Descriptive Inorganic chemistry of Main groups (contd.) Compounds of Group IIA Metals Halides: M + Cl2 → MCl2 (M: Be, Mg, Ca, Sr, Ba) MCO3 + HCl → MCl2 + H2O + CO2 (M: Be, Mg, Ca, Sr, Ba) 2BeO + CCl4 → 2BeCl2 + CO2 (at 800°C); BeCl2 + 4H2O → [Be(H2O)4]Cl2; [Be(H2O)4]Cl2 → Be(OH)2 + 2HCl Nitrides: 3M + N2 → M3N2 Ca3N2 → 3Ca + N2 (thermal decomposition) Ca3N2 + 6H2O → 3Ca(OH)2 + 2NH3 (hydrolysis) Carbides: 2BeO + 2C → Be2C + CO2 CaO + 3C → CaC2 + CO (at 2000°C) Be + C2H2 → BeC2 + H2 Ca + 2C → CaC2 (at 1100°C) Be2C + 4H2O → CH4 + 2Be(OH)2 CaC2 + 2H2O → Ca(OH)2 + C2H2 (used for welding) BeC2 + H2O → BeO + C2H2 CaC2 + N2 → C + CaNCN (calcium cyanamide) CaNCN + H2O → CaCO3 + 2NH4OH (ammonium hydroxide) CaNCN + H2SO4 → CaSO4 + H2NCN (hydrogen cyanamide) H2NCN + H2O → CO(NH2)2 (urea) H2NCN + H2S → CS(NH2)2 (thiourea) Organic compounds of group IIA metals RBr + Mg → RMgBr (R: alkyl or aryl) Descriptive Inorganic chemistry of Main groups (contd.) Group IIIA elements occupy the first p-block of the Periodic Table because their last valence electron is located in p-orbital (ns2 np1). Elements in the group are: 5B ([He] 2s2 2p1), 13Al ([Ne] 3s2 3p1), 31Ga ([Ar] 4s2 3d10 4p1), 49In ([Kr] 5s2 4d10 5p1) and 81Tl ([Xe] 6s2 4f14 5d10 6p1). Sources: Boron: borax (Na2[B4O5(OH)4].8H2O) and kernite (Na2[B4O5(OH)4].2H2O) Aluminum is found in bauxite (Al2O3.xH2O) and cryolite (Na3[AlF6]) Gallium, Indium and Thallium exist as sulphides in nature. Al2O3 Al2O3 Al2SiO4(F, OH)2 Descriptive Inorganic chemistry of Main groups (contd.) Extraction: Boron is extracted from borax in amorphous form. Na2[B4O5(OH)4].8H2O + H2SO4 → Na2SO4 + B(OH)3 + 5H2O (precipitation of boric acid) 2B(OH)3 → B2O3 + 3H2O (dehydration to obtain boron oxide) B2O3 + 3Mg → 3MgO + 2B (reduction of boron oxide to obtain amorphous boron) 2BI3 → 3I2 + 2B (thermal decomposition over red hot tungsten to obtain crystalline boron) 2BCl3 + 3H2 → 6HCl + 2B (reduction over red hot tungsten to obtain crystalline boron) B2H6 → 3H2 + 2B (thermal decomposition to obtain crystalline boron) Aluminum is extracted from bauxite: Al2O3 (from roasted bauxite) + NaOH + 3H2O → 2NaAl(OH)4 (digestion) NaAl(OH)4 → Al(OH)3 + NaOH (crystallization aided with Al(OH)3) Al(OH)3 → Al2O3 + 3H2O (thermal dehydration) Al(OH)3 + 3NaOH + 6HF → Na3[AlF6] + 6H2O (formation of cryolite) Al3+ + 3e- → Al (reduction reaction at the cathode) 2O2- → O2 + 4e- (oxidation reaction at the anode) 2F- → F2 + 2e- (trace oxidation reaction at the anode as the concentration of F- increases) Descriptive Inorganic chemistry of Main groups (contd.) Physical Properties of Group IIIA Metals The metals are silvery-white in appearance except boron High accumulated 1st, 2nd and 3rd ionization energies give room for covalency, They are harder than those in group IIA. The tendency for the formation of +3 oxidation state decrease down the group while the tendency for +1 oxidation state increases down the group They have irregular melting points with boron having the highest melting and boiling points. Gallium has the lowest melting point. Descriptive Inorganic chemistry of Main groups (contd.) Chemical Properties of Group IIIA Metals Reaction with air: 4M + 3O2 → 2M2O3 (M: B, Al, Ga, In, Tl) ; 4Tl + O2 → 2Tl2O; 2M + N2 → 2MN (M: B, Al) Reaction with water: B and Al (no react with water). B reacts with steam, when it is red hot to form boric acid and hydrogen but Al will not react with steam. 2B + 6H2O → B(OH)3 + 3H2 Reaction with acids: Al, Ga, In and Tl react with cold diluted HCl slowly. Boron does not react with HCl. 2Al + 6HCl → 2AlCl3 + 3H2 2In + 4HCl → In[InCl4] + 2H2 2Al + 6H2SO4 → Al2(SO4)3 + 3H2 (diluted sulphuric acid) 2Al + 6H2SO4 → Al2(SO4)3 + 6H2O + 3SO2 (concentrated sulphuric acid) 2B + 3H2SO4 → 2B(OH)3 + 3SO2 (concentrated sulphuric acid) 2B + 6HNO3 → 2B(OH)3 + 6NO2 Reaction with Alkalis: 2B + 6NaOH → 2Na3BO3 + 3H2 (fused NaOH) 2Al + 2NaOH + 6H2O → 2NaAl(OH)4 + 3H2 (aq. NaOH) Reaction with ammonia: 2B + 2NH3 → 2BN + 3H2 2Al + 6NH3 → 2Al(NH2)3 + 3H2 Reaction with halogens: 2M + 3X2 → 2MX3 ; 2Tl + X2 → 2TlX (X: F, Cl, Br, I) TlI + I2 → Tl+I3- Descriptive Inorganic chemistry of Main groups (contd.) Group IVA elements show transition from nonmetallic character to metallic character down the group Elements in the group are: 6C ([He] 2s2 2p2), 14Si ([Ne] 3s2 3p2), 32Ge ([Ar] 4s2 3d10 4p2), 50Sn ([Kr] 5s2 4d10 5p2) and 82Pb ([Xe] 6s2 4f14 5d10 6p2). Sources: Carbon: graphite and diamond; silicon: sand and quartz (SiO2); germanium: trace in minerals of other elements; Tin: cassiterite (SnO2); lead: galena (PbS), anglesite (PbSO4) and cerrusite (PbCO3). Lead battery Tin candle stand Tin cans Descriptive Inorganic chemistry of Main groups (contd.) Extraction: Carbon is obtained by incomplete combustion of hydrocarbon from natural gas or oil. Silicon is obtained by reduction of silica (SiO2) with high purity coke. Further purification by conversion to SiCl4 SiO2 + 2C → Si + 2CO Si + 2Cl2 → SiCl4 SiCl4 + 2Mg → Si + 2MgCl2 High purity germanium is obtained by reduction. GeO2 + 2H2 → Ge + 2H2O Tin is obtained by high temperature reduction SnO2 + 2C → Sn + 2CO (at a temperature of 1300°C) Lead is extracted from galena 2PbS + 3O2 → 2PbO + 2SO2 PbO + C → Pb + CO PbO + CO → Pb + CO2 2PbS + 3O2 → 2PbO + 2SO2 (partial roasting) 2PbO + PbS → 3Pb + SO2 (self-reduction) Descriptive Inorganic chemistry of Main groups (contd.) Physical Properties of Group IVA Metals Carbon exists as graphite (black amorphous) and diamond (colourless crystal), silicon is grey solid while other elements in the group are lustrous They have high melting and boiling points Electrical conductivity of the elements in the group shows an increase in trend down the group. There is tendency for the formation of +4 and +2 oxidation states. +4 stability decreases down the group while +2 stability increases down the group Graphite Diamond structure structure Carbon Allotropes Descriptive Inorganic chemistry of Main groups (contd.) Chemical Properties of Group IVA Metals Reaction with air: Group IVA elements react with oxygen in the air at elevated temperature to form oxides; usually in +2 and +4 oxidation state depending on the supply of Oxygen. 