CAE ATPL 11. Radio Navigation PDF
Document Details
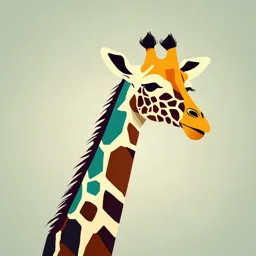
Uploaded by FelicitousEnlightenment
CAE Oxford Aviation Academy
2014
EASA
Tags
Summary
This is a textbook covering radio navigation topics for the EASA ATPL theoretical knowledge examinations. It examines radio wave properties, radio propagation, various navigation systems such as VOR, ILS, and DME, and includes practice questions.
Full Transcript
15 RADIO NAVIGATION 18 ATPL GROUND TRAINING SERIES 21 24 3 27 30 I Introduction © CAE Oxford Aviation Aca...
15 RADIO NAVIGATION 18 ATPL GROUND TRAINING SERIES 21 24 3 27 30 I Introduction © CAE Oxford Aviation Academy (UK) Limited 2014 I All Rights Reserved Introduction This text book is to be used only for the purpose of private study by individuals and may not be reproduced in any form or medium, copied, stored in a retrieval system, lent, hired, rented, transmitted or adapted in whole or in part without the prior written consent of CAE Oxford Aviation Academy. Copyright in all documents and materials bound within these covers or attached hereto, excluding that material which is reproduced by the kind permission of third parties and acknowledged as such, belongs exclusively to CAE Oxford Aviation Academy. Certain copyright material is reproduced with the permission of the International Civil Aviation Organisation, the United Kingdom Civil Aviation Authority and the European Aviation Safety Agency (EASA). This text book has been written and published as a reference work to assist students enrolled on an approved EASA Air Transport Pilot Licence (ATPL) course to prepare themselves for the EASA ATPL theoretical knowledge examinations. Nothing in the content of this book is to be interpreted as constituting instruction or advice relating to practical flying. Whilst every effort has been made to ensure the accuracy of the information contained within this book, neither CAE Oxford Aviation Academy nor the distributor gives any warranty as to its accuracy or otherwise. Students preparing for the EASA ATPL (A) theoretical knowledge examinations should not regard this book as a substitute for the EASA ATPL (A) theoretical knowledge training syllabus published in the current edition of ‘Part-FCL 1’ (the Syllabus). The Syllabus constitutes the sole authoritative definition of the subject matter to be studied in an EASA ATPL (A) theoretical knowledge training programme. No student should prepare for, or is currently entitled to enter himself/herself for the EASA ATPL (A) theoretical knowledge examinations without first being enrolled in a training school which has been granted approval by an EASA authorised national aviation authority to deliver EASA ATPL (A) training. CAE Oxford Aviation Academy excludes all liability for any loss or damage incurred or suffered as a result of any reliance on all or part of this book except for any liability for death or personal injury resulting from CAE Oxford Aviation Academy’s negligence or any other liability which may not legally be excluded. Printed in Singapore by KHL Printing Co. Pte Ltd ii Introduction I Textbook Series I Introduction Book Title Subject 1 010 Air Law 2 020 Aircraft General Knowledge 1 Airframes & Systems Fuselage, Wings & Stabilising Surfaces Landing Gear Flight Controls Hydraulics Air Systems & Air Conditioning Anti-icing & De-icing Fuel Systems Emergency Equipment 3 020 Aircraft General Knowledge 2 Electrics – Electronics Direct Current Alternating Current 4 020 Aircraft General Knowledge 3 Powerplant Piston Engines Gas Turbines 5 020 Aircraft General Knowledge 4 Instrumentation Flight Instruments Warning & Recording Automatic Flight Control Power Plant & System Monitoring Instruments 6 030 Flight Performance & Planning 1 Mass & Balance Performance 7 030 Flight Performance & Planning 2 Flight Planning & Monitoring 8 040 Human Performance & Limitations 9 050 Meteorology 10 060 Navigation 1 General Navigation 11 060 Navigation 2 Radio Navigation 12 070 Operational Procedures 13 080 Principles of Flight 14 090 Communications VFR Communications IFR Communications iii I Introduction I Introduction iv Introduction I Contents I Introduction ATPL Book 11 Radio Navigation 1. Properties of Radio Waves................................... 1 2. Radio Propagation Theory.................................. 17 3. Modulation.......................................... 41 4. Antennae............................................ 53 5. Doppler Radar Systems.................................... 65 6. VHF Direction Finder (VDF).................................. 73 7. Automatic Direction Finder (ADF).............................. 83 8. VHF Omni-directional Range (VOR)............................ 109 9. Instrument Landing System (ILS).............................. 145 10. Microwave Landing System (MLS)............................. 169 11. Radar Principles....................................... 181 12. Ground Radar........................................ 197 13. Airborne Weather Radar.................................. 205 14. Secondary Surveillance Radar (SSR)............................ 225 15. Distance Measuring Equipment (DME).......................... 241 16. Area Navigation Systems (RNAV).............................. 259 17. Electronic Flight Information System (EFIS)........................ 283 18. Global Navigation Satellite System (GNSS)......................... 303 19. Revision Questions...................................... 329 20. Index............................................. 381 v I Introduction I Introduction vi Chapter 1 Properties of Radio Waves Introduction.............................................. 3 The Radio Navigation Syllabus.................................... 3 Electromagnetic (EM) Radiation................................... 3 Polarization.............................................. 4 Radio Waves.............................................. 5 Wavelength.............................................. 6 Frequency Bands........................................... 8 Phase Comparison.......................................... 9 Practice Frequency (f) - Wavelength (λ) Conversions....................... 11 Answers to Practice Frequency (f) - Wavelength (λ) Conversions................. 12 Questions............................................... 13 Answers................................................ 