CELL0008 Developmental Biology Lecture Notes 2023-2024 PDF
Document Details
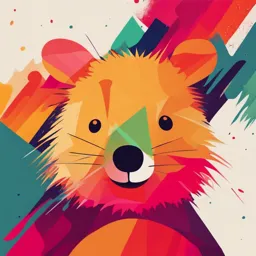
Uploaded by Jenkin
University College London
2024
Dr. Richard Poole
Tags
Summary
These are lecture notes on developmental biology, focusing on embryonic development, model organisms, and key processes. The document covers the aims, objectives, and introduction to the topic, as well as a review of different model organisms.
Full Transcript
CELL0008: Developmental Biology Lecture 1 Lecturer: Dr. Richard Poole Department: Cell and Developmental Biology Location: 202a Anatomy Building Contact: [email protected] Tel (of ce): +44 20 7679 6577 (internal 46577) Notes edited by Dr. Richard Poole based on those generated by Professor Leslie Da...
CELL0008: Developmental Biology Lecture 1 Lecturer: Dr. Richard Poole Department: Cell and Developmental Biology Location: 202a Anatomy Building Contact: [email protected] Tel (of ce): +44 20 7679 6577 (internal 46577) Notes edited by Dr. Richard Poole based on those generated by Professor Leslie Dale (Department of Cell and Developmental Biology) Aims To provide an overview of embryonic development, from the fertilised egg through to the blastula stage. Objectives At the end of this lecture you should be able to describe: The key model organisms used to study development Differences between the four main types of egg Fertilisation of the egg by sperm Holoblastic and meroblastic cleavage divisions Changes to the cell-cycle required for rapid cleavage divisions ________________________________________________________________________________ “The student of Nature wonders the more and is astonished the less, the more conversant he becomes with her operations; but of all the perennial miracles she offers to his inspection, perhaps the most worthy of admiration is the development of a plant or of an animal from its embryo”. Thomas H Huxley (1860). ________________________________________________________________________________ Introduction Developmental biologists, who may also be known as embryologists, look at the formation of a multicellular organism from a single cell, the fertilised egg, to a fully-grown larva and adult. They study how the fertilised egg converts the information in the genome (the genotype) into adult anatomy (the phenotype), passing through a series of increasingly complex embryological and larval forms. Anatomical variation, the basis of evolution, is generated by modifying embryonic or larval development. fi Embryonic development usually begins at fertilisation, when the haploid egg meets, and then fuses, with a single haploid sperm. There are examples in nature whereby development is initiated by the egg without fertilisation, which is called parthenogenesis, but these are rare and there are no examples in mammals1. Fertilisation creates a diploid genome composed of both maternal and paternal genes. Once activated, development follows a series of clearly defined steps that can be recognised in the embryos of most animals: (A) Cleavage, a period of rapid cell divisions in which the large egg is divided into smaller cells. Cleavage divisions usually generate a ball of cells, the blastula, surrounding a fluid filled cavity called the blastocoel. (B) Gastrulation, a phase of coordinated cell movements that generate the multi-layered structure of the embryo. Most animals are triploblasts and composed of three germ layers known as ectoderm, mesoderm and endoderm2. Ectoderm is the outer layer of the embryo, forming the skin and nervous system. Mesoderm is the middle layer of the embryo and forms the skeleton, muscle, connective tissue, blood, heart and kidney. Endoderm is the inner most layer of the embryo and forms the digestive tract and associated organs such as the lungs, liver and pancreas. (C) Neurulation is the formation of an internal nervous system, which is part of the surface ectoderm following gastrulation. (D) Organogenesis is the differentiation of the organs and tissues so that they are fully functional. (E) Metamorphosis is the transformation of the larva into an adult organism. It occurs relatively late in development and may involve major remodelling of the body. Model Organisms Most developmental biologists study the embryos of just a few model organisms, chosen because they are relatively easy to keep in the laboratory and are ideal for the experiments that they wish to perform. The main model organisms include the mouse (Mus musculus), chick (Gallus gallus), frog (Xenopus laevis), fish (Danio rerio), fruit fly (Drosophila melanogaster), and nematode (Caenorhabditis elegans). Additional model organisms include sea urchins and ascidians (sea squirts)3, with many different species being used. Many insects other than Drosophila have also been used, including the red flour beetle Tribolium castaneum and the silk moth Bombyx mori. They are primarily used to compare their genetic programmes for embryonic development with that of Drosophila melanogaster. Tribolium, for example, has a radically different embryonic form to that of Drosophila4. Recent advances in genome sequencing technology and CRISPR-based genome editing techniques may well lead to an expansion in the range