2C + O2 → 2CO (limited supply of O2) C + O2 → CO2 (excess supply of O2) CO2 + H2O → H2CO3 CO2 + 2NaOH → Na2CO3 + H2O SnO2 + 2NaOH → Na2[Si(OH)6] SnO2 + 4HCl → SnCl4 + 2H2O Reaction with water: C + H2O → CO + H2(steam) Si + 2H2O → SiO2 + 2H2 (steam) 2Pb + 4H2O + O2 → 2Pb(OH)2 + 2H2 ( water rich in O2) Reaction with acid: Ge + 4HCl (conc.) → GeCl4 + 2H2 Sn + 2HCl(conc.) → SnCl2 + H2 Sn + 2HNO3 (dil.) → Sn(NO3)2 + H2 Sn + 4HNO3 (conc.) → SnO2 + 4NO2 + 2H2O Sn + 2H2SO4 (conc.) → Sn(SO4) + 2H2O + SO2 Pb + 2H2SO4 (conc.) → Pb(SO4) + 2H2O + SO2 Reaction with Bases: Si + 4NaOH → Na4SiO4 + 2H2 Sn + 2NaOH + 4H2O → Na2[Sn(OH)6] + 2H2 Reaction with halogens: Si + 2X2 → SiX4 (X: F, Cl, Br, I) Ge + 2X2 → GeX4 (X: F, Cl, Br, I) Sn + 2X2 → SnX4 (X: F, Cl, Br, I) Pb + X2 → PbX2 (X: F, Cl) Descriptive Inorganic chemistry of Main groups (contd.) Chemical Properties of Group IVA Metals Carbides: Carbon reacts with less electronegative elements to form carbides. 2BeO + 2C → Be2C + CO2 4Al +3C → Al3C4 Be2C + 2H2O → 2BeO + CH4 Al3C4 + 6H2O → 2Al2O3 + 3CH4 CaO + 3C → CaC2 + CO CaC2 + 2H2O → Ca(OH)2 + C2H2 Si + C → SiC SiC + NaOH + 2O2 → Na2SiO3 + CO2 + H2O Carbon disulphide: C + S2 → CS2 CH4 + 4S → CS2 + 2H2S Hydrides: group IVA elements are known to form large number of hydrides. SiCl4 + LiAlH4 → SiH4 + LiCl + AlCl3 Si2Cl6 + 6NaH → Si2H6 + 6NaCl GeCl4 + LiAlH4 → GeH4 + LiCl + AlCl3 Ge2Cl6 + 6NaH → Ge2H6 + 6NaCl Differences between carbon and other elements in group IVA Carbon is the nonmetal in the group with the highest ionization energy. It has strong preference for the formation of covalent compounds. Carbon is known to form multiple bonds with itself and other electronegative elements. Examples include; C=C, C≡C, C=O, C≡N and C=S. Carbon also has unique ability to catenate, which results in formation of long and branched chains as well as cyclic compounds. Silicon and germanium show catenation to a limited extent. Descriptive Inorganic chemistry of Main groups (questions) 1. Describe the major trends that emerge when atomic radii are plotted against atomic number. Describe the trends observed when first ionization energies are plotted against atomic number. 2. What atom has the smallest radius among the alkaline earth elements? 3. What main group in the periodic table has elements with the most negative electron affinities for each period? 4. The ions Na+ and Mg 2+ occur in chemical compounds, but the ions Na 2+ and Mg 3+ do not. Explain. 5. Describe the major trends in metallic character observed in the periodic table of the elements. 6. Distinguish between an acidic and a basic oxide. Give examples of each. 7. What is the name of the alkali metal atom with valence shell configuration 5s1? 8. List the elements in Groups IIIA to VIA in the same order as in the periodic table. Label each element as a metal, a metalloid, or a nonmetal. Does each column of elements display the expected trend of increasing metallic characteristics? 9. For the list of elements in group IVA, note whether the oxides of each element are acidic, basic, or amphoteric. 10. A metalloid has an acidic oxide of the formula R2O3. The element has no oxide of the formula R2O5. What is the name of the element? 11. Two elements are in the same group, one following the other. One is a metalloid; the other is a metal. Both form oxides of the formula RO2. The first is acidic; the next is amphoteric (slightly acidic and basic). Identify the two elements. 12. Two elements in Period 5 are adjacent to one another in the periodic table. The ground-state atom of one element has only s electrons in its valence shell; the other has at least one d electron in an unfilled shell. Identify the elements. 13. Give the names and formulas of two oxides of carbon 14. List the unique difference of Li among group IA metals 15. What is diagonal relationship? Where does it exist? Descriptive Inorganic chemistry of Main groups (questions) 16. Which of the following: Li, Na, K and Cs will react with both oxygen and nitrogen in the air? 17. Which of the following: Li, Na, K and Mg will form superoxide when reacted with oxygen? 18. The carbonate of group IA metals used in treatment of water hardness _____ 19. An important bicarbonate in baking powder and fire extinguisher is_____ 20. Which of the following salts: NaCl, Na2CO3, CaCO3 and CaSO4 will be least stable thermally? 