Radio Navigation PDF
Document Details
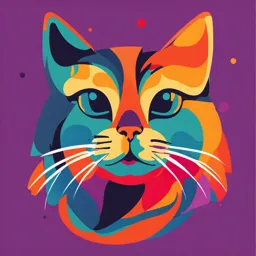
Uploaded by IntimateSelenium
University of Gazig National - Engineering Sector
Tags
Summary
This document provides a comprehensive introduction to radio navigation, focusing on automatic direction finder (ADF) systems and non-directional beacons (NDBs). It details the principles of operation, frequencies, uses in aviation, and factors that affect the accuracy and range of ADF.
Full Transcript
RADIO NAVIGATION Chapter 7 AUTOMATIC DIRECTION FINDER ❑ NON DIRECTIONAL BEACON ( NDB ) ❑ PRINCIPLE OF OPERATION ❑ CORRECT NULL ❑ FREQUENCIES AND TYPES OF NDB ❑ AIRCRAFT EQUIPME ❑ EMISSION CHARACTERISTICS and BEAT FREQUENCY OSCILLATOR (BFO) ❑ PRESENTATION OF INFORMATION ❑ US...
RADIO NAVIGATION Chapter 7 AUTOMATIC DIRECTION FINDER ❑ NON DIRECTIONAL BEACON ( NDB ) ❑ PRINCIPLE OF OPERATION ❑ CORRECT NULL ❑ FREQUENCIES AND TYPES OF NDB ❑ AIRCRAFT EQUIPME ❑ EMISSION CHARACTERISTICS and BEAT FREQUENCY OSCILLATOR (BFO) ❑ PRESENTATION OF INFORMATION ❑ USES OF THE NON DIRECTIONAL BEACON ❑ PLOTTING ADF BEARINGS ❑ TRACK MAINTENANCE USING THE RBI ❑ INBOUND TRACKING ❑ MAINTAINING AN INBOUND TRACK IN A CROSSWIND ❑ OUTBOUND TRACKINGDRIFT ASSESSMENT AND REGAINING INBOUND TRACK ❑ DRIFT ASSESSMENT AND OUTBOUND TRACK MAINTENANCE HOLDING. ❑ RUNWAY INSTRUMENT APPROACH PROCEDURES. ❑ FACTORS AFFECTING ADF ACCURACY ❑ FACTORS AFFECTING ADF RANGE ACCURACY ADF SUMMARY INTRODUCTION Automatic Direction Finder ( ADF) equipment in the aircraft is used in conjunction with a simple low and medium frequency non-directional beacon (NDB) on the ground to provide an aid for navigation and for non- precision approaches to airfields. However, it was due to be phased out in the 2005, but still continues in use. Indeed, many UK aerodromes still have NDB instrument approach procedures, and it is the only instrument approach procedure available at some aerodromes. NON DIRECTIONAL BEACON ( NDB ) The Non Directional Beacon (NDB) is a ground based transmitter which transmits vertically polarized radio signals, in all directions (hence the name), in the Low Frequency (LF) and Medium Frequency (MF) bands. When an aircraft’s Automatic Direction Finding (ADF) is tuned to an NDB’s frequency and its callsign identified, the direction of the NDB will be indicated. A ‘cone of silence’ exists overhead the NDB transmitter during which the aircraft does not receive any signals. The diameter of the cone increases with aircraft height. PRINCIPLE OF OPERATION The ADF measures the bearing of a NDB relative to the fore/aft axis of the aircraft. If a loop aerial is placed in the plane of the transmitted radio frequency a voltage will be generated in the vertical elements of the loop because of the phase difference of the wave in each of the vertical elements. As the loop is rotated the voltage induced will decrease until it becomes zero when the loop is perpendicular to the radio wave. As the loop continues to rotate a voltage will be induced in the opposite sense etc. The ADF works by using the electromagnetic properties of the signal produced by the beacon. Two antennae are required, which are known as the loop antenna and the sense antenna. The Loop Antenna: The loop antenna can be simplistically described as two insulated coils of wire wound perpendicular to each other onto a ferrite core. The bi-directional antenna is horizontally polarized, and couples with the magnetic component of the beacon signal. The maximum voltage is induced when the antenna coil is perpendicular to the transmitter. As the antenna pattern contains two nulls, it cannot determine whether the signal is from the 0 or 180 position, hence the need for the sense antenna. The Sense Antenna: In its basic form, this can be a long wire antenna, often seen mounted from the aircraft cabin roof to the tail fin. For more modern types of antennae, both loop and sense are located in the same teardrop- shaped housing, mounted as near to the aircraft centreline as possible. This omnidirectional capacitive antenna couples with the electric component of the signal. The Composite Effect: In a typical ADF receiver, the signals received by the loop and sense antennae are combined to create the equivalent of a cardioid pattern, The polar diagram It can be seen that there are two null positions and that by rotating the loop until a null is reached the direction of the beacon can be determined. This is fine if the approximate direction of the beacon is known, but if that is not the case then there