16 1 1 Properties of Radio Waves 1 Properties of Radio Waves 2 Properties of Radio Waves 1 Introduction 1 Properties of Radio Waves Radio and radar systems are now an integral and essential part of aviation, without which the current intensity of air transport operations would be unsustainable. In the early days of aviation aircraft were flown with visual reference to the ground and flight at night, in cloud or over the sea was not possible. As the complexity of aircraft increased it became necessary to design navigational systems to permit aircraft to operate without reference to terrain features. The early systems developed were, by modern standards very basic and inaccurate. They provided reasonable navigational accuracy for en route flight over land, but only a very limited service over the oceans, and, until about 40 years ago, flight over the oceans used the traditional seafarer’s techniques of astro-navigation, that is using sights taken on the sun, moon, stars and planets to determine position. Developments commenced in the 1910s, continued at an increasing rate during the 1930s and 1940s and up to the present day leading to the development of long range systems which by the 1970s were providing a global navigation service. It is perhaps ironic that, having forsaken navigation by the stars, the most widely used navigation systems in the last few years are once again space based, that is the satellite navigation systems we now take as being the norm. Whilst global satellite navigation systems (GNSS) are becoming the standard in aviation and many advocate that they will replace totally all the terrestrial systems, the ICAO view is that certain terrestrial systems will have to be retained to back up GNSS both for en route navigation and runway approaches. The development of radar in the 1930s allowed air traffic control systems to be developed providing a control service capable of identifying and monitoring aircraft such that aircraft operations can be safely carried out at a much higher intensity than would be otherwise possible. Modern satellite technology is being used to provide a similar service over oceans and land areas where the provision of normal radar systems is not possible. The Radio Navigation Syllabus The syllabus starts by looking at the nature of radio waves and how they travel through the atmosphere. This is essential to understand why different radio frequencies are selected for particular applications and also the limitations imposed. The introductory chapters also cover how radio waves are produced, transmitted, received and how information is added to and recovered from radio waves. Electromagnetic (EM) Radiation If a direct electric current (DC) is passed through a wire then a magnetic field is generated around the wire perpendicular to the current flow. If an alternating electric current (AC) is passed through the wire then, because the direction of current flow is changing, the polarity of the magnetic field will also change, reversing polarity as the current direction reverses. At low frequencies the magnetic field will return to zero with the current, but as frequency increases the magnetic field will not have collapsed completely before the reversed field starts to establish itself and energy will start to travel outwards from the wire in the form of electromagnetic radiation i.e. radio waves. 3 1 Properties of Radio Waves The resulting EM energy is made up of two components, an electrical (E) field parallel to the 1 wire and a magnetic (H) field perpendicular to the wire. Properties of Radio Waves Figure 1.1 Vertical polarization Polarization The polarization of radio waves is defined as the plane of the electric field and is dependent on the plane of the aerial. A vertical aerial will emit radio waves with the electrical field in the vertical plane and hence produce a vertically polarized wave, and a horizontal aerial will produce a horizontally polarized wave. To receive maximum signal strength from an incoming radio wave it is essential the receiving aerial is in the same plane as the polarization of the wave, so a vertically polarized radio wave would require a vertical aerial. Circular polarization can be produced in a variety of ways, one of which is using a helical antenna. In circular polarization the electrical (and hence magnetic) field rotates at the frequency of the radio wave. The rotation may be right handed or left handed dependent on the orientation of the aerial array. For reception of a circularly polarized wave an aerial of the same orientation is required, or a simple dipole aerial. There are two significant advantages. Firstly in radar systems, if circular polarization is used, when the energy is reflected from water droplets the circularity is reversed and therefore the ‘clutter’ caused by precipitation can be eliminated. Secondly, if a dipole aerial is used the orientation of the aerial is no longer critical, as it is with linear polarization, and, clearly, this will be a major advantage in mobile systems, such as cellular phones and satellite communication and navigation systems. 4 Properties of Radio Waves 1 Radio Waves 1 Properties of Radio Waves The length of time it takes to generate one cycle of a radio wave is known as the period and is generally signified by the Greek letter tau (τ), and measured in microseconds (µs). (1 µs = 10-6 second). Figure 1.2 Sinusoidal wave - period If, for example, the period of one cycle of a radio wave is 0.125 µs then the number of cycles produced in one second would be the reciprocal of this giving: 1 1 = = 8 000 000 cycles per second which are known as hertz (Hz) τ 0.125 ×10-6 This is known as the frequency (f) of the wave; hence: 1 f = (1) τ The frequency of radio waves is expressed in hertz (Hz). Since the order of magnitude of the frequency of radio waves is very high, for convenience, the following terms are used to express the frequency: Kilohertz (kHz) = 103 Hz = 1 000 Hz Megahertz (MHz) = 106 Hz = 1 000 000 Hz Gigahertz (GHz) = 109 Hz = 1 000 000 000 Hz So in the example above the frequency would be expressed as 8 MHz. 5 1 Properties of Radio Waves Wavelength 1 Properties of Radio Waves The speed of radio waves (c) is the same as the speed of light (which is also EM radiation) and is approximately: 300 000 000 ms-1 (= 300 × 106 ms-1), or 162 000 nautical miles per second Wavelength ( λ ) Figure 1.3 Sinusoidal wave - wavelength If a radio wave travels at 300 × 106 ms-1 and the period is 0.125 µs, then the length (λ) of each wave will be: λ = c. τ (2) 300 × 106 × 0.125 × 10-6 = 37.5 m This is known as the wavelength. From equation (1) this can also be stated as: c λ = (3) f Giving: λ 300 × 106 = = 37.5 m 8 × 106 Hence if the frequency is known then the wavelength can be determined and if the wavelength is known then the frequency can be calculated from: c (4) f = λ 6 Properties of Radio Waves 1 Examples: 1 1. If the frequency of a radio wave is 121.5 MHz calculate the wavelength. Properties of Radio Waves c 300 × 106 λ = = = 2.47 m f 121.5 × 106 2. If the wavelength is 1515 m, what is the corresponding frequency? c 300 × 106 f = = = 198 000 Hz = 198 × 103 Hz = 198 kHz λ 1515 For ease of calculation we can simplify the formulae: 300 f = MHz λ (m) 300 λ = m f (MHz) But we must ensure that our input arguments are correct, i.e. to calculate the frequency the wavelength must be in metres and to calculate the wavelength the frequency must be input in MHz. Examples: 3. Determine the frequency corresponding to a wavelength of 3.2 cm. Noting that 3.2 cm = 0.032 m the calculation becomes: 300 f = = 9375 MHz (or 9.375 GHz) 0.032 4. Determine the wavelength corresponding to a frequency of 357 kHz. Noting that 375 kHz = 0.375 MHz the calculation is: 300 λ = = 800 m 0.375 7 1 Properties of Radio Waves Frequency Bands 1 Properties of Radio Waves The radio part of the electromagnetic spectrum extends from 3 kHz to 300 GHz. For convenience it is divided into 8 frequency bands. These are shown below with the frequencies, wavelengths and the uses made of the frequency bands in civil aviation. Note that each frequency band is related to its neighbouring band(s) by a factor of 10. Frequency Band Frequencies Wavelengths Civil Aeronautical Usage Very Low Frequency (VLF) 3 – 30 kHz 100 – 10 km Nil Low Frequency (LF) 30 – 300 kHz 10 – 1 