How Cells Communicate PDF
Document Details
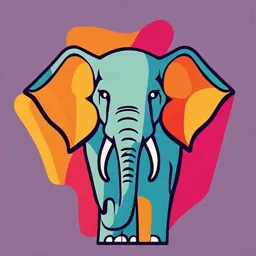
Uploaded by BestSellingAnemone
Tags
Summary
This document explains the methods of cell communication. It details how cells respond to external signals, including chemical signals, light, taste, and smell. It covers topics such as local signals, like paracrine and synaptic signaling, and long-distance communication, including endocrine signaling.
Full Transcript
How cells communicate Describe the overall method of cell signalling cellular Responsiveness: ○ Cells are versatile actors—they respond both individually and as part of a larger ensemble (like a tissue or organ). ○ When the conductor (external signals) raises the baton, cells listen attentively. Sig...
How cells communicate Describe the overall method of cell signalling cellular Responsiveness: ○ Cells are versatile actors—they respond both individually and as part of a larger ensemble (like a tissue or organ). ○ When the conductor (external signals) raises the baton, cells listen attentively. Signal Language: ○ These cues are often chemical signals—molecules that convey messages. ○ Imagine neurotransmitters, hormones, and growth factors as musical notes in this cellular score. ○ But don’t forget other signals: light (photoreceptors), taste, and smell (chemoreceptors). Cellular Orchestra: ○ Cells harmonise their responses: Neurons transmit electrical signals. Immune cells coordinate defence. Endocrine cells release hormones. Muscle cells contract in unison. Epithelial cells form protective barriers. Stem cells await their cues for differentiation. Feedback Loops: ○ Like a musical motif that repeats, cells adjust their actions based on feedback. ○ Negative feedback keeps the performance in balance. ○ Positive feedback amplifies responses (like applause after a breathtaking solo). Local Signals (Nearby Target Cells): ○ Paracrine Signalling: Growth factors, like fibroblast growth factor (FGF1), act locally. They’re secreted by one cell and affect nearby target cells. Think of it as a neighbourhood chat—cells communicate with their immediate neighbours. ○ Synaptic Signalling: Neurotransmitters, such as acetylcholine (ACh), zip across synapses. Neurons use this rapid communication to transmit signals to adjacent cells. It’s like a quick text message between close friends. ○ Autocrine Signalling: Cells respond to signals they themselves secrete. Imagine a cell talking to its own reflection in the mirror. Long-Distance Signals: ○ Endocrine Signalling: Hormones play the role of long-distance messengers. They’re secreted by endocrine cells (like pancreatic beta cells). Hormones travel via the circulatory system (blood) to reach distant target cells. For example, insulin secreted by pancreatic beta cells enters the bloodstream and affects various body cells. It’s like sending a letter to someone across the country. Local vs. Long Distance: ○ Local: Nearby cells chat over the fence. ○ Long Distance: Hormones embark on cross-country journeys. The overall method 1. Reception: ○ A signaling protein (the primary messenger) approaches the cell. ○ It binds to a receptor protein on the cell’s surface. ○ This interaction causes a change in the receptor’s shape or chemical state. ○ It’s like a secret handshake—the cell recognizes the signal. 2. Transduction: ○ The altered receptor activates another protein (like a G-protein or adenylyl cyclase). ○ This activated protein often acts as an enzyme, setting off a relay of changes. ○ Second messengers (like cAMP or IP₃) come into play. ○ Multiple proteins join the dance, creating a phosphorylation cascade. ○ It’s like a domino effect—the message spreads through the cell. 3. Response: ○ All the activated proteins collaborate to make things happen within the cell. ○ This is where the cell takes action: Enzymes may be activated or inhibited. Gene expression may change. Cellular processes (like metabolism or growth) kick into gear. Understanding the receptors 1. Chemical Signaling Precision: ○ The human body orchestrates a symphony of chemicals and molecules to evoke specific responses. ○ However, only target receptors interact with these signals (ligands) and activate signal transduction pathways. ○ Exquisite control ensures that only specific cells, at specific times, possess particular receptors—making the signal transmission precise and localised. 2. The Source of Specificity: ○ The 3D molecular shape of proteins plays a pivotal role. ○ Structure determines function: Each receptor acts like a lock, and only specific ligands fit into their binding sites. ○ This specificity allows our body to respond effectively while minimising unnecessary activation. 