are two possible choices. Furthermore, if equipment is to automatically determine position, then with only the single loop it would have an insoluble problem. To resolve this ambiguity a simple dipole aerial, called a sense aerial, is added. The polar diagram of the sense aerial is circular. The currents generated are combined electronically as if It is arranged for the field from the sense aerial to be in phase with the one element of the loop aerial The resultant polar diagram is known as a CARDIOID. The cardioid has a single null which as can be seen is ill-defined and would not in itself provide an accurate bearing. However, the correct null in the loop aerial can be defined by introducing a logic circuit which defines the correct null as being that null, in the loop aerial which, when the loop aerial is rotated clockwise, produces an increase in signal strength in the cardioid. The resultant null with a single cardioid is not precise enough to meet the ICAO accuracy requirement of +/-5°. To improve the accuracy to meet the requirements, the polarity of the sense aerial is reversed to produce a right hand cardioid. Then by rapidly switching (about 120 Hz) between the two cardioids, the null is more precisely defined and hence the accuracy is improved. In reality it is not feasible to have a rotating loop outside the aircraft, so the loop is fixed and has four elements, two aligned with the fore-aft axis of the aircraft with the other two perpendicular to the fore-aft axis. The electrical fields are transmitted to a similar four elements in a goniometer reproducing the electro-magnetic field detected by the aerial. The signal from the sense aerial is also fed to the goniometer where a search coil detects the unambiguous direction. The principle employed within the goniometer is as described above. FREQUENCIES AND TYPES OF NDB The allocated frequencies for NDB,s are 190 - 1,750 kHz in the LF and MF bands. Since the mode of propagation used is surface wave, most NDBs will be found between about 250 and 450 kHz. There are two types of NDB in current use: Locator (L). These are low powered NDBs used for airfield or runway approach procedures or are co-located with, and supplement, the outer and middle markers of an ILS system. They normally have ranges of 10 to 25nm and may only be available during an aerodromes published hours of operation. En route NDBs. These have a range of 50nm or more, and where serving oceanic areas may have ranges of several hundred miles. They are used for homing, holding, en route and airways navigation. AIRCRAFT EQUIPMENT The aircraft equipment comprises: ▪ A loop aerial ▪ A sense aerial ▪ A control unit ▪ A receiver ▪ A display EMISSION CHARACTERISTICS and BEAT FREQUENCY OSCILLATOR (BFO) The NDBs have a 2 or 3 letter identification and there are two types of emission: N0NA1A N0NA2A The N0N part of the emission is the transmission of an unmodulated carrier wave, which would not be detectable on a normal receiver, so a BFO is provided on ADF equipment. When selected, the BFO produces an offset frequency within the receiver which when combined with the received frequency produces a tone of say 400 or 1,020 Hz. The A1A part is the emission of an interrupted unmodulated carrier wave which requires the BFO to be on for aural reception. A2A is the emission of an amplitude modulated signal which can be heard on a normal receiver. Hence, when using N0NA1A beacons, the BFO should be selected ON for (manual) tuning, identification and monitoring. N0NA2A beacons require the BFO ON for (manual) tuning but OFF for identification and monitoring. (The BFO may be labelled TONE or TONE/VOICE on some equipment's). PRESENTATION OF INFORMATION The information may be presented on a relative bearing indicator (RBI) or a radio magnetic indicator (RMI). In either case the information being presented is relative bearing. The RBI has a standard compass rose where 360° is aligned with the fore aft axis of the aircraft, although with some RBIs it is possible to manually set heading to directly read the magnetic bearing. In the diagram the aircraft is heading 300°(M), the RBI is showing a relative bearing of 136°, thus the magnetic bearing is 300°+136°-360°=076°. The information from the ADF to the RMI is still relative, but the RMI compass card is fed with magnetic heading, so the bearing shown is the magnetic bearing of the NDB. The needle always points to the beacon (QDM) and the tail of the needle gives the QDR. USES OF THE NON DIRECTIONAL BEACON ▪ En-route navigational bearings. ▪ Homing to or flying from the NDB when maintaining airway centre-lines. ▪ Holding overhead at an assigned level in a race-track pattern. ▪ Runway instrument approach procedures. PLOTTING ADF BEARINGS The plotting of ADF bearings is dealt with in depth in the Navigation General syllabus. At this stage it is sufficient to remind the reader that the bearing is measured at the aircraft so variation to convert to a true bearing must be applied at the aircraft. Account will also need to be taken of the convergency between the aircraft and beacon meridians. TRACK MAINTENANCE USING THE RBI ▪ An aircraft is required to maintain track(s): ▪ When flying airway centre-line between NDBs. ▪ When holding over an NDB or Locator. ▪ When carrying out a let-down procedure at an airfield based solely upon ▪ NDB(s)/Locator(s) or NDB(s)/Locators combined with other navaids. ▪ When requested by ATC to intercept and maintain a track or airway centre-line. DRIFT ASSESSMENT AND REGAINING INBOUND TRACK Initially, fly the aircraft on the required track with the beacon dead ahead (000°rel.). Maintain the aircraft heading and watch the relative bearing indicator. If the relative bearing increases the aircraft is experiencing port drift. Alter heading, say 30° starboard, to regain track. The relative bearing will become 330° when track is regained. Assume a likely drift (say10° port) and calculate a new heading to maintain track. When this heading has been taken up, the relative bearing will become 350°. If the drift has been correctly assessed this relative bearing will be maintained until overhead the NDB. If the relative bearing changes however, further heading alterations and a new assessment of drift will be necessary. HOLDING THE HOLDING SYSTEM When density of traffic or bad weather delay an aircraft’s landing at an airport, the air traffic controller directs it to a Holding Area. The area, also known as ‘stack’, is organised over a ‘radio’ beacon where each waiting aircraft flies a special circuit separated vertically from other aircraft by a minimum of 1,000ft. An aircraft drops to the next level as soon as it is free of other traffic, until it finally flies from the stack and comes in to land. FACTORS AFFECTING ADF ACCURACY Designated Operational Coverage (DOC). The DOC of NDBs is based upon a daytime rotection ratio (signal/noise ratio of 3:1) between wanted and unwanted signals that permits the required level of bearing accuracy. At ranges greater than those promulgated, bearing errors will increase. Adverse propagation conditions particularly at night will also increase bearing errors. Static Interference. There are two types of static interference that can affect the performance of ADF: Precipitation static. Precipitation static is generated by the collision of water droplets and ice crystals with the aircraft. It causes a reduction in the signal: noise ratio which affects the accuracy of the bearings and can, in extreme circumstances completely mask the incoming signal. The indications on the RMI/RBI will be a wandering needle and the audio will have a background hiss, which is also likely to be present on VHF frequencies. Thunderstorms. Thunderstorms have very powerful discharges of static electricity across the electromagnetic spectrum including LF and MF. These discharges cause bearing errors in the ADF. A static discharge in a cumulonimbus cloud (Cb) will be heard as a loud crackle on the audio and the needle will move rapidly to point to the Cb. When there are several active cells close together, it is possible for the needle to point to them for prolonged periods. Care must be taken in the use of ADF when Cb activity is forecast. It has been said that during Cb activity the only sensible use of the ADF is to indicate where the active cells are. Night Effect. By day the D-region absorbs signals in the LF and MF bands. At night the D- region disappears allowing skywave contamination of the surface wave being used. This arises for two reasons: phase interference of the skywave with the surface wave because of the different paths and the induction of currents in the horizontal elements of the loop aerial. The effect is reduced by the aerial design having very short vertical elements and by screening the aerial above and below, but the contamination is not eliminated. The effect first becomes significant at 70 - 100nm from the NDB. The effect is manifest by fading of the audio signal and the needle ‘hunting’ and is worst around dawn and