km NDB/ADF NDB/ADF, long range Medium Frequency (MF) 300 – 3000 kHz 1000 – 100 m communications long range High Frequency (HF) 3 – 30 MHz 100 – 10 m communications Short range Very High Frequency communication, VDF, 30 – 300 MHz 10 – 1 m (VHF) VOR, ILS localizer, marker beacons ILS glide path, DME, SSR, Ultra High Frequency 300 – 3000 MHz 100 – 10 cm Satellite communications, (UHF) GNSS, long range radars Super High Frequency RADALT, AWR, MLS, short 3 – 30 GHz 10 – 1 cm (SHF) range radars Extremely High 30 – 300 GHz 10 – 1 mm Nil Frequency (EHF) 8 Properties of Radio Waves 1 Phase Comparison 1 Properties of Radio Waves Some radio navigation systems use the comparison of phase between two signals to define navigational information. The first important point is that the two signals being compared must have the same frequency, otherwise any phase comparison would be meaningless. The second point is that one signal will be designated the reference signal and the other a variable signal and that the comparison must yield a positive result. Figure 1.4 Sinusoidal wave - phase comparison To determine the phase difference between 2 signals, first identify the position of (for example) zero phase on each of the waves, then move in the positive direction from the chosen point on the reference wave to measure the phase angle through which the reference wave has travelled before zero phase is reached on the variable wave. In this example, starting at zero phase on the reference wave (point A), we observe that the reference wave has travelled through a phase angle of 270° before zero phase is reached on the variable wave (point B), hence the phase difference is 270°. The relationship can also be found mathematically. At the origin the phase of the reference wave is 0° (= 360°) and the phase of the variable wave is 090°. Subtracting the instantaneous phase of the variable wave from the instantaneous phase of the reference wave gives the same result, note the result must always be positive. Reference – variable = 360° – 90° = 270° Note: The phase difference must be positive, so if the calculation yields a negative result simply add 360° to get a positive answer. 9 1 Properties of Radio Waves 1 Properties of Radio Waves 10 Questions 1 Practice Frequency (f) - Wavelength (λ) Conversions 1 Questions In each of the following examples, calculate the frequency or wavelength as appropriate and determine in which frequency band each of the frequencies lies. Wavelength Frequency Frequency Band 1 198 kHz 2 2.7 m 3 5.025 GHz 4 137.5 m 5 137.5 MHz 6 3 km 7 329 MHz 8 29 cm 9 500 kHz 10 5 cm 11 1 Answers Answers to Practice Frequency (f) - Wavelength (λ) Conversions 1 Answers Wavelength Frequency Frequency Band 1 1515 m 198 kHz LF 2 2.7 m 111.1 MHz VHF 3 5.97 cm 5.025 GHz SHF 4 137.5 m 2181.8 kHz MF 5 2.18 m 137.5 MHz VHF 6 3 km 100 kHz LF 7 91.2 cm 329 MHz UHF 8 29 cm 1034 MHz UHF 9 600 m 500 kHz MF 10 5 cm 6 GHz SHF 12 Questions 1 Questions 1 1. A radio wave is: Questions a. an energy wave comprising an electrical field in the same plane as a magnetic field b. an electrical field alternating with a magnetic field c. an energy wave where there is an electrical field perpendicular to a magnetic field d. an energy field with an electrical component 2. The speed of radio waves is: a. 300 km per second b. 300 million metres per second c. 162 NM per second d. 162 million NM per second 3. The plane of polarization of an electromagnetic wave is: a. the plane of the magnetic field b. the plane of the electrical field c. the plane of the electrical or magnetic field dependent on the plane of the aerial d. none of the above 4. If the wavelength of a radio wave is 3.75 metres, the frequency is: a. 80 kHz b. 8 MHz c. 80 MHz d. 800 kHz 5. The wavelength corresponding to a frequency of 125 MHz is: a. 2.4 m b. 24 m c. 24 cm d. 24 mm 6. The frequency which corresponds to a wavelength of 6.98 cm is: a. 4298 GHz b. 4.298 GHz c. 429.8 GHz d. 42.98 GHz 7. The frequency band containing the frequency corresponding to 29.1 cm is: a. HF b. VHF c. SHF d. UHF 13 1 Questions 8. To carry out a phase comparison between two electromagnetic waves: 1 a. both waves must have the same amplitude Questions b. both waves must have the same frequency c. both waves must have the same amplitude and frequency d. both waves must have the same phase 9. The phase of the reference wave is 110° as the phase of the variable wave is 315°. What is the phase difference? a. 205° b. 025° c. 155° d. 335° 10. Determine the approximate phase difference between the reference wave and the variable wave: (The reference wave is the solid line and the variable wave is the dashed line) a. 045° b. 135° c. 225° d. 315° 11. The wavelength corresponding to a frequency of 15 625 MHz is: a. 1.92 m b. 19.2 m c. 1.92 cm d. 19.2 cm 12. Which frequency band is a wavelength of 1200 m? a. UHF b. LF c. HF d. MF 14 Questions 1 1 Questions 15 1 Answers Answers 1 Answers 1 2 3 4 5 6 7 8 9 10 11 12 c b b c a b d b c c c b 16 Chapter 2 Radio Propagation Theory Introduction............................................. 19 Factors Affecting Propagation.................................... 19 Propagation Paths.......................................... 21 Non-ionospheric Propagation.................................... 21 Ionospheric Propagation....................................... 24 Sky Wave............................................... 27 HF Communications......................................... 32 Propagation Summary........................................ 34 Super-refraction........................................... 35 Sub-refraction............................................ 35 Questions............................................... 37 Answers................................................ 40 17 2 Radio Propagation Theory 2 Radio Propagation Theory 18 Radio Propagation Theory 2 Introduction In the context of radio waves the term propagation simply means how the radio waves travel 2 through the atmosphere. Different frequency bands use different propagation paths through Radio Propagation Theory the atmosphere; the propagation path often determines the uses to which a particular frequency band can be put in either communication or navigation systems. The different propagation paths associated with particular frequencies can also impose limitations on the use of those frequencies. Factors Affecting Propagation There are several factors which affect the propagation of radio waves and need to be considered when discussing the propagation paths: Attenuation Attenuation is the term given to the loss of signal strength in a radio wave as it travels outward from the transmitter. There are two aspects to attenuation: Absorption As the radio wave travels outwards from a transmitter the energy is absorbed and scattered by the molecules of air and water vapour, dust particles, water droplets, vegetation, the surface of the earth and the ionosphere. The effect of this absorption, (except ionospheric) increases as frequency increases and is a very significant factor above about 1000 MHz. Inverse Square Law The EM radiation from an aerial spreads out as the surface of a sphere so the power available decreases with increasing distance from the transmitter. For example, if, at a certain distance from a transmitter, the field intensity is 4 Wm-2 at double the distance that energy will be spread over an area of 4 m2 and the field intensity will be 1 Wm-2. That is, power available is proportional to the inverse of the square of the range. 