Organic Molecules CH3.pdf
Document Details
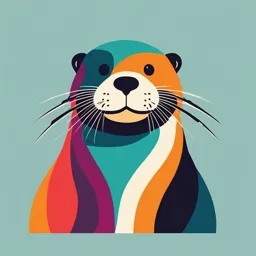
Uploaded by RightSunflower
The University of Texas at Dallas
Tags
Summary
This document provides an overview of organic molecules, including their structure, properties, and significance in living organisms. Sections cover fundamental concepts such as hydrocarbons, functional groups, isomers, and various types of organic molecules crucial for life processes.
Full Transcript
Why It Matters... Using energy from sunlight, trees and other plants combine water and carbon dioxide into sugars and other carbon-based compounds through the process of photosynthesis The lives of plants and almost all other organisms depend directly or indirectly on...
Why It Matters... Using energy from sunlight, trees and other plants combine water and carbon dioxide into sugars and other carbon-based compounds through the process of photosynthesis The lives of plants and almost all other organisms depend directly or indirectly on the productsof photosynthesis Carbon compounds form structures of living organisms, take part in all biological reactions, and serve as energy sources for living organisms and industry (ossil fuels) Organic Molecules Four major classes of organic molecules are found in living organisms: carbohydrates, lipids, proteins, and nucleic acids In organic molecules, carbon atoms bond covalently to each other and to other atoms in molecules that range in size from a few atoms to thousands or millions of atoms Molecules consisting of carbon linked only to hydrogen atoms are called hydrocarbons Hydrocarbons (1 of 2) Carbon has four unpaired outer electrons The simplest hydrocarbon, CH4(methane), consists of a single carbon atom bonded to four hydrogen atoms More complex hydrocarbons involve two or more carbon atoms arranged in a linear unbranched chain, a linear branched chain, or a structure with one or more rings Single and double bonds are found in linear and ring hydrocarbons; triple bonds only in two-carbon hydrocarbons Hydrocarbons (2 of 2) Chemical Evolution Chemical evolution resulted in the first forms of life on Earth after formation of organic molecules Resulted from reactions involving inorganic molecules on primordial Earth and conditions on the planet at that time Stanley Miller and Harold Urey performed a classic set of experiments in 1953 to simulation chemical evolution and formed several complex organic molecules Functional Groups Functional groups are small, reactive groups of atoms which give larger molecules specific chemical properties Functional groups that enter most frequently into biological reactions are the hydroxyl, carbonyl, carboxyl, amino, phosphate, and sulfhydrylgroups Functional groups are linked by covalent bonds to other atoms in biological molecules, usually carbon atoms –represented by the collective symbol R Hydroxyl and Carbonyl Groups Groups which can donate a “Hydrogen” ion in water, acts as an Acid. Example: Carboxyl, phosphate group. Groups which can accept a “Hydrogen” ion in water, acts as a Base. Example: Amino group. Phosphate, Sulfhydryl, and Methyl Groups Isomers Carbons that are linked to four different atoms or functional groups are asymmetric Can take either of two fixed positions with respect to other carbons in a chain The two forms (isomers) have the same chemical formula, but have different molecular structures Example: In glyceraldehyde, the —H and —OH groups can take either of two positions, left or right of the carbon chain Types of Isomers Isomers that are mirror images of each other (such as glyceraldehyde) are called stereoisomers Typically, only one of the two forms (L-form or D-form) can enter into a reaction Structural isomers are two molecules with the same chemical formula but atoms are arranged in different ways Example: glucose, an aldehyde and fructose, a ketone Stereoisomers (of an amino acid) Most of the enzymes that Most of the enzymes that catalyze the biochemical catalyze the biochemical reactions involving amino acids reactions involving sugars recognize the L-stereoisomer. recognize the d stereoisomer Lisomer (L forlaevus= left) D isomer (D fordexter= right) Structural Isomers (A)In glucose, the aldehyde isomer, the carbonyl group (shaded region) is located at the end of the carbon chain. (B)In fructose, the ketone isomer, the carbonyl group is located inside the carbon chain. For convenience, the carbons of the sugars are numbered, with 1 