1) Describe, compare and contrast the two major cell surface receptors (G Protein coupled and ligand-gated ion channel receptors) Water-Soluble Molecules: Membrane-bound receptors (e.g., GPCRs, RTKs, ligand-gated ion channels). Lipid-Soluble Molecules: Not membrane-bound; located in cytoplasm or nucleus. GPCRs (G-protein-coupled receptors): Hundreds of different GPCRs exist, serving diverse functions such as development and sensory reception (vision, taste, smell). G Proteins: These molecular switches toggle between on (GTP-bound) and off (GDP-bound) states. They play a crucial role in cellular signalling. Clinical Significance: GPCRs are associated with many diseases and are targeted by one-third of modern drugs. Nobel prizes have recognized their impact. How are the G-protein coupled receptors (GRCRs)? 1. Resting State: ○ The receptor is unbound, and the G protein is bound to GDP (guanosine diphosphate). ○ The enzyme associated with the G protein remains inactive. 2. Ligand Binding: ○ When a ligand binds to the receptor, it also binds to the G protein. ○ GTP (guanosine triphosphate) displaces GDP, but the enzyme remains inactive. 3. Activation: ○ The activated G protein dissociates from the receptor. ○ The enzyme becomes active, initiating cellular responses. 4. GTPase Activity: ○ The G protein possesses GTPase activity, promoting its release from the enzyme. ○ The system reverts back to the resting state. 5. Conformational Changes: ○ Throughout this process, conformational changes in the receptor and G protein determine their function. ○ Although not always evident in cartoons, these shape alterations orchestrate cellular responses. Ligand-gated ion channels/receptors 1) Definition: These receptors are membrane proteins that contain a “gate.” The channel opens or closes as the receptor changes shape. 2) Activation: When a ligand (e.g., neurotransmitter) binds to a specific site on the receptor, it triggers a change in shape. 3) Components: ○ Receptor: A molecule or protein that responds to a specific ligand. ○ Ligand: A signalling molecule that binds specifically to another protein. ○ Ion Channel: A membrane protein through which specific ions (e.g., Na+, K+, Ca2+, Cl−) can travel. ○ Ion Channel Receptor (Ionotropic Receptor): A membrane protein that allows specific ions to pass through in response to ligand binding. How do the ligand-gated ion channels work? At Rest: ○ The ligand is unbound, and the gate of the ion channel is closed. Upon Ligand Binding: ○ ○ When a ligand (such as a neurotransmitter) binds to the receptor site on the ion channel, the gate opens. Specific ions (such as Na+, K+, or Ca2+) can flow into the cell. After Ligand Dissociation: ○ Once the ligand dissociates, the gate closes, returning to the resting state. Question: Which body system relies heavily on ligand-gated ion channels? Answer: The nervous system: Released neurotransmitters bind as ligands to ion channels on target cells, allowing the propagation of action potentials. 2) There are two ways that signal transduction process works Describe signal transduction via a phosphorylation cascade - transduction Phosphorylation Cascade: ○ Imagine a relay race where each runner (protein kinase) passes the baton (phosphate group) to the next. ○ In a phosphorylation cascade, a series of protein kinases sequentially add a phosphate group to the next kinase in line. ○ These kinases act like molecular switches, activating specific proteins upon phosphorylation. Protein Kinases and Phosphatases: ○ Protein kinases are enzymes that transfer a phosphate group from ATP to another protein. ○ This phosphorylation event often activates the target protein, triggering downstream effects. ○ Conversely, phosphatases remove the phosphate group, rendering the protein inactive but recyclable. Key Residues and Mutations: ○ Phosphorylation typically occurs at serine or threonine residues within proteins. ○ Mutations affecting these critical residues could have detrimental consequences Describe the role of the two main secondary messengers (cAMP and Ca2+) transduction Adenylyl Cyclase Activation: ○ In GPCR signalling, when a ligand binds to the receptor, it activates the associated G protein. ○ The activated G protein, specifically the Gα subunit, stimulates adenylyl cyclase. ○ Adenylyl cyclase converts ATP (adenosine triphosphate) into cAMP. cAMP as a Second Messenger: ○ cAMP acts as a second messenger, relaying the signal from the receptor to downstream proteins. ○ One key downstream player is PKA (protein kinase A). ○ PKA phosphorylates other proteins, influencing various cellular processes. Cholera Toxin Disruption: Cholera toxin interferes with this pathway by permanently activating adenylyl cyclase. ○ This leads to excessive cAMP production, causing severe diarrhoea in infected individuals. Other Second Messengers: ○ Besides cAMP, other small molecules, such as calcium ions, also participate in signalling cascades. ○ Calcium ions (Ca²⁺) and their crucial role in cellular homeostasis: 1. Intracellular Calcium Concentration: ○ Inside the cell, the concentration of calcium ions is typically low, around 100 nanomolar (nM). ○ However, outside the cell, the concentration of calcium ions is very high, more than 1000-fold higher. 