1m 1W 1W 4W 1 m 2m 1W 1W 2m R 2×R Figure 2.1 Inverse Square Law 19 2 Radio Propagation Theory 1 P ∝ R2 2 The practical effect of this is that if it is required to double the effective range of a transmitter Radio Propagation Theory then the power would have to be increased by a factor of 4. Static Interference There is a large amount of static electricity generated in the atmosphere by weather, human activity and geological activity. The effect of static interference is greater at lower frequencies whereas at VHF and above the effect of interference is generally negligible. However, radio waves travelling through the ionosphere will collect interference at all frequencies. Additionally the circuitry in the receivers and transmitters also produces static interference. The static, from whatever source, reduces the clarity of communications and the accuracy of navigation systems. The strength of the required signal compared to the amount of interference is expressed as a signal to noise ratio (S/N) and for the best clarity or accuracy the unwanted noise needs to be reduced to the lowest possible levels. Fading Transmissions following different paths can occur for a number of reasons, e.g. reflections, and can arrive at a receiver simultaneously; however, the two signals will not necessarily be in phase. In extreme cases the two signals will be in anti-phase and will cancel each other out. Signals going in and out of phase are indicated by alternate fading and strengthening of the received signal. Power An increase in the power output of a transmitter will increase the range, within the limits of the inverse square law. As noted above, to double the range of a radio transmitter would require the power to be increased by a factor of 4. Receiver Sensitivity If internal noise in a receiver can be reduced then the receiver will be able to process weaker signals hence increasing the effective range at which a useable signal can be received. However, this is an expensive process. Directivity If the power output is concentrated into a narrow beam then there will be an increase in range, or a reduction in power required for a given range. However the signal will only be usable in the direction of the beam. 20 Radio Propagation Theory 2 Propagation Paths There are four propagation paths of which four need to be considered for aviation purposes: 2 Radio Propagation Theory PROPAGATION NON-IONOSPHERIC IONOSPHERIC Surface Wave Sky wave 20 kHz-50 MHz 20 kHz-50 MHz (Used 20 kHz-2 MHz) (Used 2-30 MHz) Space Wave SatComm > 50 MHz Direct Wave (UHF, SHF) Figure 2.2 Ionospheric propagation is propagation affected by the properties of the ionosphere. At this stage it is only necessary to discuss sky wave, satellite propagation will be considered in conjunction with global navigation satellite systems (GNSS) in Chapter 18. Knowledge of propagation below 30 kHz is not required. Non-ionospheric propagation covers the other propagation paths. Non-ionospheric Propagation Surface Wave Surface wave propagation exists at frequencies from about 20 kHz to about 50 MHz (from the upper end of VLF to the lower end of VHF). The portion of the wave in contact with the surface of the earth is retarded causing the wave to bend round the surface of the earth; a process known as diffraction. Figure 1.7. Figure 2.3 Surface Wave. Surface Wave 21 2 Radio Propagation Theory The range achievable is dependent on several factors: the frequency, the surface over which the wave is travelling and the polarization of the wave. As the frequency increases, surface attenuation increases and the surface wave range decreases; it is effectively non-existent above 2 HF. Radio Propagation Theory The losses to attenuation by the surface of the earth are greater over land than over sea, because the sea has good electrical conductivity. Hence greater ranges are attainable over the sea. A horizontally polarized wave will be attenuated very quickly and give very short ranges; therefore, vertical polarization is generally used at these lower frequencies. 10 k LAND SEA 100 k f (Hz) 1M 10 M 100 M 1 10 100 1000 10 000 NM Figure 2.4 This is the primary propagation path used in the LF frequency band and the lower part of the MF frequency band (i.e. frequencies of 30 kHz to 2 MHz). An approximation to the useable range achievable over sea and land for an MF transmission at a frequency of 300 kHz is given by: Sea: range ≈ 3 × √Power Land: range ≈ 2 × √Power So, for example, a 300 kHz transmitter with a power output of 10 kW would give a surface wave range of about 300 NM over the sea and 200 NM over the land. 22 Radio Propagation Theory 2 Space Wave The space wave is made up of two paths, a direct wave and a reflected wave. 2 Radio Propagation Theory Figure 2.5 Space wave At frequencies of VHF and above radio waves start to behave more like visible light and as we have a visual horizon with light we have a radio horizon with the radio frequencies. So the only atmospheric propagation at these frequencies is line of sight. RX TX Figure 2.6 Maximum theoretical range There is some atmospheric refraction which causes the radio waves to bend towards the surface of the earth increasing the range slightly beyond the geometric horizon. Since the diameter of the earth is known and the atmospheric refraction can be calculated it is possible to determine the maximum theoretical range at which a transmission can be received. The amount of refraction decreases as frequency increases but for practical purposes for the EASA syllabus the line of sight range can be calculated using the formula: Range (NM) = 1.23 × (√hTX + √hRX) hTX : Transmitter height in feet hRX : Receiver height in feet At VHF and above it does not matter how powerful the transmitter is, if the receiver is below the line of sight range, it will receive nothing. 23 2 Radio Propagation Theory For example: What is the maximum range a receiver at 1600 ft can receive VHF transmissions from a 2 transmitter at 1024 ft? Radio Propagation Theory Range = 1.23 × (√1600 + √1024) = 1.23 × (40 + 32) = 88.6 NM Note 1: R egardless of the possible propagation paths, if a receiver is in line of sight with a transmitter, then the space wave will be received. Ionospheric Propagation Before studying ionospheric propagation it is necessary to know about the processes which produce the ionization in the upper atmosphere and the properties of the ionosphere that produce sky wave. The Ionosphere The ionosphere extends upwards from an altitude of about 60 km to limits of the atmosphere (notionally 1500 km). In this part of the atmosphere the pressures are very low (at 60 km the atmospheric pressure is 0.22 hPa) and hence the gaseous atoms are widely dispersed. Within this region incoming solar radiation at ultra-violet and shorter wavelengths interacts with the atoms raising their energy levels and causing electrons to be ejected from the shells of the atoms. Since an atom is electrically neutral, the result is negatively charged electrons and positively charged particles known as ions. The electrons are continually attempting to reunite with the ions, so the highest levels of ionization will be found shortly after midday (about 1400) local time, when there is a balance between the ionization and the decay of the ionization with the electrons rejoining the ions and the lowest just before sunrise (at the surface). In summer the ionization levels will be higher than in winter, and ionization levels will increase as latitude decreases, again because of the increased intensity of the solar radiation. Increased radiation from solar flares is unpredictable but can give rise to exceptionally high levels of ionization, which in turn can cause severe disruption of communication and navigation systems, particularly those which are space based. It is not unusual for communication (and other) satellites to be shut down during periods of intense solar flare activity to avoid damage. As the incoming solar energy is absorbed by the gaseous atoms the amount of energy available to ionize the atoms at lower levels reduces and hence the levels of ionization increase with increase in altitude. However, because the normal atmospheric mixing processes associated with the lower levels of the atmosphere are absent in the higher levels, gravitation and terrestrial magnetism affect the distribution of gases. This means that the increase in ionization is not linear but the ionized particles form into discrete layers. 24 Radio Propagation Theory 2 F LAYER 2 Radio Propagation Theory E LAYER Km D LAYER e‐ Figure 2.7 Effect of ionisation with height The ionization is most intense at the centre of the layers decreasing towards the lower and upper edges of the layers. The characteristics of these layers vary with the levels of ionization. The lowest of these layers occurs at an average altitude of 75 km and is known as the D-region or D-layer. This is a fairly diffuse area which, for practical purposes, forms at sunrise and disappears at sunset. The next layer, at an average altitude of 125 km, is present throughout the 24 hours and is known as the E-layer. The E-layer reduces in altitude at sunrise and increases in altitude after sunset. The final layer of significance is the F-layer at an average altitude of 225 km. The F-layer splits into two at sunrise and rejoins at sunset, the F1-layer reducing in altitude at sunrise and increasing in altitude after sunset. The behaviour of the F2-layer is dependent on time of year, in summer it increases in altitude and may reach altitudes in excess of 400 km and in winter it reduces in altitude. 25 2 Radio Propagation Theory 2 Radio Propagation Theory Figure 2.8 Layers of the ionosphere Although, overall the levels of ionization increase from sunrise to midday local time and then decrease until sunrise the following morning, the levels are continually fluctuating as the intensity of high energy radiation from the sun fluctuates. So it would be possible for the ionization levels to decrease temporarily during the morning, or increase temporarily during the afternoon. The structure of the ionosphere gives stable conditions by day and by night. Around dawn and dusk, however, the ionosphere is in a transitional state, which leads to what can best be described as electrical turbulence. The result is that around dawn and dusk, radio navigation and communication systems using the ionosphere are subject to excessive interference and disruption. 26 Radio Propagation Theory 2 Sky Wave The ionization levels in the layers increase towards the centre of the layer. This means that as a 2 radio wave transits a layer it encounters an increasing density of ions as it moves to the centre Radio Propagation Theory of the layer and decreasing density as it moves out of the layer. If the radio waves travel across the layer at right angles they will be retarded, but will maintain a straight path. However, if the waves penetrate the layer at an angle they will be refracted away from the normal as they enter, then back towards the normal as they exit the layer. Figure 2.9 Sky wave propagation - critical angle The amount of refraction experienced by the radio waves is dependent on both the frequency and the levels of ionization. If the radio wave refracts to the (earth) horizontal before it reaches the centre of the layer then it will continue to refract and will return to the surface of the earth as sky wave; this is total internal refraction at the layer. Starting from the vertical at the transmitter, with a frequency which penetrates the ionosphere, as the angle between the vertical and the radio wave increases, an angle will be reached where total internal refraction occurs and the wave returns to the surface. This is known as the first returning sky wave and the angle (measured from the vertical) at which this occurs is known as the critical angle. The distance from the transmitter to the point where the first returning sky wave appears at the surface is known as the skip distance. As sky waves occur in the LF, MF and HF frequency bands there will also be some surface wave present. From the point where the surface wave is totally attenuated to the point where the first returning sky wave appears there will be no detectable signal, this area is known as dead space. 27 2 Radio Propagation Theory 2 Radio Propagation Theory Figure 2.10 Sky wave propagation - dead space The height at which full internal refraction occurs is dependent on frequency, but, as a generalization frequencies up to 2 MHz will be refracted at the E-layer and from 2 – 50 MHz at the F-layers. Sky wave is only likely to occur above 50 MHz when there are abnormal ionospheric conditions associated with intense sunspot or solar flare activity, therefore, VHF frequencies used for navigation systems do not produce sky waves. Effect of Change in Ionization Intensity Since the reason for the refraction is the ionization of the upper atmosphere it follows that if ionization intensity changes, then the amount of refraction of radio waves will also change. At a given frequency, as ionization increases the refractive index and hence the amount of refraction affecting the radio waves will also increase. This means that refraction will take place at a smaller critical angle and the skip distance and dead space will decrease. Conversely, a decrease in ionization will result in an increase in critical angle, skip distance and dead space. 28 Radio Propagation Theory 2 2 Radio Propagation Theory HIGH IONIZATION LOW IONIZATION Figure 2.11 Sky wave propagation - effect of increased ionization Effect of Change of Frequency For a given ionization intensity, the amount of refraction of radio waves decreases as frequency increases, because as frequency increases the energy contained in the radio wave increases and therefore refraction decreases. So, as frequency increases, the critical angle will increase and the skip distance and dead space will also increase. As frequency increases, the surface wave range will decrease, so there is an increase in dead space caused by both the increase in skip distance and decrease in surface wave range. Conversely, a decrease in frequency will give a decrease in critical angle, skip distance and dead space. Height of the Layers The skip distance will also be affected by the altitude of the refracting layers. As the altitude of the layer increases then the skip distance will also increase and greater ranges will be experienced by refraction at the F-layer than the E-layer. 