being the carbon at the end nearest the carbonyl group Adding and Removing Water In many reactions involving functional groups, the components of a water molecule (—H and —OH) are removed from or added to the groups as they interact When water components are removed, the reaction is called a dehydration synthesis reaction or condensation reaction When water components are added, the reaction is called hydrolysis Macromolecules (1 of 2) Carbohydrates, lipids, proteins, and nucleic acids are large polymers, assembled from subunit molecules (monomers) into a chain by covalent bonds Polymers are assembled from monomers (polymerization) by dehydration synthesis reactions The breakdown of polymers into monomers occurs by hydrolysis Macromolecules (2 of 2) Each type of polymeric biological molecule contains one type of monomer Individual monomers may be identical, or may have chemical variations, depending on the molecule A single polymer molecule with a mass of 1,000 daltons (Da) or more is called a macromolecule Includes many carbohydrates, proteins, and nucleic acids Lipids are not large enough to be classed as macromolecules 3.2 Carbohydrates Carbohydrates serve many functions Energy-providing carbohydrates are stored in plant cells as starch, and in animal cells as glycogen Structural carbohydrates include cellulose, a primary component of plant cell walls Carbohydrates contain only carbon, hydrogen, and oxygen atoms in a ratio of about 1C:2H:1O (CH2O) Monosaccharides contain three to seven carbon atoms Two monosaccharides polymerize to form a disaccharide Carbohydrate polymers with more than 10 linked monosaccharide monomers are polysaccharides Monosaccharides (1 of 2) Carbohydrates occur either as monosaccharides or as polymers of monosaccharide units Monosaccharides (such as glucose, C6H12O6) are soluble in water and sweet tasting The most common monosaccharides contain three carbons (trioses), five carbons (pentoses), or six carbons (hexoses) All monosaccharides can occur in linear form Monosaccharides (2 of 2) Ring Forms (1 of 2) Monosaccharides with five or more carbons (such as glucose) can fold back on themselves through a reaction btween two functional groups to assume a ring form Glucose exists as two different enantiomer α-glucose, with an —OH group pointing below the plane of the ring β-glucose, with an —OH group pointing above the plane Ring Forms (2 of 2) α β Disaccharides (1 of 2) Disaccharides are assembled from two monosaccharides covalently joined by a dehydration synthesis reaction Example: Maltose is formed by a glycosidic bondlinking two α- glucose molecules with oxygen as a bridge between the 1 carbon of the first glucose and the 4 carbon of the second glucose unit – an α (1→4) linkage Other common disaccharides include sucrose (glucose + fructose) and lactose (glucose + galactose) Disaccharides (2 of 2) A dehydration synthesis reaction involving two monosaccharides produces a disaccharide. The components of a water molecule (in blue) are removed from the monosaccharides as they join. Polysaccharides (1 of 5) The most common polysaccharides (plant starches, glycogen, and cellulose) are polymers of hundreds or thousands of glucose units Chitin is assembled from glucose units modified by the addition of nitrogen-containing groups Polysaccharides may be linear, unbrnched molecules, or they may contain one or more branches in which side chains of sugar units are attached to a main chain Polysaccharides (2 of 5) Ed Reschke/Photolibrary/Getty Images Polysaccharides (3 of 5) Mike Rosecope/Shutterstock.com Polysaccharides (4 of 5) Biophoto Associates/Science Source Polysaccharides (5 of 5) Carolina K. Smith MD/Shutterstock.com 3.3 Lipids Lipids are water-insoluble, primarily nonpolar biological molecules composed mostly of hydrocarbons Three common types of lipid molecules: Neutral lipids are stored and used as an energy source Phospholipids form cell membranes Steroids serve as hormones that regulate cellular activities Neutral Lipids Neutral lipids, found in cells as energy-storage molecules, have no charged groups (nonpolar) There are two types of neutral lipids: Oils are liquid at biological temperatures Fats are semisolid A fatty acid contains a single hydrocarbon chain with a carboxyl group (—COOH) at one end Glycerol and Triglyceride Formation Triglycerides form by dehydration synthesis between three- carbon glycerol (an alcohol) and three fatty acid side chains A covalent