of model systems. Although current model organisms represent only a small fraction of the huge diversity of animal forms, the evolutionary distance between them is so large (>500 million years) that we can be confident that any features possessed by them all would have been present in their last common ancestor5. It is now clear that all embryos make use of a common “genetic toolkit” to build their bodies, although the way they use this toolkit may vary. Toolkit genes include the Hox genes6 required for anterior-posterior patterning, Pax6 required for eye development, and Chordin/BMP required for neural development. The similarity of these genes in divergent organisms has been demonstrated in transgenic studies, when they are inserted into the genomes of very different organisms. For example, the vertebrate transcription factor Pax6 is localised to the developing eyefield7 and causes eye defects when mutated8. Moreover, it will induce ectopic eyes when expressed more widely in the head. Pax6 is very similar to a Drosophila gene called eyeless9, so named because the eyes are missing in flies that are homozygous for loss of function mutations in this gene. eyeless will also induce ectopic eyes when expressed in other tissues, the legs and wings for example. Pax6 and eyeless are known as orthologues, the same gene from different animals, and mammalian Pax6 will induce ectopic (insect) eyes when expressed in the legs and wings of Drosophila. The mouse (Mus musculus) was chosen because it is easy to maintain and handle in the laboratory, has a high reproductive rate, and is a mammal. It should therefore be a good model for human development, although there are some differences. The mouse has very powerful genetics and for many years was the only vertebrate in which genetic mutations could be targeted to a specific gene of interest (knockouts). Thomas Capecchi, Sir Martin Evans, and Oliver Smithies were awarded the 2007 Nobel Prize in Physiology or Medicine "for their discoveries of principles for introducing specific gene modifications in mice by the use of embryonic stem cells"10. Mouse mutants are used extensively in biomedical research, modelling numerous human genetic diseases and studying embryonic development. Transgenesis, when foreign genes are inserted into the genome, has also been used extensively in the mouse. However, because mammalian development occurs inside the uterus the mouse embryo is inaccessible and its small size makces micromanipulation very difficult. Chickens (Gallus gallus domesticus) have a long history as a research model for developmental studies. Aristotle (384–322 BCE), for example, cracked open chicken eggs on each day of their 3-week incubation period and recorded the changes that he could observe with his naked eye. The first microscopic study of embryonic development was also performed on chicken embryos (in 1673), by Marcello Malpighi (1628-1694). Chicken eggs were chosen because they are large, easy to obtain, and accessible at most developmental stages11. Their large eggs/embryos mean that they are excellent for micromanipulation (grafting studies) and it is also possible to modify gene function. Like mammals, the chick is an amniote12 and shares many developmental features with humans. Chicken eggs have also been used to propagate viruses to aid in the development of vaccines. The chick genome has been sequenced and shows significant similarity to that of mammals. The South African clawed frog Xenopus laevis has been the main amphibian model for more than 50 years. Amphibians have a long history of developmental studies, but for much of this time numerous species of salamander were the amphibians of choice. Xenopus laevis came to prominence because it can be maintained in the laboratory and egg laying can be induced all year by injecting females with human pregnancy hormone, Chorionic Gonadotrophin13. They lay large numbers (several thousand) of large eggs that are easy to maintain, accessible at all stages of development, and excellent for micromanipulation. It is very easy to inject reagents into Xenopus embryos, including dyes, mRNA, and DNA. When combined with molecular techniques it is easy to regulate the activity of many genes and assess their roles during embryonic development. Sir John Gurdon used Xenopus laevis for his pioneering studies on single cell nuclear transfer (animal cloning) for which he was awarded the 2012 Nobel Prize in Physiology or Medicine “for the discovery that mature cells can be reprogrammed to become pluripotent”14. However, its long-life cycle (1-2 years to reach sexual maturity) and largely tetraploid genome, mean that it is not suitable for classical genetics. A related diploid species, Xenopus tropicalis, has a shorter life cycle (6-12 months to reach sexual maturity) and is being used for both embryological and genetic studies. The genomes of both species have been sequenced and shows significant similarity to that of mammals. Zebra sh (Danio rerio) is the main fish model organism and one of the most recent to be adopted, with large scale studies beginning in the early 1990s. Largely the result of pioneering studies by Nobel laureate Christiane Nüsslein-Volhard, who initiated