29 2 Radio Propagation Theory LF and MF Sky Wave Propagation During the day the D-layer absorbs radio energy at frequencies below about 2 MHz (LF and MF bands). At night the D-layer is effectively non-existent so, at these frequencies, sky waves, 2 refracted at the E-layer are present. This means the sky waves at LF and MF are not reliable for Radio Propagation Theory continuous long range use and the presence of sky waves at night at the relatively short ranges associated with these lower frequencies will cause interference with short range navigation (and broadcasting) systems relying on surface wave reception. This affects ADF and will be discussed in more detail in Chapter 7. E LAYER D LAYER Sky Wave Surface Wave EARTH DAY E LAYER EARTH NIGHT Figure 2.12 LF/MF Sky wave propagation 30 Radio Propagation Theory 2 Achievable Ranges The maximum range for sky wave will be achieved when the path of the radio wave is tangential at the surface of the earth at both the transmitter and receiver. 2 Radio Propagation Theory A simple calculation shows that the average maximum range for refraction from the E-layer at 125 km is 1350 NM, and the average maximum range from the F-layer at 225 km is 2200 NM. These ranges will obviously change as the height of the ionized layers changes. Multi-hop sky wave occurs when the wave is refracted at the ionosphere then the sky wave is reflected back from the surface of the earth to the ionosphere etc. Multi-hop sky wave can achieve ranges of half the diameter of the earth. Figure 2.13 Multi-hop Sky wave propagation 31 2 Radio Propagation Theory HF Communications Over inhabited land areas VHF communications are ideal for all communications between 2 aircraft and ground. However, over oceans and uninhabited land areas, long range systems Radio Propagation Theory are required. Satellite Communications (SatCom) are not yet the norm, so long range communication must be provided by surface wave or sky wave propagation. To achieve ranges of 2000 - 3000 NM using surface wave propagation would require low frequencies either from the lower end of LF band or the upper end of VLF band. Communication systems utilizing these frequencies would require relatively complex equipment with an associated weight penalty. Lower frequencies are also subject to greater static interference than higher frequencies, making such systems somewhat tedious to use. Furthermore, data rates associated with low frequencies are notoriously low. Currently, therefore, the only practical solution is HF Communications utilizing sky wave propagation. In the future, no doubt, SatCom will become commonplace. Figure 2.14 The maximum usable frequency (MUF) for a given range will be that of the first returning sky wave and this is the ideal frequency for that range because it will have had the shortest path through the ionosphere, and therefore, will have experienced less attenuation and contain less static interference. However, since the ionization intensity fluctuates, a decrease in ionization would result in an increase in skip distance and hence loss of signal. So a compromise frequency is used, known as the optimum working frequency (OWF), which by decades of experimentation and experience has been determined to be 0.85 times the MUF. Since ionization levels are lower by night than by day it follows that the frequency required for use at a particular range by night will of necessity be less than the frequency required for use by day. A good rule of thumb is that the frequency required at night is roughly half that required by day. 32 Radio Propagation Theory 2 Because skip distance increases as frequency increases, the range at which communication is required will also influence the selection of the frequency to be used. Short ranges will require lower frequencies and longer ranges will require higher frequency. 2 A typical example of the sort of problem that may appear is: Radio Propagation Theory An aircraft on a flight from London, UK to New York, USA is in mid-Atlantic at sunrise. The pilot is in communication with the UK on a frequency of 12 MHz. What frequency can the pilot expect to use with the USA? (See Figure 2.16). Figure 2.15 HF Communications Mid-Atlantic Answer: 6 MHz. The wave will be refracted halfway between the aircraft and the UK, and halfway between the aircraft and the USA. Midway between the aircraft and the UK it is day, so a relatively high frequency will be required. Midway between the aircraft and the USA it is night so a relatively low frequency will be required. 33 2 Radio Propagation Theory Propagation Summary The propagation characteristics of each of the frequency bands are summarized below, where 2 propagation paths are in brackets this indicates that the path is present but not normally Radio Propagation Theory utilised. Frequency Band Propagation Path Surface Wave LF (Sky Wave) Surface Wave MF (Sky Wave) Sky Wave HF (Surface Wave) VHF Space Wave UHF Space Wave SHF Space Wave EHF Space Wave Figure 2.16 34 Radio Propagation Theory 2 Super-refraction This is a phenomenon which is significant at frequencies above 30 MHz (that is VHF and 2 above). Radio waves experience greater refraction, that is, they are bent downwards towards Radio Propagation Theory the earth’s surface more than in normal conditions, giving notable increases in line of sight range to as much as 40% above the usual. The conditions which give rise to super-refraction are: Decrease in relative humidity with height Temperature falling more slowly with height than standard Fine weather and high pressure systems Warm air flowing over a cooler surface In extreme cases when there is a low level temperature inversion with a marked decrease in humidity with increasing height (simply, warm dry air above cool moist air), a low level duct may be formed which traps radio waves at frequencies above 30 MHz giving extremely long ranges. This phenomenon is known as duct propagation and can lead to exceptionally long ranges. When interference is experienced on UK television channels from continental stations, the reason for this is the forming of such a duct. This phenomenon is most common where warm desert areas are bordering oceanic areas, e.g. the Mediterranean and Caribbean seas. It can also occur in temperate latitudes when high pressure predominates, particularly in the winter months when the dry descending air in the high pressure system is heated by the adiabatic process and is warmer than the underlying cool and moist air. Sub-refraction Much rarer than super-refraction, but still of significance in radio propagation, sub-refraction causes a reduction in the normal refraction giving a decrease in line of sight range by up to 20%. The conditions which give rise to sub-refraction are: An increase in relative humidity with increasing height Temperature decreasing with increasing height at a greater rate than standard Poor weather with low pressure systems Cold air flowing over a warm surface 35 2 Radio Propagation Theory 2 Radio Propagation Theory 36 Questions 2 Questions 1. The process which causes the reduction in signal strength as range from a transmitter 2 increases is known as: Questions a. absorption b. diffraction c. attenuation d. ionisation 2. Which of the following will give the greatest surface wave range? a. 243 MHz b. 500 kHz c. 2182 khz d. 15 MHz 3. It is intended to increase the range of