dusk, when the ionosphere is in transition. To minimize the above effects: ❑ Positively identify the NDB callsign. ❑ Continue to check the tuning and the identification. ❑ Avoid use of the equipment within 1 hour of sunrise or sunset. ❑ Use NDBs within their promulgated range which is valid during daytime only. ❑ Treat bearings with caution if the needle wanders and the signal fades. ❑ Cross check NDB bearing information against other navigation aids. Station Interference. Due to congestion of stations in the LF and MF bands, the possibility of interference from stations on or near the same frequency exists. This will cause bearing errors. By day, the use of an NDB within the DOC will normally afford protection from interference. However, at night, one can expect interference even within the DOC because of skywave contamination from stations out of range by day. Therefore positive identification of the NDB at night should always be carried out. Mountain Effect. Mountainous areas can cause reflections and diffraction of the transmitted radio waves to produce errors in ADF systems. These errors will increase at low altitude and can be minimized by flying higher. Coastal Refraction. Radio waves speed up over water due to the reduced absorption of energy (attenuation) compared to that which occurs over land. This speeding up causes the wave front to bend (Refract) away from its normal path and pull it towards the coast. Refraction is negligible at 90 to the coast but increases as the angle of incidence increases. For an aircraft flying over the sea the error puts the aircraft position closer to the coast than its actual position. The effect can be minimized by: Using NDBs on or near to the coast. Flying higher. Using signals that cross the coast at or near to 90° Lack of Failure Warning System. False indications due to a failure in the system are not readily detectable because of the absence of failure warning on most ADF instruments. Particular care should therefore be exercised in identifying and monitoring the NDB and independent cross checks made with other navigational aids where possible. It is essential that when using the ADF as the primary navigation aid, for example for a runway approach procedure, that it is continuously monitored to detect any failure. Quadrantal Error. The theoretical reception polar diagram of the loop aerial is distorted by the airframe which produces a strong electrical field aligned fore and aft. Incoming NDB signals are thus refracted towards the fore and aft airframe axis. The maximum refraction occurs in the quadrants (ie on relative bearings of 045°, 135°, 225° & 315°. Older ADF systems are regularly ‘swung’ to assess the value of quadrantal error. In modern aircraft the error is determined by the manufacturer and corrections are put into the equipment to reduce the effect to a minimum. Angle of Bank (dip). A loop aerial is designed to use vertically polarized waves for direction finding. If the incoming wave has any horizontal component of polarization it will induce currents in the top and bottom horizontal members of the loop resulting in a circulating current. This would destroy the nulls of polar diagram ( similar to night effect ) and reduce the accuracy of the bearings. The angle of bank during a turn causes emfs to be induced in the horizontal elements of the loop thereby leading to a bearing error which is referred to as dip error. This error is only present when the aircraft is not in level flight. FACTORS AFFECTING ADF RANGE ACCURACY The accuracy of ADF is +/-5° within the designated operational coverage, by day only. This refers to the measured bearing and does not include any compass error. ADF SUMMARY NDB Ground transmitter in LF or MF band ( 190 - 1750 kHz ) Types of NDB: Locator (L) - airfield let-down (10 - 25 nm) En Route - Nav-aid (50 nm or more) Range (nm) 3 x √P (W) over water 2 x √P (W) over land ADF Airborne equipment - aerials, receiver, control unit, indicator (RBI / RMI) Principle of operation (Relative) Bearing by switched cardioids Frequencies 190 - 1750 kHz (LF & MF) Emission characteristics N0NA1A - BFO ON for tuning, identification and monitoring N0NA2A - BFO ON for tuning, OFF otherwise Presentation RBI or RMI Uses of NDB Homing, Holding, Approach, En route nav-aid Errors Static interference (precipitation and thunderstorms) Station interference Night effect Mountain effect Coastal refraction Quadrantal error Bank angle (dip) Lack of failure warning Accuracy (Day Only) +/- 5° within the DOC