21. The metal hydroxide used in production of lubricating soap is ______ 22. Laundry soaps are made using which of the following bases: KOH, Mg(OH)2, LiOH and NaOH? 23. Boron shows diagonal relationship with _____ 24. An important impurity in the extraction of Be from BeCl2 via electrolysis is _____ 25. In the extraction of Mg from sea water, Mg2+ is precipitated using ____ 26. List the industrial applications of Pb, Li, NaHCO3, KO2 and Sn 27. State the variation in the oxides of group IA metals 28. State the difference in the oxide of group IIIA and IVA 29. What makes C unique in group IVA? 30. State the reason for the difference in electrical conductivity of graphite and diamond Brief introduction to transition metal Chemistry The elements of the first transition series with their electronic The transition metals are elements located between configurations and known oxidation states. (Lee, 2005) the s and p blocks of the Periodic Table. Element Electronic configuration Known oxidation states They have electrons in the d-orbitals. Hence, they are 21Sc [Ar] 4s2 3d1 +2 to +3 called d-block elements. 22Ti [Ar] 4s2 3d2 +2 to +4 Each row of the d-block metals consists of ten [Ar] 4s2 3d3 23V +2 to +5 elements. 24Cr [Ar] 4s1 3d5 +2 to +6 The name, transition metals, refers to elements with 25Mn [Ar] 4s2 3d5 +2 to +7 partially filled d-orbitals. 26Fe [Ar] 4s2 3d6 +2, +3 By definition; Zn, Zn2+, Cu, Cu+, Hg, Hg2+, Cd, Cd2+, Ag, Ag+, Au and Au+ with filled d-orbitals (d10) and Ti4+, 27Co [Ar] 4s2 3d7 +2, +3 Sc3+, V5+, Mn7+, Cr6+ with empty d-orbitals (d0) are d- 28Ni [Ar] 4s2 3d8 +2 block members but not transition metals. 29Cu [Ar] 4s1 3d10 +1, +2 However, the study of metals with d10 and d0 configuration are considered as part of transition 30Zn [Ar] 4s2 3d10 +2 metal chemistry. Brief introduction to transition metal Chemistry (contd.) General properties of transition metals From scandium to zinc, each successive member in the first row of the transition series has one electron more than the preceding element in the 3d orbitals. The continuous addition of electrons to the d-orbitals in a given row for each successive metal results in decrease in atomic size across the series. The decrease in atomic size is not regular and it is so small that the atomic sizes of the d-block metals are very close in value. Thus, the metals share similar (not the same) physical and chemical properties. All the metals are solids except mercury, which is a liquid at room temperature. They are good conductors of heat and electricity. They are hard, strong and ductile with characteristic metallic lustre. They exist in variable oxidation states because of the ease with which the d-orbital electrons are lost. They have minimum oxidation state of +1 (Cu+, Ag+, Au+) or +2 (Co2+, Mn2+, Sc2+) and maximum oxidation states, which correspond to the number of electrons in their outermost orbitals (ns and (n-1)d orbitals). Exception to this occurs when the d-orbitals contain more than five electrons. Brief introduction to transition metal Chemistry (contd.) The presence of the d-orbitals also allows the transition metals to form coordination compounds with coordination number greater than 4. CrCl3 + 6NH3 → [Cr(NH3)6]Cl3 FeCl3 + 6KCN → K3[Fe(CN3)6] + 3KCl Atomic radii: The transition metals show decrease in atomic radii on moving from the left to the right of each row but towards the end, an increase is observed with metals having d10 configuration. The decrease across each row is less than expected, which is due to the ineffective shielding of the d-orbital. The decrease in atomic size can be partially attributed to; 1. The successive addition of electrons to the penultimate 3d orbitals rather than the outer 4p and 4d orbitals in the first transition series, which is a similar trend observed in the second and third rows of the transition series. 2. The poor shielding effect of the outermost shell by the d-orbitals from the nuclear attraction. On descending each group of three metals called triads, an increase is observed in atomic radii between the first and second row metals but there is little or no difference between the atomic radii of the second and third row metals. This is due to poor shielding power of the f-orbitals which contain a series of 14 elements called lanthanides. Brief introduction to transition metal Chemistry (contd.) 3. The f-orbitals poorly shield the outermost shell from the nuclear attraction. Thus, the strong nuclear forces cause the shrinking of the atomic radii. This phenomenon is called ‘lanthanide contraction’. The atomic radii of the transition metals (10-10m). (Lee, 2005) Sc (1.44) Ti (1.32) V (1.22) Cr (1.17) Mn (1.17) Fe (1.17) Co (1.16) Ni (1.15) Cu (1.17) Zn (1.25) Y (1.62) Zr (1.45) Nb (1.34) Mo (1.29) Tc Ru (1.24) Rh (1.25) Pd (1.28) Ag (1.34) Cd (1.41) Ln (1.69) Hf (1.44) Ta (1.34) W (1.30) Re (1.28) Os (1.26) Ir (1.26) Pt (1.29) Au (1.34) Hg (1.44) Density: The contraction of the atomic radii of transition metals leads to decrease in volume and consequently increase in density. Transition metals are denser than s-block metals. Brief introduction to transition metal Chemistry (contd.) Melting and boiling points: Transition metals have very high melting and boiling points, which are often above 1000°C. This is because the d-orbitals electrons also contribute to metallic bonding. Notable exceptions are the group IIB metals, zinc, cadmium and mercury with melting point of 420°C, 321°C and -38°C respectively, where the d-orbitals electrons are all paired and not available for metallic bonding. Ionization energy: Transition metals have high ionization energies due to their small atomic radii. Therefore, they