a VHF transmitter from 50 NM to 100 NM. This will be achieved by increasing the power output by a factor of: a. 2 b. 8 c. 16 d. 4 4. The maximum range an aircraft at 2500 ft can communicate with a VHF station at 196 ft is: a. 79 NM b. 64 NM c. 52 NM d. 51 NM 5. What is the minimum height for an aircraft at a range of 200 NM to be detected by a radar at 1700 ft AMSL? a. 25 500 ft b. 15 000 ft c. 40 000 ft d. 57 500 ft 6. Determine which of the following statements concerning atmospheric ionization are correct: 1. The highest levels of ionization will be experienced in low latitudes 2. Ionization levels increase linearly with increasing altitude 3. The lowest levels of ionization occur about midnight 4. The E-layer is higher by night than by day because the ionization levels are lower at night a. statements 1, 2 and 3 are correct b. statements 1, 3 and 4 are correct c. statements 2 and 4 are correct d. statements 1 and 4 are correct 37 2 Questions 7. The average height of the E-layer is …… and the maximum range for sky wave will be …… 2 a. 60 km, 1350 NM b. 125 km, 2200 km Questions c. 225 km, 2200 km d. 125 km, 1350 NM 8. Concerning HF communications, which of the following is correct? a. The frequency required in low latitudes is less than the frequency required in high latitudes b. At night a higher frequency is required than by day c. The frequency required is dependent on time of day but not the season d. The frequency required for short ranges will be less than the frequency required for long ranges 38 Questions 2 2 Questions 39 2 Answers Answers 2 1 2 3 4 5 6 7 8 c b d a b d d d Answers 40 Chapter 3 Modulation Introduction............................................. 43 Keyed Modulation.......................................... 43 Amplitude Modulation (AM).................................... 44 Single Sideband (SSB)........................................ 45 Frequency Modulation (FM)..................................... 46 Phase Modulation.......................................... 47 Pulse Modulation........................................... 47 Emission Designators......................................... 47 Questions............................................... 50 Answers................................................ 52 41 3 Modulation 3 Modulation 42 Modulation 3 Introduction Modulation is the name given to the process of adding information to a radio wave or the formatting of radio waves for other purposes. Of the main forms of modulation, five have 3 application in aviation: Modulation Keyed Modulation Amplitude Modulation (AM) Frequency Modulation (FM) Phase Modulation Pulse Modulation The modulation of a radio frequency is generally associated with the transmission of audio information, although the transmission of data, including that in satellite navigation systems, and the determination of bearing in VOR, for example, require modulation for other purposes. Before an audio signal can be added to a radio wave it must be converted to an electrical signal. This will be achieved by the use of a microphone, which is quite simply a device that converts sound waves to an electrical current. It will be assumed for AM and FM that this conversion has already been accomplished. Keyed Modulation The simplest way to put information onto a carrier wave is to quite simply interrupt the wave to give short and long bursts of energy. ‘K’ Figure 3.1 Morse ‘K’ in keyed modulation By arranging the transmissions into short and long periods of carrier wave transmission we can send information using the Morse code. This is known as telegraphy and until the development of other forms of modulation was the only means of passing information. Keyed modulation is still used by some non-directional beacons (NDBs) for identification and will be discussed further in Chapter 7. 43 3 Modulation Amplitude Modulation (AM) In AM the amplitude of the audio frequency (AF) modifies the amplitude of the radio frequency (RF). 3 Modulation Figure 3.2 Amplitude modulation As can be seen from the diagram above, positive amplitude in the AF gives an increase in amplitude in the RF and negative amplitude in the AF gives a decrease in amplitude in the RF. The process of combining a radio frequency with a current at audio frequencies is known as heterodyning. Looking in more detail at the process; the heterodyning process combines the two frequencies, leaving the RF unchanged but producing new frequencies at the sum and difference of the RF and AF. For example an audio frequency of 3 kHz is used to amplitude modulate a radio frequency of 2182 kHz. The RF remains unchanged but the AF is now split into 2 sidebands extending upwards from 2182.001 kHz to 2185 kHz – the upper sideband (USB) and a lower sideband (LSB) extending downwards from 2181.999 kHz to 2179 kHz. The spread of frequencies is from 2179 kHz to 2185 kHz giving a bandwidth of 6 kHz, i.e. double the audio frequency used. 2185 kHz (25 W) ⇧ Upper Sideband (USB) (100 W) RF 2182 kHz 2182.001 kHz ⇧ (100 W) 2182 kHz (50 W) AF 3 kHz 2181.999 kHz (25 W) ⇧ Lower Sideband (LSB) 2179 kHz Figure 3.3 AM sideband production 44 Modulation 3 As can be seen from the table the power that is in the AF is divided equally between the two sidebands, furthermore the information in the AF is contained in both sidebands. It should also be noted that only one third of the signal is carrying the information. Single Sideband (SSB) 3 Modulation There is redundancy in double sideband transmissions in that the information is contained in both the upper and lower sidebands. Additionally, the original RF carrier wave having served its purpose to get the audio information into radio frequencies is now redundant. So it is possible to remove one of the sidebands and the carrier wave because the remaining sideband contains all the information. This is known as single sideband (SSB) operation. 2185 kHz (25 W) (150 W) ⇧ Upper Sideband (USB) (100 W) RF 2182 kHz 2182.001 kHz ⇧ (100 W) 2182 kHz (50 W) AF 3 kHz 2181.999 kHz (25 W) ⇧ Lower Sideband (LSB) 2179 kHz Figure 3.4 Single sideband When using sky wave propagation for communication, the differing refraction occurring at different frequencies leads to an increase in distortion if the bandwidth is too large. The ionosphere comprises electrically charged particles which cause high levels of static interference on radio waves, the use of SSB significantly reduces the effect of this interference. The MF & HF frequencies used for long range communication are in great demand, hence the use of SSB transmissions increases the number of channels available. The use of SSB also reduces the amount of power required. Thus the main advantages of SSB are: Double the number of channels available with double sideband Better signal/noise ratio (less interference) Less power required hence lighter equipment 45 3 Modulation Frequency Modulation (FM) In Frequency Modulation, the amplitude of the audio frequency modifies the frequency of the carrier wave. 3 Modulation Figure 3.5 Frequency modulation The change in the carrier wave frequency is dependent on the rise and fall of the amplitude of the modulating wave/audio frequency: the greater the amplitude, the greater the frequency deviation. The frequency of the modulating wave determines the rate of change of frequency within the modulated carrier wave. When FM is used for sound broadcasting (for example, music radio stations), the bandwidth permitted by international agreements is 150 kHz, compared to 9 kHz allowed for AM. In general, therefore, FM is unsuitable for use on frequencies below VHF. For voice communications the bandwidth can be considerably reduced whilst still maintaining the integrity of the information; this is known as Narrow Band FM (NBFM). Typically, NBFM systems have a bandwidth of 8 kHz, which is greater than the 6 kHz permitted for Aeronautical Communications and the 3 kHz used in HF Communications; therefore, NBFM communication systems are not yet used in aviation. 