a systematic attempt to identify all the genes that are involved in zebrafish development. It was chosen because of the ease with which large scale genetic screens can be performed and large numbers of mutations that effect embryonic development have now been identified and studied in detail. Zebrafish produce large numbers of transparent embryos that are accessible at all stages of development and easy to study using microscopes. They are easy to inject with reagents that regulate gene function but micromanipulation is difficult. The zebrafish genome has been sequenced and shows significant similarity to that of mammals. fi The fruit fly Drosophila melanogaster has been used for genetic studies since the pioneering work of Thomas Hunt Morgan (1866-1945) in the early 20th century. It was Morgan’s group that showed that genes are linked to chromosomes, for which he was awarded the 1933 Nobel Prize for Physiology or Medicine "for his discoveries concerning the role played by the chromosome in heredity". Although mutations that affect Drosophila development were quickly isolated and studied, most notably by Edward Lewis (1918-2004), it was not until 1978 that a systematic attempt to identify, by mutation, all the genes involved in development began. These studies were pioneered by Christiane Nüsslein-Volhard and Eric Wieschaus, and were so successful that we understand the genetic control of Drosophila better than any other organism. Lewis, Nüsslein-Volhard, and Wieschaus were awarded the 1995 Nobel Prize for Physiology or Medicine "for their discoveries concerning the genetic control of early embryonic development". In total six Nobel prizes have been awarded for work performed in Drosophila. Most recently, Jeffery Hall, Michael Rosbash and Michael Young were awarded the 2017 Nobel Prize for Physiology or Medicine “for their discoveries of the molecular mechanisms controlling the circadian rhythm”. The Drosophila genome has been fully sequenced and comparisons with the human genome have shown that they contain many genes (>50%) that are very similar to human genes. By identifying homologues of important Drosophila genes, vertebrate embryologists have identified many genes that play an essential role in regulating vertebrate development. Most recently One difficulty with Drosophila is that it is impossible to perform micromanipulations on the embryo. The nematode worm Caenorhabditis elegans is another animal that combines great genetics with a remarkable ability to visualise development down the microscope. It was developed as a model organism by Sydney Brenner, who with Sir John Sulston and Robert Horvitz was awarded the 2002 Nobel Prize for Physiology or Medicine "for their discoveries concerning genetic regulation of organ development and programmed cell death". C. elegans was the first animal to have its genome fully sequenced and it shares significant similarities with the human and Drosophila genomes. It was also the first animal to have its connectome, the connectivity between each and every neuron in the nervous system, fully described. C. elegans has a precisely defined cell-lineage that led early workers to believe that it was an excellent model for mosaic development. It is possible to ablate individual cells using lasers and observe the effects on development. These studies showed that cell-cell interactions are in fact also required for development. Gametes Sperm and eggs are haploid cells produced in the gonads (testes and ovaries respectively) by a process known as gametogenesis, which requires a specialised form of cell-division called meiosis. In males, meiotic divisions are usually equal and produce 4 spermatozoa, while in females they are usually unequal and produce a single ovum and two polar bodies15 Eggs vary enormously in size but they are always much larger than both the sperm and somatic cells. For example, the diameter of the egg is about 0.1 mm in mammals, 0.8 mm in zebrafish, 1.3 mm in Xenopus laevis, and 5 cm in the chick16. Eggs are so large because the mother packs them full of all the ‘goodies’ that are necessary to get them through early development, until they are able to feed for themselves or, in the case of mammals, gain nutrition through the placenta. They are usually stockpiled with yolk proteins17 (90% of protein in amphibian eggs), which are synthesised in maternal organs and transported to the egg18. Eggs from different animals can be classified based on their yolk content and distribution within the cytoplasm. Isolecithal eggs tend to be relatively small and have little yolk that is evenly distributed in the cytoplasm. Most mammalian eggs, including mouse and human, belong to this class, as do the eggs of C. elegans. Mesolecithal eggs tend to be large eggs with moderate to large amounts of yolk that is localised to one half of the egg, the vegetal hemisphere. Most amphibian eggs, including Xenopus laevis eggs, belong to this class. Telolecithal eggs have moderate to large amounts of yolk that fill the cytoplasm, except for a small area near the animal pole. Most fish, reptile, and bird eggs, including zebrafish and chick, belong to this class. Finally, centrolecithal eggs have yolk that is concentrated in