are less reactive than s-block metals. Compound of transition metals in lower oxidation are more ionic in character than those in higher oxidation state. The higher the oxidation state the higher the covalent character. Some of the transition metals are noble in nature (not very reactive) because of high ionization energy and high energy of sublimation. The noble character is present in ruthenium, rhodium, palladium, osmium, iridium, platinum and gold. Gold and platinum are relatively stable in air, which make them very attractive as jewelries. Colour in transition metals: The bright colours of transition metal ions are due to absorption of light energy in the visible region by the electrons in the partially filled d-orbitals. Examples are; Cu2+ (d9) blue, Ti3+ (d1) yellow and Fe2+ (d6) green. When a transition metal ion absorbs light energy, its electrons are excited to higher energy levels. If the absorbed energy is in the visible region, the transition metal will reflect the complementary colour of the absorbed energy. Brief introduction to transition metal Chemistry (contd.) The colours of transition metals depend on the following: 1. The nature of the metals: Each metal ion has its unique colour. Examples; Cu2+ (blue), Mn2+ (pink), Fe3+ (brown) and Zn2+ (white). 2. The metals in different oxidation states show different colours. Examples; Fe2+ (green) and Fe3+ (brown). This is due to difference in d-electronic configuration; Fe2+ (d6) and Fe3+ (d5). 3. The nature of the ligands coordinated to metal ions. Examples; [Ni(NH3)6]2+ (blue), [Ni(OH2)6]2+ (green) and [Ni(NO2)6]4- (brownish red). This is due to effect of the ligands on the d-orbitals electrons. The ligands coordinate to the metal ions using lone pairs of electrons. The approach of the lone pair for the coordination will caused the d-orbital electron to experience a repulsive force, which depends on the influence of the ligands. 4. The colour also depends on the number of ligands coordinated and the shape of the product formed after the coordination. Examples; [Cu(OH2)4]2+ (pale-blue) and [Cu(OH2)6]2+ (blue). 5. The nature of the d-electronic configuration, metal ions with d1-d9 configurations show different colours. Metal ions with d0 and d10 are generally white solids and colourless in aqueous solution. Examples; Zn2+ (white) and Ti4+ (white). This is because they do not absorb light energy in the visible region. 6. vi. Electron charge transfer mechanism can take place in transition metal ions with d10 or d0 configurations, when the ligands transfer some of their electrons to the metal empty orbitals or vice versa. Examples MnO4- d0 (purple), CrO42- d0 (yellow) and VO2+ d0 (pale yellow). The metals are coloured due to transfer of electron from the O2- ions to the empty d-orbitals of the metals. Brief introduction to transition metal Chemistry (contd.) Magnetic properties of transition metals A substance that is attracted by a magnetic field is said to be magnetic. Metals contain unpaired electrons which are in continuous random motion. However, in the presence of a magnetic field, they tend to lose their randomness and become aligned in the direction of the magnetic field. This will lead to the attraction of the metal bearing the unpaired electrons in the direction of the magnetic field. A metal with unpaired electron(s) attracted by a magnetic field is said to be paramagnetic. A metal with no unpaired electrons are not going to be attracted by a magnetic field and are said to be diamagnetic. Transition metal ions with d1-d9 electronic configuration are most likely to be paramagnetic based on the number of unpaired electrons in them, while those with d0 and d10 are diamagnetic due to absence of unpaired electrons. The higher the number of unpaired electrons the greater the magnetism. Theoretically, the spin only magnetic moment (μ(spin only)) of the transition metals can be calculated using the formula: μ(spin only) = 𝑛(𝑛 + 2) BM, n = number of unpaired electrons and BM is Bohr Magneton (9.27 x 10-24 Am2) Cu2+ d9 (n = 1) 1(1 + 2) BM = 1.73 BM Ti3+ d1 (n = 1) 1(1 + 2) BM = 1.73 BM Cr3+ d3 (n = 3) 3(3 + 2) BM = 3.87 BM Brief introduction to transition metal Chemistry (contd.) Formation of transition metal complexes A transition metal complex consists of a central metal ion surrounded by a number of molecules (NH3, H2O, CO) and/or negative ions (CN-, Cl-, O2-, NH2-) called ligands in a coordinate bonding system. For a complex to be formed, the metal must have suitable empty orbitals to receive lone pairs of electrons from the ligands. The metal is called a Lewis acid because is an electron pair acceptor and the ligands are called Lewis bases because they are electron pair donors. Example: Ag+ + 2NH3 → [Ag(NH3)2]+ FeCl2 + 6KCN → K4[Fe(CN3)6] + 2KCl A complex is described by Alfred Werner using the concept of primary and secondary valencies. The primary valency is the oxidation state of the metal and the secondary valency is the number of ligands coordinated to the metal. [Ag(NH3)2]+ primary valency is +1 and secondary valency is 2 K4[Fe(CN3)6] primary valency is +2 and secondary valency is 6 [Ni(CO)4] primary valency is 0 and secondary valency is 4 [PtCl2(NH3)2] primary valency is +2 and secondary valency is 4 Questions Determine the primary and secondary valencies of the following complexes and calculate their spin only magnetic