2. Maintenance and Regulation: ○ Precise control of calcium levels is essential for cellular function. ○ Calcium pumps play a vital role in maintaining this balance: Out of the Cell: Calcium pumps actively transport calcium ions out of the cell. Into the Endoplasmic Reticulum (ER): The ER serves as an intracellular calcium reservoir. Into Mitochondria: Mitochondria also take up calcium ions, influencing energy production and other processes. Calcium Ions (Ca²⁺): ○ ○ ○ Calcium ions flow out of the endoplasmic reticulum (ER) down their concentration gradient. This increase in cytosolic calcium concentration activates various proteins, leading to cellular responses. One key player in this process is phospholipase C (PLC). Phospholipase C (PLC): ○ ○ PLC cleaves a phospholipid called PIP2 (phosphatidylinositol-4,5-bisphosphate) into two molecules: Diacylglycerol (DAG) remains in the inner layer of the plasma membrane. IP3 (inositol trisphosphate) diffuses through the cytosol and binds to a gated channel in the ER. IP3 triggers the release of calcium ions from the ER, further amplifying the signal. Muscle Contraction: ○ ○ Muscles use calcium ions (Ca²⁺) to contract. Refer to ET:M lectures for more details on this fascinating process. Why are there so many different cell signalings? 1. Amplification: ○ Multiple signalling pathways amplify the response. ○ A single initial signal can trigger a cascade of events, magnifying the effect. ○ Think of it as turning up the volume on a stereo—more pathways, louder response! 2. Control Points: ○ Diverse pathways provide multiple control points. ○ Cells can fine-tune their responses by adjusting different steps. ○ Imagine a complex dance where each move matters. 3. Specificity: ○ Different pathways allow for specific responses. ○ Temporal specificity: Some pathways activate only at certain times. ○ Spatial specificity: Specific cells or regions respond. ○ Despite common molecules, the context matters. 4. Coordination: ○ Pathways coordinate like synchronised dancers. ○ They interact, ensuring harmony. ○ Imagine a grand ballet with many performers 3) The transduction of a signal leads to the regulation of one or more cellular activities Cellular Responses include: Examples of a cellular response include activation or regulation of: Gene expression Alteration of protein function to gain or lose an activity Opening or closing of an ion channel Alteration of cellular metabolism Regulation of cellular organelles or organisation Rearrangement/movement of cytoskeleton A combination of any of these Give examples of cellular activities as outputs of cell signalling Temporal Regulation: Cellular signals are transient, ensuring homeostatic equilibrium. Caffeine and PDE: Caffeine blocks phosphodiesterases (PDEs), affecting cyclic nucleotide breakdown. Therapeutic Potential: Inhibiting specific PDEs (e.g., Viagra) can be therapeutic. Cellular Readiness: Feeling “weird” after coffee means cells are primed to respond if needed. Adrenaline and Glycogen Breakdown: ○ ○ ○ Adrenaline acts through a GPCR (G protein-coupled receptor). It activates cAMP (cyclic adenosine monophosphate) and triggers a phosphorylation cascade involving two protein kinases. The ultimate result is the activation of glycogen phosphorylase. Glycogen Phosphorylase: ○ ○ ○ Glycogen phosphorylase catalyses the release of glucose 1-phosphate from glycogen. Glycogen serves as a long-term energy store in the liver and skeletal muscle. Glucose 1-phosphate is then converted to glucose 6-phosphate, which can be used in glycolysis to generate ATP. Amplification: ○ ○ Remarkably, one adrenaline molecule can lead to the production of 10⁸ glucose 1-phosphate molecules! This impressive amplification ensures efficient energy mobilisation. Angiotensin-converting enzyme 2 (ACE2) serves as the cellular receptor for the coronavirus SARS-CoV-2. The virus’s surface spike glycoprotein (S protein) interacts with ACE2, allowing viral entry into our cells. Think of ACE2 as the lock, and the S-protein on the virus as the key. This critical interaction is central to COVID-19 infection. For a deeper dive into how COVID-19 mRNA vaccines work, you can explore this resource.