46 Modulation 3 Phase Modulation In phase modulation the phase of the carrier wave is modified by the input signal. There are two cases: the first is where the input is an analogue signal when the phase of the carrier wave 3 is modified by the amplitude of the signal; secondly, with a digital signal it is known as phase shift keying, the phase change reflects a 0 or 1; e.g. 0° phase shift indicates a zero and 180° Modulation phase shift represents a 1. (Note: this is the simplest case as multiple data can be represented by using many degrees of phase shift.) There are two cases used in navigation systems, MLS and GPS. GPS uses binary phase shift keying, MLS uses differential phase shift keying. Amplitude Modulation Frequency Modulation Phase Modulation Figure 3.6 Pulse Modulation Pulse modulation is used extensively in radar systems and for data exchange in communications systems. An intermittent carrier wave is formed by the generation and transmission of a sequence of short period pulses. Emission Designators In order to easily identify the characteristics and information provided by electronic signals, a list of designators has been devised. They comprise 3 alphanumerics, where the first letter defines the nature of the modulation, the second digit the nature of the signal used for the modulation and the third letter the type of information carried. 47 Modulation 3 3 48 EMISSION CHARACTERISTICS First Symbol Second Symbol Third Symbol Type of modulation of the main carrier Nature of signals modulating the main carrier Type of information transmitted Modulation Emissions of an unmodulated N 0 No modulating signal N No information transmitted carrier Single channel containing quantized or Amplitude modulation - Double digital information without the use of a A 1 A Telegraphy for aural reception sideband modulating sub-carrier, excluding time division multiplex Single channel containing quantized Amplitude modulation - Single or digital information with the use of a Telegraphy for automatic H 2 B sideband, full carrier modulating sub-carrier, excluding time reception division multiplex Amplitude modulation - Single Single channel containing analogue J 3 C Facsimile sideband – suppressed carrier information Data transmission, telemetry, D telecommand Two or more channels containing Telephony, including sound F Frequency modulation 7 E quantized or digital information broadcasting Two or more channels containing G Phase modulation 8 F Television (video) analogue information Composite system with one or more channels containing quantized or 9 digital information, together with one W Combinations of the above or more channels containing analogue information P Sequence of unmodulated pulses Sequence of pulses modulated in K X Cases not otherwise covered X Cases not otherwise covered amplitude Modulation 3 For example, VHF radio telephony communications have the designation A3E. Reference to the table gives the following breakdown: A - Amplitude modulation - Double sideband 3 3 - Single channel containing analogue information Modulation E - Telephony, including sound broadcasting This means an RF carrier wave is being amplitude modulated with speech. HF radio telephony communications have the designation J3E, this gives: J - Amplitude modulation – single sideband with suppressed carrier 3 - Single channel containing analogue information E - Telephony, including sound broadcasting This means an RF carrier wave is being amplitude modulated with speech then the RF carrier wave is being removed along with one of the sidebands. It is not necessary to know the details of the table. Other designators relevant to the equipments discussed in phase 2 are: ADF N0NA1A or N0NA2A VHF RTF A3E HF RTF J3E VOR A9W ILS A8W Marker Beacons A2A DME P0N MLS N0XG1D With the exception of ADF it is unlikely that knowledge of these designators will be examined. 49 3 Questions Questions 1. The bandwidth produced when a radio frequency (RF) of 4716 kHz is amplitude modulated with an audio frequency (AF) of 6 kHz is: 3 a. 6 kHz Questions b. 3 kHz c. 12 kHz d. 9 kHz 2. Which of the following statements concerning AM is correct? a. The amplitude of the RF is modified by the frequency of the AF b. The amplitude of the RF is modified by the amplitude of the AF c. The frequency of the RF is modified by the frequency of the AF d. The frequency of the RF is modified by the amplitude of the AF 3. Which of the following is an advantage of single sideband (SSB) emissions? a. More frequencies available b. Reduced power requirement c. Better signal/noise ratio d. All of the above 4. Which of the following statements concerning FM is correct? a. The amplitude of the RF is modified by the frequency of the AF b. The amplitude of the RF is modified by the amplitude of the AF c. The frequency of the RF is modified by the frequency of the AF d. The frequency of the RF is modified by the amplitude of the AF 50 Questions 3 3 Questions 51 3 Answers Answers 1 2 3 4 c b d d 3 Answers 52 Chapter 4 Antennae Introduction............................................. 55 Basic Principles............................................ 55 Aerial Feeders............................................ 56 Polar Diagrams............................................ 57 Directivity............................................... 58 Radar Aerials............................................. 60 Modern Radar Antennae...................................... 61 Questions............................................... 63 Answers................................................ 64 53 4 Antennae 4 Antennae 54 Antennae 4 Introduction Antennae or aerials are the means by which radio energy is radiated and received. The type of antenna used will be determined by the function the radio system is required to perform. This chapter will look at the principles which are common to all antennae and at the specialities required for particular radio navigation systems. 4 Basic Principles Antennae There are two basic types of aerial used for receiving and transmitting basic communications, the half-wave dipole and the Marconi or quarter-wave aerial. V λ 4 I λ 4 Figure 4.1: Half-wave dipole With the dipole aerial the power is fed to the centre of the aerial and radiates in all directions perpendicular to the aerial. The Marconi aerial is set on, but insulated from, a metal surface which acts as the second part of a dipole, with the radio energy radiating perpendicular to the aerial. Because of the better aerodynamic qualities, Marconi aerials are used on aircraft. λ 4 Figure 4.2: Marconi aerial For an aerial to operate with maximum efficiency it must be the correct length for the wavelength of the frequency in use. As the names imply the ide