moment. i. K[Ti(CN)4] ii. [V(NH3)4Br2] iii. [Cu(OH2)6]SO4 iv. K3[Cr(CN)6] Nuclear Chemistry Nuclear chemistry is the study of the nucleus, its constituents, and the behaviour of these constituents. The nucleus is the core (central part) of any given atom. According to atomic theory, an atom consists of a positively charged nucleus surrounded by cloud of negatively charged electrons revolving round the nucleus in orbits. The nucleus itself contains positively charged protons and electrically neutral particles called neutrons. Both the protons and neutrons are held together in the nucleus by short range attractive forces. The particles in the nucleus are called nucleons. Nuclear Structure: The structure of the nucleus has been described by the liquid drop model and the shell model 1. The liquid drop model: This is a model in which the nucleus is considered as a liquid drop. According to this model, increase in mass of the nucleus will lead to increase in volume. Consequently, there will be increase in surface tension of the nucleus. Therefore, deformation will become inevitable. Continuous increase in mass will cause the spherical shape of the nucleus to deform to oval shape. If the mass is further increased, with continuous vibration and rotation from the absorption of energy, the nucleus becomes unstable. Eventually, it will disintegrate to smaller unit. Similarly, a stable nucleus can disintegrate under an external force, when bombarded with a nucleon, just like a stable droplet will splash if it is impacted by a strong external force. Nuclear Chemistry (contd.) The disintegration from an unstable nucleus due to high mass is considered to be natural radioactivity while that resulting from impact of an external force is considered to be artificial radioactivity. This model was proposed in 1929 by George Gamow and adopted by Niels Bohr and John Archibald Wheeler to explain nuclear fission (disintegration of heavy nucleus) in 1939. The illustration of natural and artificial radioactivity based on the liquid drop model. Nuclear Chemistry (contd.) 2. The shell model: This model proposed that just has the electrons are located in different shells or energy levels which are described by four quantum numbers, the nucleons are also arranged in shell or energy levels in the nucleus. The nucleons are in ground state when they occupy the lowest energy level but may be excited to a different energy level under different conditions. The nucleus is stable, when the nucleons are in ground state. However, the nucleus becomes unstable if the nucleons are excited to higher energy level on absorption of energy. The excited nucleons will emit energy in the form of radiation on returning to the ground state. If the energy absorbed is too high the nucleus becomes very unstable and may disintegrate to smaller nuclei with release of various subatomic particles and energy. This process is most often spontaneous and it is called radioactivity. The shell model is more acceptable than the liquid drop model. The illustration of the nuclear energy level based on the shell model. Nuclear Chemistry (contd.) Nuclear stability The stability of any given nucleus is dependent on certain observed principle, which are: (i) Nuclear forces, (ii) Odd-even rule, (iii) Magic number and (iv) Neutron to proton ratio Nuclear forces: The nucleus contains both protons and neutrons, which are positively charged and neutral respectively. In a nucleus with two or more protons, electrostatic repulsion will occur between the protons because like charges repel. For the repulsion not to have effect on the binding of the nucleons, there must be another force stronger than the electrostatic repulsion. This force is often referred to as nuclear force. The nuclear forces exist between proton-proton, proton-neutron and neutron-neutron. In a stable nucleus, the nuclear forces of attraction are stronger than the electrostatic repulsion between the protons. However, the nucleus becomes unstable when the nuclear forces are less than the repulsion between the protons. An unstable nucleus will disintegrate to smaller nuclei to attain stability. The nuclear forces of attraction act within short range of 1-2.5 x 10-15 m and it is independent of the charges on the nucleons. As two atoms may be bonded by sharing of electrons, nucleons are held together in the nucleus by sharing of a subatomic particle called meson represented with “π”. Nucleons are known to contain meson and it is with the exchange of mesons that nucleons can bind together. Meson may have a positive charge (π+), a negative (π-) or no charge (π0). Nuclear Chemistry (contd.) A neutron-neutron or proton-proton attraction can take place by transfer of π0, while a proton-neutron attraction occurs by transfer of a π+ or π-. The transfer of a charged meson leads to changes in identity of the nucleons but the total number of nucleons is maintained in a stable nucleus because the changes are in equilibrium as illustrated below. The illustration of nuclear forces of attraction between nucleons. (Lee, 2005) Nuclear Chemistry (contd.) Odd-even rule: This rule proposed that nuclei with even number of protons or/and neutrons are more stable than those with odd numbers. This could be related to the noble gases with completely filled shells. The following deductions are made based on odd-even rule of nuclear stability: 1. Elements with even atomic numbers (number of protons) are more stable and more abundant than nearest elements of odd atomic number, the exception in this class is hydrogen ( 11𝐻). 