bond (ester linkage) forms between the -COOH group of the fatty acid and the -OH group of the glycerol The polar groups of glycerol are eliminated, forming a nonpolar triglyceride Triglycerides (A) Formation of a triglyceride by dehydration synthesis of glycerol with three fatty acids. The fatty acid shown is palmitic acid, and the triglyceride product is glyceryl palmitate. The components of a water molecule (in blue) are removed from the glycerol and fatty acids in each of the three bonds formed. (B) Spacefilling model of the triglyceride, glyceryl palmitate. Saturated and Unsaturated Fatty Acids (1 of 2) The most common fatty acids have chains of 14 to 22 carbons As chain length increases, fatty acids become less water-soluble and more oily A saturated fatty acid binds the maximum number of hydrogen atoms Only single bonds exist between carbon atoms Fatty acids with one double bond are monounsaturated; those with more than on double bond are polyunsaturated Saturated and Unsaturated Fatty Acids (2 of 2) Unsaturated fatty acids (such as vegetable oils) bend at a double bond (called “kink”) and are more fluid at biological temperatures Saturated fatty acids are found in solid animal fats such as butter Unsaturated fats are considered healthier than saturated fats in the human diet Plant oils are converted commercially to saturated fats by hydrogenation Fatty Acids (A)Stearic acid, a saturated fatty acid. kink (B) Oleic acid, an unsaturated fatty acid. An arrow marks the “kink” introduced by the double bond. Functions of Triglycerides Triglycerides serve as energy reserves in animals Store more than twice the calories per gram as carbohydrates A layer of fatty tissue just under the skin acts as insulation in mammals and birds (e.g., penguins) Triglycerides also help make bird feathers waterproof Focus on Research (1 of 2) Atherosclerosis occurs from the build up of plaques in the coronary arteries Plaques form from the buildup Micrograph Louis L. Lainey of excess cholesterol Waxes Fatty acids combine with long-chain alcohols or hydrocarbon structures to form waxes, which are harder ad less greasy than fats Waxy coatings help animals keep skin, hair, or feathers protected, lubricated, and pliable Plants secrete waxes that form a protective exterior layer, which reduces water loss and resists infective agents Phospholipids Phosphate-containing phospholipids are the primary lipids of cell membranes The most common phospholipid has a glycerol backbone linked to two fatty acid chains and a polar phosphate group, which is linked to another polar group The end of the molecule containing the fatty acids is nonpolar and hydrophobic, and the end with the phosphate group is polar and hydrophilic Phospholipid Bilayer In watery environments, only the polar ends of phospholipid molecules are exposed to water; their nonpolar ends collect together in a region that excludes water The phospholipid bilayer– a film of phospholipids two molecules thick – is the structural basis of membranes In a bilayer, the polar groups face the surrounding water molecules at the surfaces of the bilayer – the hydrocarbon chains form a nonpolar, hydrophobic region in the interior Phospholipid Structure (A) The arrangement of components in phospholipids. (B) Phosphatidyl ethanolamine, a comon membrane phospholipid. (C) Space-filling model of phosphatidyl ethanolamine. The kink in the fatty acid chain on the right reflects a double bond at this position. (D) Diagram widely used to depict a phospholipid molecule in cell membrane diagrams. The sphere represents the polar end of the molecule, and the zigzag lines represent the nonpolar fatty acid chains. (The kink in the fatty acid chain is not shown.) Steroids (1 of 2) Steroids are lipids with structures based on a framework of four carbon rings Sterols, the most common steroids, have a single polar OH group linked to one end of the ring framework and a complex, nonpolar hydrocarbon chain at the other end Cholesterol is an important component of animal cell membranes Similar sterols (phytosterols) occur in plant cell membranes Steroids (2 of 2) (A)Typical arrangement of four carbon rings in a steroid molecule. (B)A sterol, cholesterol. Sterols have a hydrocarbon side chain linked to the ring structure at one end and a single -OH group at the other end (boxed in red). The -OH group makes its end of a sterol slightly polar. The rest of the molecule is nonpolar. Steroid Hormones