2. Elements with even number of protons and neutrons are more stable than those with odd number of protons or neutrons, while those with odd numbers of protons and neutrons are rarely stable. Only four are known of such elements to be stable; 21𝐻 (deuterium), 63𝐿𝑖 (helium), 105𝐵 (boron) and 147𝑁 (nitrogen). 3. Elements with even number of protons have at least 3 stable isotopes but elements with odd number of protons have at most two stable isotopes. 4. Two elements having the same mass number (sum of the numbers of protons and neutrons) but with a 1-unit difference in atomic numbers cannot both be stable. Examples: 146𝐶 (unstable) and 147𝑁 (stable); 24 11𝑁𝑎 (unstable) and 24 64 64 12𝑀𝑔 (stable); 29𝐶𝑢 (unstable) and 28𝑁𝑖 (stable) 5. Two elements having the same mass number (sum of the numbers of protons and neutrons) and with a 2-unit difference in atomic numbers will both be stable. Examples: 64 64 28𝑁𝑖 (stable) and 30𝑍𝑛 (stable). In conclusion, these rules suggest that nucleons may be paired in nucleus just as electrons are paired in shells of covalently bonded atoms; as this allows for stability. Other factors are also known to contribute to nuclear stability than pairing. A summary Table is shown below on percentage abundance of nuclei based even-odd numbers of protons and neutrons. Nuclear Chemistry (contd.) Odd-even rule: This rule proposed that nuclei with even number of protons or/and neutrons are more stable than those with odd numbers. This could be related to the noble gases with completely filled shells. The following deductions are made based on odd-even rule of nuclear stability: 1. Elements with even atomic numbers (number of protons) are more stable and more abundant than nearest elements of odd atomic number, the exception in this class is hydrogen ( 11𝐻). 2. Elements with even number of protons and neutrons are more stable than those with odd number of protons or Abundance (%) No. of protons No. of neutrons Mass number 60 Even Even Even 40 Odd Even Odd Even Odd Odd 𝟐 𝟔 𝟏𝟎 𝟏𝐇, 𝟑𝐋𝐢, 𝟓𝐁 and 𝟏𝟒𝟕𝐍 Odd Odd Even Nuclear Chemistry (contd.) Magic number: Nuclei with certain numbers of protons and neutrons are observed to be stable. These numbers are called magic numbers, which have been associated to complete filling of the shells in nucleus just like the electronic configurations of the noble gases. The magic numbers are; 2, 8, 20, 28, 50, 82 and 126. The magic numbers stand for the number of proton or neutron and not mass number. It should be noted that the magic number is not applicable to all stable nuclei as some stable nuclei have no magic number and some unstable nuclei have magic number. Nevertheless, the concept of magic number is acceptable. Examples are shown in the Table below. Isotope No. of protons No. of neutrons Stability 𝟒 2 (magic no.) 2 (magic no.) Stable 𝟐𝐇𝐞 𝟏𝟔 8 (magic no.) 8 (magic no.) Stable 𝟖𝐎 𝟐𝟑 11 12 Stable 𝟏𝟏𝐍𝐚 𝟏𝟒 7 7 Stable 𝟕𝐍 𝟏𝟓 8 (magic no.) 7 Unstable 𝟖𝐎 𝟏𝟒 6 8 (magic no.) Unstable 𝟔𝐂 𝟒𝟎 20 (magic no.) 20 (magic no.) Stable 𝟐𝟎𝐂𝐚 𝟔𝟒 28 (magic no.) 36 Stable 𝟐𝟖𝐍𝐢 Nuclear Chemistry (contd.) Neutron to proton ratio (n/p): The ratio of number of neutrons to number of protons has been observed to also influence nuclear stability. In summary; 1. For stable light nuclei with atomic number up to 20, the n/p ratio should be one. Examples include; 168𝑂, 42𝐻𝑒, 6 24 14 3𝐿𝑖, 12𝑀𝑔 and 7𝑁. 2. Nuclei with high atomic number will experience greater repulsion between the protons. This is overcome by higher number of neutrons than protons to maintain stability. Stable heavy nuclei have n/p ratio of 1.6. Therefore, stability based on n/p ratio is given as 1.0 ≤ n/p ≤ 1.6. Above or below this range a nucleus may become unstable and it will disintegrate through different radioactive decay modes. Modes of radioactive decay An unstable nucleus with n/p ratio outside the stability range will disintegrate to attain stability. The process of disintegration also called decay process is accompanied with release of radioactive particles, which can be either alpha (α), beta (β), gamma (γ) or other subatomic particles. Alpha (α) particle: This is helium nucleus represented as 42𝐻𝑒 or 42𝛼. It is positively charged with atomic number of 2 and mass number of 4. It has the lowest penetration power and the highest ionization energy. It is very slow and can be stopped by a sheet of paper. Nuclear Chemistry (contd.) Beta (β) particle: This is an electron and it is negatively charged. Its penetration power and speed is higher than those of alpha particle but its ionization effect is lower. It is represented as −10𝛽, which implies that it has zero mass number and charge of -1. It is faster than alpha particle and it can only be stopped by aluminum sheet. Gamma (γ) particle: This is a radiation with zero rest mass and it is electrically neutral. It is the fastest of the three particles with the lowest ionization effect. It can only be stopped by thick block of lead. The speed (υ) of a radioactive particle is inversely proportional to its rest mass (μ). Thus, the heavier the particle the slower the speed The ionization effect of a particle is inversely proportional to the square of its speed. Thus, the faster the particle the lesser the ionization effect it will cause. Decay modes The following decay modes lead to decrease in n/p ratio: 1. Beta (β) particle emission: This process is believed to involve the transformation of a proton to a neutron, which accompany by the release of beta particle with a neutrino (Ʋ). The neutrino has zero rest mass and no charge. It is released to balance the nuclear spins. It has no effect in mass and charge balance during decay process. 1 1 0 0𝑛 → 1𝑝 + −1𝛽 + Ʋ; Examples: i. 146𝐶 → 147𝑁 + −10𝛽 + Ʋ ii. 24 24 0 11𝑁𝑎 → 12𝑀𝑔 + −1𝛽 + Ʋ Nuclear Chemistry (contd.) 2. Neutron emission: Neutron emission can also occur to reduce the n/p ratio. 87 86 1 36𝐾𝑟 → 36𝐾𝑟 + 0𝑛 The following decay modes lead to increase in n/p ratio: 1. Positron (β+) emission: Positron is positively charged with mass equivalent to that of electron. It is considered as the antiparticle counterpart of electron. It is also called antielectron. Positron emission involves the transformation of a proton to a neutron. This transformation is accompanied with antineutrino (Ū) just to keep the nuclear spin balanced. The emission of positron results in decrease in atomic number but no increase in mass number. 1 1 0 1𝑝 → 0𝑛 + +1𝛽 + Ū, Examples: i. 19 19 0 10𝑁𝑒 → 9𝐹 + +1𝛽 + Ū ii. 116𝐶 → 115𝐵 + +10𝛽 + Ū 2. Electron capture: This occurs when an electron in K-shell close to a nucleus is absorbed into the nucleus. This is observed, when there is insufficient energy for a positron emission to occur. The atomic number will decrease but the mass number remains unchanged. 1 0 1 1𝑝 + +1𝛽 → 0𝑛 + Ʋ, Examples: i. 195 0 195 79𝐴𝑢 + −1𝛽 → 78𝑃𝑡 + Ʋ ii. 74𝐵𝑒 + −10𝛽 → 73𝐿𝑖 + Ʋ 3. Proton emission: Proton emission also leads to increase in n/p ratio but very high energy is required and it rarely occurs. Decay mode without effect on n/p ratio: Gamma radiation: After nuclear reactions, most of the nucleons will be in excited state. To return to ground state, the nucleons usually emit gamma radiation. Gamma radiation often accompanies most nuclear reactions. Examples: i. 60 60 0 27𝐶𝑜 → 28𝑁𝑖 + −1𝛽 + γ, ii. 99 99 0 42𝑀𝑜 → 43𝑇𝑐 + −1𝛽 + γ Nuclear Chemistry (contd.) Some basic nuclear terminologies Isotopes: These are atoms with the same atomic number but different mass numbers. Examples: 1 2 3 12 13 14 15 16 1𝐻 1𝐻 1𝐻 6𝐶 6𝐶 6𝐶 8𝑂 8𝑂 Isobars: These are atoms with the same mass number but different atomic numbers. Examples: 140 140 140 140 14 14 24 24 55𝐶𝑠 56𝐵𝑎 57𝐿𝑎 58𝐶𝑒 6𝐶 7𝑁 11𝑁𝑎 12𝑀𝑔 Isotones: These are atoms with the same number of neutrons but different mass numbers. Examples: 14 15 16 6𝐶 7𝑁 8𝑂 Radioactive decay constant (λ) and half-life (𝒕𝟏/𝟐 ) The Half-life of a radioactive nuclei is the time required for half of a given population (or mass) of the radioactive 𝑑𝑁 nuclei to disintegrate. The rate of decay per unit time ( 𝑑𝑡 ) is directly proportional to the number (N) of atoms present at a given time. −𝑑𝑁 αN t = time taken, N = population at a given time and λ = decay constant 𝑑𝑡 −𝑑𝑁 = λN 𝑑𝑡 𝑁𝑡 −𝑑𝑁 𝑡 𝑡 = 𝑁 𝑁λ Nt and N0 represent the population at a given time t and at initial time t0 0 0 Nuclear Chemistry (contd.) 𝑁 −[𝑙𝑛 𝑁]𝑁𝑡0 = λt Worked Example 1 Note: = 𝑥𝑑 𝑥 ln x 1. If the half-life of a radioactive nucleus is 3 days what fraction of a [𝑙𝑛𝑁𝑡 − 𝑙𝑛𝑁0 ] = λt given sample of the nucleus will remain after 12 days? 𝑁 After 3 days, ½ is left 𝑙𝑛( 𝑡 ) = −λt or 𝑁𝑡 = 𝑁0 𝑒 −𝜆𝑡 𝑁0 Nt = ½N0 at t = 𝒕𝟏/𝟐 After 6 days, ½ (½) or (½)2 is left 0.5𝑁0 After 9 days, ½ (½) (½) or (½)3 is left 𝑙𝑛 = −λ𝒕𝟏 or 0.5𝑁0 = 𝑁0 𝑒 −𝜆𝒕𝟏/𝟐 𝑁0 𝟐 After 12 days, ½ (½) (½) (½) or (½)4 is left −𝒍𝒏𝟎.𝟓 𝟎.𝟔𝟗𝟑 𝒕𝟏/𝟐 = = 𝝀 𝝀 Therefore, fraction left after 12 days = 1/16 Note: -ln 0.5 = 0.693 Fraction left can also be derived using (½)n, where n = time given/ half- 𝟎.𝟔𝟗𝟑 λ= 𝒕𝟏/𝟐 life, n= 12/3 = 4 Nuclear Chemistry (contd.) ii. If the half-life of 222 86𝑅𝑛 is 3.8 days, how long will it take for 0.05 mmol of a sample of 222 86𝑅𝑛 to decay to 0.00625 mmol? λ = 0.693/3.8 days = 0.1824 day-1 𝑁𝑡 = 𝑁0 𝑒 −𝜆𝑡 0.00625 = 0.05𝑒 −(0.1824)𝑡 0.00625 −(0.1824)𝑡 0.05 = 𝑒 𝑙𝑛 0.125 t = −0.1824 = 11.4 days Carbon dating Carbon has three known isotopes, 12C, 13C and 14C. Carbon-14 (14C) is the heaviest and it is radioactive in nature with half-life of 5730 years. Thus, it is called radiocarbon. Its long half-life makes it useful in archeological dating. Carbon-14 is formed when atmospheric nitrogen is bombarded with neutron in cosmic radiation. The carbon-14 reacts with oxygen to for CO2 which is converted by plant to glucose through photosynthesis. 14 1 14 1 7𝑁 + 70𝑛 → 6𝐶 + 1𝐻 14C + O → 14CO 614CO2 + 6H2O → 14C6H12O6 + 6O2 2 2 The glucose is used to build plant tissues or as a source of energy. Animal can feed on the tissues, which makes carbon-14 available in all living and dead organisms. A natural balance exists between intake of radiocarbon and loss by decay. When an organism dies, the intake stops but the decay will continue. Thus, old samples of dead plants and animals will have less carbon-14 than the corresponding new samples from living plants and animals. Comparison of the amount of carbon-14 in old and new samples of a particular plant or animal can be used to determine the age of the old sample. To determine the amount of radiocarbon left in a given sample, the sample is subjected to combustion in oxygen to generate CO2 which is introduced into a radioactive counter to determine the amount of radiocarbon present. 