Steroid hormones control development, behavior, and many internal biochemical processes Examples: The sex hormones that control differentiation of the sexes and sexual behavior Estradiol (female sex hormone) has an —OH in the position where testosterone (male sex hormone) has an =O, and testosterone has a methyl group (—CH3) that is absent from estradiol Difference between Estradiol and Testosterone Steroid Sex Hormones The female sex hormone, estradiol (A), and the male sex hormone, testosterone (B), differ only in substitution of an - OH group for an oxygen and the absence of one methyl group (-CH3) in the estrogen. Although small, these differences greatly alter sexual structures and behavior in animals, such as humans. Other Lipids Several other lipid types have structures unrelated to triglycerides, phospholipids, or steroids Chlorophylls and carotenoids are pigments that absorb light and help convert it to chemical energy in plants Lipid groups combine with carbohydrates to form glycolipids, and with proteins to form lipoproteins, which have important structural and functional roles in cell membranes 3.4 Proteins Proteins perform many vital functions in living organisms Structural support; enzymes; movement; transport; recognition and receptor molecules; regulation of proteins and DNA; hormones; antibodies; toxins and venoms Proteins are macromolecules Polymers of amino acid monomers, which contain both an amino and a carboxyl group Major Protein Functions (1 of 2) Major Protein Functions (2 of 2) Amino Acids (1 of 4) All organisms use 20 different amino acids to build proteins Most have the same structural plan: a central carbon atom is attached to an amino group, a carboxyl group, a hydrogen atom, and a variable Rgroup Amino Acids (3 of 4) One amino acid, proline, differs slightly in that it has a ring structure that includes the central carbon atom – the central carbon bonds to a —COOH group on one side and to an =NH (imino) group at the other side All amino acids can act as acids or bases The amino group can produce a basic reaction by accepting H+, or the carboxyl group can produce an acidic reaction by releasing H+ Amino Acids (4 of 4) Some chemical groups are polar and some are nonpolar Among the polar chemical groups, some carry a positive or negative charge and some act as acids or bases Many chemical groups contain reactive functional groups, such as —NH2, —OH, —COOH, or —SH, which interact with other atoms in the same protein or outide the protein Sulfhydryl groups (in cysteines) can produce disulfide linkages (—S—S—) that help hold proteins in their 3-D shape Nonpolar Amino Acids Practice different types/groups of Amino acid structures, 3-letter abbreviations, and 1-letter abbreviation for exam. The R group (side chain) of each amino acid is boxed in light brown. The amino acids are shown in the ionic forms in which they are found at the pH within the cell. Three-letter and one- letter abbreviations commonly used for the amino acids appear below each diagram. All amino acids assembled into proteins are in the l-form, one of two possible stereoisomers. Uncharged Polar Amino Acids Negatively Charged Amino Acids Positively Charged Amino Acids A Disulfide Linkage The linkage is formed by a reaction between the sulfhydryl groups (-SH) of cysteines. The circled “R”s indicate the side chains of other amino acids in the chains. Figure 3.21 shows how disulfide linkages help maintain a protein’s conformation. Russell, Biology: The Dynamic Science, 5th edition. © 2021 Cengage. All Rights Reserved. May not be scanned, copied or duplicated, or posted to a publicly accessible website, in whole or in part. Peptide Bonds (1 of 2) Covalentpeptide bondslink amino acids into polypeptide chains – the subunits of proteins A peptide bond is formed by a dehydration synthesis reaction between the —NH2 group of one amino aci and the —COOH group of another amino acid The growing polypeptide chain has an N-terminal endand a C-terminal end New amino acids are linked only to the C-terminal end Russell, Biology: The Dynamic Science, 5th edition. © 2021 Cengage. All Rights Reserved. May not be scanned, copied or duplicated, or posted to a publicly accessible website, in whole or in part. Peptide Bonds (2 of 2) The reaction is a typical dehydration synthesis reaction. Four Levels of Protein Structure Protein Structure The protein shown in: (C) is one of the subunits of a hemoglobin molecule; the heme group (in red) is an iron-containing group that binds oxygen. (D) A complete hemoglobin molecule. Primary Structure Primary structure of a protein is the precise sequence in which amino acids are linked Changing even a single amino acid alters secondary, tertiary, and quaternary structures – which can alter or destroy the biological function of a protein Example: Substitution of a single amino acid in hemoglobin produces an altered form responsible for sickle-cell disease Secondary Structure The amino acid chain (primary structure) is folded into arrangements that form the protein’s secondary structure The alpha(α) helix is twisted into a regular right-hand spiral The beta (β) strandzigzags in a flat plane, forming a sheet Most proteins have segments of both arrangements An α Helix, a type of secondary structure in proteins Amino acid chemical groups extend outward from the twisted backbone Stabilized by regularly spaced hydrogen bonds Forms igid,rod-like structures (A)Ball-and-stick model of the α-helix. The backbone of the amino acid chain is held in a spiral by hydrogen bonds formed at regular intervals between backbone atoms. (B)The cylinder often is used to depict an α-helix in protein diagrams; peptide and hydrogen bonds may also be shown. A β-Sheet, formed by side-by-side alignment of two-β strands, a type of secondary structure in proteins. The amino acid chain zigzags in a flat plane β strands are aligned side by side in the same or opposite directions Hydrogen bonds stabilize the sheet The β-strands are held together stably by hydrogen bonds. In this sheet, the β-strands run in opposite directions, as shown by the arrows, which point in the direction of the C-terminal end of each polypeptide chain. Strands may also run in the same direction in a β-sheet. Arrowsalone often are used to represent β-strands in protein diagrams. The Random Coil A random coilhas an irregularly folded arrangement Segments of random coil provide flexible sites that allow α- helical or β-strand segments to bend or fold back on themselves Segments of random coil act as “hinges” that allow major parts of proteins to move with respect to one another Tertiary Structure (1 of 2) Tertiary structure gives a protein its overall three-dimensional shape, or conformation The positions of secondary structures, disulfide linkages, and hydrogen bonds play major roles in folding each protein into its tertiary structure Attractions between positively and negatively charged chemical groups and polar or nonpolar associations also contribute to tertiary structure Tertiary structure determines a protein’s functin The distribution and 3-D arrangement of chemical groups, in combination with their chemical properties, determine the oveall chemical activity of the protein Tertiary structure also determinesthe solubilityof a protein, depending on the arrangement of polar (hydrophilic) and nonpolar (hydrophobic) segments Tertiary Structure (2 of 2) Tertiary structure of most proteins is flexible, allowing them to undergo limited conformational changes Conformational changes are important to the function of enzymes, and to proteins involved in cellular movements or transport of substances across cell membranes Five important types of R-group interactions: Hydrogen bonding—form between polar side chains and opposite partial charges 1. 2. Hydrophobic interactions—water forces hydrophobic side chains together 3. van der Waals interactions—weak electrical interactions, between hydrophobic side chains 4. Covalent bonding—covalent bonds between side chains of sulfhydryl groups (disulfide bonds) 5. Ionic bonding—form between groups with full and opposing charges Figure 3.9 Tertiary Structure of Proteins Results from Interactions Involving R-Groups Tertiary Structure of Lysozyme Lysozyme is an enzyme found in nasal mucus, tears, and other body secretions; It destroys the cell walls of bacteria by breaking down molecules in the wall. Disulfide bonds are shown in red. A space-filling model of lysozyme is shown for comparison. Protein Structures: From Primary to Tertiary Interactive Protein Folding: https://www.labxchange.org/library/items /lb:LabXchange:f93a9e87:lx_simulation:1 Denaturation Unfolding a protein from its active conformation so that it loses its structure and function (caused by chemicals, changes in pH, or high temperatures) is called denaturation Experiments by Christian Anfinsen showed that breaking the disulfide linkages holding