146𝐶 → 147𝐶 + −10𝛽 Nuclear Chemistry (contd.) Example: The −10𝛽 activity of 1g of carbon from a recently cut tree is 0.26 s-1. If the activity of 1 g of carbon in a similar wood sample is 0.16 after some time under the same conditions, determine the storage time. λ = 0.693/5730 years = 1.209 x 10-4/year 𝑁𝑡 = 𝑁0 𝑒 −𝜆𝑡 0.16 = 0.26𝑒 −(0.0001209)𝑡 0.16 −(0.0001209)𝑡 0.26 = 𝑒 𝑙𝑛 0.6154 t = −0.0001209 = 4015.7 years Questions 1. Calculate the moles of 222 222 86𝑅𝑛 left after 15.2 days if the initial amount is 90mmol. The half-life of 86𝑅𝑛 is 3.8 days. (Ans.: 5.6 mmol) 2. A bone of an animal was found to emit beta particles from carbon-14. If the activity of the bone was 0.19 s-1 and that of a related recent bone of the same animal showed activity of 0.25 s-1 for the same mass sample of the bone under the same condition. Determine the age of the bone. Carbon-14 half-life is 5730 years. (Ans.: 2268 years). 3. A rock contains 0.257 mg of 206 238 238 206 9 82𝑃𝑏 for every mg of 92𝑈. The half-life of the decay of 92𝑈 to 82𝑃𝑏 is 4.5 x 10 years. How old is the rock? (Ans.: 1.7 x 109 years) Nuclear Chemistry (contd.) Nuclear binding energy and binding energy per nucleon The binding energy is the energy released during the formation of a nucleus from the appropriate number of neutrons, protons and electrons. Nuclear binding energy is determined by calculating the difference in mass of the nucleus and the sum of the masses of all its subatomic particles. This is called mass defect. The mass defect and energy are related by Einstein theory of mass and energy relationship. ΔE = ΔmC2, ΔE = binding energy, Δm = mass defect, C = speed of light (3 x108 m/s) When mass is in atomic mass unit (amu), 1 amu = 931 MeV and 1MeV = 106(1.6 x 10-19) V. Binding energy per nucleon is the energy required to pull out a nucleon from the nucleus. it is obtained by dividing the total binding energy by the number of nucleons in the nucleus. Example: calculate the binding energy of helium, given that it is formed by: 2 11𝐻 + 2 10𝑛 → 42𝐻𝑒 1 1 4 1𝐻 = 1.0078 amu 0𝑛 = 1.00087 amu 2𝐻𝑒 = 4.0026 Δm = 2(1.0078) + 2(1.0087) – 4.0026 = 0.0304 ΔE = 931 x 0.0304 = 28.3024 MeV Binding energy per nucleon = 28.3024/4 = 7.0756 MeV Question 1. calculate the energy released during the nuclear reaction : 32𝐻𝑒 + 10𝑛 → 42𝐻𝑒 + ΔE 3 1 4 2𝐻𝑒 = 3.0160 amu 0𝑛 = 1.00087 amu 2𝐻𝑒 = 4.0026 (Ans.: 20.58 MeV) 1. Calculate the total binding energy and binding energy per nucleon for i. 24 12𝑀𝑔 with mass = 23.9850 amu and ii. 60 1 1 27𝑐𝑜 with mass = 59.9338 amu. Given that 1𝐻 = 1.0078 amu and 0𝑛 = 1.00087 amu Nuclear Chemistry (contd.) Nuclear reactions A nuclear reaction can be a spontaneous disintegration, which is associated with unstable nucleus. There can also be induced nuclear reaction, when a stable nucleus is bombarded with a subatomic particle such as beta particle, neutron or alpha particles. The is called artificial radioactivity. 30 14 7𝑁 + 42𝐻𝑒 → 17 8𝑂 + 11𝐻 + ΔE 27 13𝐴𝑙 + 42𝐻𝑒 → 15𝑃 + 10𝑛 + ΔE 1. Nuclear fusion is another induced radioactivity, which involves the breaking down a heavy nucleus into two or more smaller nuclei, when the heavy nucleus is bombarded with a subatomic particle such as neutron. Huge energy is released during this process and it is the reaction behind nuclear power plants. In nuclear power plant, the energy is converted to electricity. 235 138 140 92𝑈 + 10𝑛 → 53𝐼 + 95 39𝑌 + 2 10𝑛 + ΔE 235 92𝑈 + 10𝑛 → 55𝐶𝑠 + 92 37𝑅𝑏 + 4 10𝑛 + ΔE 2. Nuclear fusion involves bombardment of two light-weight nuclei to give a relatively heavier nucleus with release of huge energy. This is the process by which solar radiation is generated. 2 1𝐻 + 31𝐻 → 42𝐻𝑒 + 10𝑛 + ΔE Nuclear Chemistry (contd.) Detection and measurement of radiation Devices used in the detection and measurement of radiation depend on the ionization effects of radiation particles and their photographic effect on plate. Examples of the detectors are: Gas filled detectors: These devices depend on the ionization effect caused by radiation on gas. Examples include; ionization chamber, proportional counter and Geiger-Muller counter. Scintillation counters: These counters depend on the absorption of radiation by organic or inorganic crystals called phosphor. On absorption of radiation, the phosphor will emit light energy called photon. Examples of organic phosphors are; anthracene and stilbene, while inorganic phosphors include; sodium iodide and zinc sulphide. Applications of some radioactive isotopes. Nucleus Nuclear activity Application 𝟏𝟒 beta emission Archeological dating 𝟔𝐂 𝟏𝟏 positron emission Brain scan 𝟔𝐂 𝟑𝟐 beta emission Detection of breast tumors 𝟏𝟓𝐏 𝟏𝟓 positron emission Lung function diagnosis 𝟖𝐎 𝟏𝟑𝟏 beta emission Measurement of iodine take up by thyroid 𝟓𝟑𝐈 Nuclear Chemistry (contd.) References 1. Babarinde, A., Atewolara-Odule, O. C. and Ogundare S. A. Introduction to organic and inorganic chemistry, Darosat, Nigeria, 2020 2. Choppin, G. and Baisden, P. Radiochemistry. America Chemical Society Washington D. C. 1978 3. De, A. Ke. A Textbook of Inorganic Chemistry (9th ed.) New Age International, New Delhi, 2003. 4. Ebbing, D. D. and Gammon, S. D. General Chemistry (9th ed.) Houghton Mifflin Company, USA, 2009. 5. Lee, J. D. Concise Inorganic Chemistry (5th ed.) Willey-Blackwell Science, London, 2005 6. Miessler, G. L. and Tarr, D. A. Inorganic Chemistry (3rd ed.) Pearson Education Int, 2010. 7. Wilson, J. G. and Newall, A. B. General and Inorganic Chemistry. Cambridge University Press, London, 1966. End of lecture