the protein ribonuclease in its functional state caused it to unfolded and lose enzyme activity For some proteins, denaturation is permanent – for others, denaturation is reversible (renaturation) Misfolding can be “infectious:” Normal proteins can be indued to fold into infectious, disease-causing agents Prions—altered folded forms of normal proteins: Prion protein (P r P) responsible for ”mad cow disease” Prions can induce normal protein molecules to change their shapeto the altered form Experimental Research: Anfinsen’s Experiment (urea and b-mercaptoethanol) Question: What is the relationship between the amino acid sequence of a protein and its conformation? Anfinsen realized that oxygen from the air had reacted with the OSH groups of the denatured enzyme causing disulfide linkages to reform, and that the enzyme had spontaneously refolded into its native, active conformation Conclusion: Anfinsen concluded that the information for determining the three-dimensional shape of ribonuclease is in its amino acid sequence. Chaperonins Proteins fold gradually as they are assembled – as successive amino acids are linked into the primary structure, the chain folds into increasingly complex structures For many proteins, “guide” proteins called chaperone proteins or chaperonins: bind temporarily with newly synthesized proteins, directing their conformation toward the correct tertiary structure and inhibiting incorrect arrangements Role of a Chaperonin The type of molecular chaperone shown is a chaperonin. Three parts of the chaperonin are the top and bottom, which form a cylinder, and the cap. Quaternary Structure Some complex proteins, such as hemoglobin and antibody molecules, have quaternary structure – the presence and arrangement of two or more polypeptide chains Hydrogen bonds, polar and nonpolar attractions, and disulfide linkages hold the multiple polypeptide chains together Chaperonins promote correct association of the individual amino acid chains and inhibit incorrect formations Functional Domains In many proteins, folding of the amino acid chain (or chains) produces large subdivisions called domains In proteins with multiple functions, individual functions are often located in different domains Domains with similar functions are found in different proteins 3-D arrangement of amino acid chains within and between domains produces highly specialized regions called motifs Two Domains in an Enzyme (A) Two domains in part of an enzyme that assembles DNA molecules in the bacterium Escherichia coli. The α-helices are shown as cylinders, the β-strands as arrows, and the random coils as ropes. “N” indicates the N-terminal end of the protein, and “C” indicates the C-terminal end. (B) The same view of the protein as in (A), showing the domain surfaces and functional sites. Protein Combinations Proteins link with lipids to form lipoproteins, which form parts of cell membranes Proteins link with carbohydrates to form glycoproteins, which function as enzymes, antibodies, recognition and receptor molecules, and parts of extracellular supports Proteins link with nucleic acids to form nucleoproteins, which form structures such as chromosomes 3.5 Nucleotides and Nucleic Acids Nucleic acids are macromolecules assembled from repeating monomers called nucleotides DNA (deoxyribonucleic acid) stores hereditary information responsible for inherited traits in all eukaryotes and prokaryotes and in a large group of viruses RNA (ribonucleic acid) is the hereditary molecule of another large group of viruses – three major types of RNA are involved in protein synthesis Nucleotides A nucleotide, the monomer of nucleic acids, consists of three parts linked together by covalent bonds: A nitrogenous base formed from rings of carbon and nitrogen atoms A five-carbon, ring-shaped sugar One to three phosphate groups Nucleotide Structure Nucleotide: Nitrogenous base + sugar + Phosphate group (s) Nucleoside: Nitrogenous base + sugar Two Types of Nitrogenous Bases Pyrimidines Nitrogenous bases with one carbon-nitrogen ring Uracil (U), thymine (T), and cytosine (C) Purines Nitrogenous bases with two carbon–nitrogen rings Adenine (A) and guanine (G) Nitrogenous Bases Practice different types/groups of Nitrogenous base and 1-letter abbreviation for exam. Red arrows indicate where the bases link to ribose or deoxyribose sugars in the formation of nucleotides. Ring-shaped Sugars Nitrogenous bases link covalently to a five-carbon sugar: Deoxyribose in DNA deoxyribonucleotides Ribose in RNA ribonucleotides The two sugars differ only in the chemical group bound to the 2′ carbon (—H in deoxyribose, —OH in ribose) In unlinked nucleotides: 1, 2, or 3 phosphate groups bond to the ribose or deoxyribose sugar at the 5′ carbon Figure 4-1 Practice and memorize all these structures, for exam Nucleosides and Nucleotide Phosphates A structure containing only a nitrogenous base and a five- carbon sugar is a nucleoside A nucleotide is a nucleoside phosphate Examples: Adenosine monophosphate (AMP) Adenosine diphosphate (ADP) Adenosine triphosphate (ATP) DNA and RNA DNA and RNA consist of polynucleotide chains, with one nucleotide linked to the next by a phosphodiester bond One nucleotide is linked to the next by a bridging phosphate group between the 5′ carbon of one sugar and the 3′ carbon of the next sugar Alternating sugar and phosphate groups forms the backbone of a nucleic acid chain The DNA Molecule The DNA molecule is a double helix (double-stranded) consisting of two polynucleotide chains wrapped around each other in a spiral that resembles a twisted ladder The sides of the ladder are the sugar- phosphate backbones of the two chains The rungs of the ladder are nitrogenous bases which extend inward from the sugars toward the center of the helix Figure 4-5 DNA Base Pairs (1 of 2) The two polynucleotide chains of a DNA double hlix are held together by hydrogen bonds between the base pairs A base pair consists of one purine and one pyrimidine Adenine pairs only with thymine (A–T), forming two stabilizing hydrogen bonds Guanine pairs only with cytosine (G–C), forming three hydrogen bonds Figure 4-5/6 D N A Strands Form an Antiparallel Double Helix (2 of 2) D N A is put together like a ladder: Antiparallel sugar–phosphate backbones form ladder side rails Bases attached to sugars form ladder rungs Hydrophobic interactions cause double-stranded D N A to twist into helix van Der Waals interactions stbilize strands D N A has two different-sized grooves: 1.Major groove 2.Minor groove Figure 4-7 Figure 4-8 Major groove Length of one complete turn of helix Minor (10 rungs per groove turn) 3.4 nm Distance between bases 0.34 nm Width of Cartoon of base pairing Cartoon of double helix the helix 2.0 nm Complementary Base Pairing Formation of A–T and G–C pairs(Watson-Crick base pair) allows the sequence of one polynucleotide chain to determine the sequence of its partner in the double helix The nucleotide sequence of one chain is said to be complementary to the nucleotide sequence of the other chain In DNA replication, one polynucleotide chain is used as a template for the assembly of a complementary chain according to the A–T and G–C base-pairing rules DNA Base Pairs Complementary Base Pairing in DNA RNA Molecules RNA molecules exist mainly as single polynucleotide chains (single-stranded) – however, RNA molecules can fold back on themselves to form double-helical regions In RNA, the uracil (U) base takes the place of thymine (T), forming A–U base pairs “Hybrid” double helices (an RNA chain paired with a DNA chain) are formed temporarily when RNA copies DNA 4.3 R N A Structure and Function Primary structure of RNA: Four types of nitrogenous bases extending from sugar– phosphate backbone Primary structure of RNA differs from DNA: 1. RNA contains ribose instead of deoxyribose 2. RNA contains uracil instead of thymine 3.2′–OHgroup on ribose is more reactive than –H 4.R N A much less stable than D N A Secondary Structure R N A ’ s secondary structure results from complementary base pairing: A with U; G with C Bases of R N A typically form hydrogen bonds with complementary bases on the same strand R N A strand folds over, forming hairpinstructure: Bases on one part of R N A strand fold over and align with bases on other part of the same strand Two sugar–phosphate strands are anti-parallel Figure 4-10 Loop Complementary Base Hairpin Pairing Directs Single stranded Secondary Structure Double stranded in RNA Stem Nitrogenous bases Figure 4.9 Complementary Base Pairing Directs Tertiary Structure in R N A Table 4.1 D N A and R N A Structure Summary Table 4.1 DNA and RNA Structure R N A Can Function as a Catalytic Molecule Ribozymes: RNAs have catalyze reactions Three-dimensional structure vital to catalytic activity Have active sites, like proteins Ability to catalyze phosphodiester bonds giving rise to possibility that RNA could replicate itself(RNA world)