the centre of the egg, with a peripheral layer of yolk free periplasm. Drosophila melanogaster have eggs belonging to this class. The egg may also contain a large supply of proteins to carryout normal cellular functions, such as polymerases for DNA and RNA synthesis, ribosomes for protein synthesis, and enzymes for metabolism. Large amounts of RNA, including mRNA, are also stored in the egg. These are all synthesised by the oocyte itself, or in the case of insects by ovarian nurse cells19. Since all the eggs contents are formed by the action of the mother’s genome they are referred to as maternal products. Many eggs are clearly polarised into animal and vegetal hemispheres. The animal hemisphere is usually defined by the presence of the eggs nucleus, called the germinal vesicle, while the vegetal hemisphere is the opposite side of the egg. In many eggs, and especially mesolecithal eggs, the vegetal hemisphere contains most of the yolk. The animal-vegetal axis is most obvious in amphibians, where the animal hemisphere is darkly pigmented and the vegetal hemisphere lightly pigmented. Egg development is initiated during embryonic development and all the eggs a female mammal will ever produce are formed in the fetal ovary20. Meiosis is unequal in females, producing a single large ovum and two small polar bodies (small cellular fragments containing the discarded chromosomes). However, meiosis is not normally completed until just prior to ovulation, with many insects holding the egg at metaphase I and most mammals, including humans, holding the egg at prophase I. In mammals, meiosis is reinitiated by ovulation but is then held at metaphase II until fertilisation. Spermatozoa are very different in both size and shape from the egg, having discarded most of their cytoplasm during maturation21. The head of a mature sperm consists of a highly condensed haploid nucleus, centriole and a cap structure called the acrosome. Behind the head, the mid- piece contains the base of the agellum (containing tubulin) and mitochondria for powering movement. The mid-piece and flagellum constitute the tail. Spermatogenesis normally begins in the adult testis and continues throughout the animal’s adult life. Males produce huge numbers of spermatozoa, a typical human male producing >1000 per second for example. Why this is necessary, when only one sperm is required to fertilise the egg, is still largely a matter of conjecture. Many of the sperm may have abnormal morphology (e.g. two heads or two tails) that renders them incapable of fertilising an egg, but up to 85% of human sperm can be abnormal without affecting fertility22. Fertilisation fl Development is usually initiated by the fusion of sperm and egg, although in some species eggs are capable of developing without sperm (parthenogenesis)23. Parthenogenesis does not occur naturally in mammals but mouse embryos have been created in the laboratory that contain only male (andromorphs) or female (gynomorphs) chromosomes. Andromorphs have abnormal growth of placental tissues and no discernible embryo, while gynomorphs have poorly developed placental tissue and as a consequence a poorly formed embryo that soon dies. In humans, hydatidiform moles24 appear to be very similar to andromorphic embryos in mice and are either diploid with only male chromosomes (complete hydatidiform mole) or triploid with two copies of male chromosomes and a single copy of female chromosomes (partial hydatidiform mole). The reasons for this are unknown but likely explanations include loss of the female chromosomes from the egg during meiosis, followed by fertilisation by either a single sperm or multiple sperm (complete hydatidiform mole), and fertilisation of a normal egg by two sperm (partial hydatidiform mole). Both male and female chromosomes are required for successful development in mammals because of genomic imprinting. This is when DNA and associated Histone proteins are chemically modified during gametogenesis (e.g. DNA and Histones may be methylated), such that associated genes are inactive during early embryonic development (this form of non-Mendelian inheritance is known as epigenetics). Up to 80 genes have been shown to be imprinted in mammalian gametes with different genes being inactivated on male and female chromosomes. Thus, only by combining both can a complete set of active genes be created. A number of genetic diseases have been linked with imprinting defects, including Angelman syndrome, Beckwith-Wiedemann syndrome, Prader-Willi syndrome, and Silver-Russell syndrome. The explanation for genomic imprinting is still debated, with the parental conflict hypothesis (or kinship theory) being most widely accepted. However, some aspects of genomic imprinting are difficult to explain with this theory. Whatever the reason for genomic imprinting, one consequence is that parthenogenesis does not normally occur in mammals. Human sperm normally encounter the egg in the ampulla of the fallopian tubes25 (the oviduct). The egg is surrounded by a membrane known as the zona pellucida in mammals, but as the vitelline or fertilisation membrane in other animals. This membrane contains species-specific sperm receptors that prevent fertilisation by sperm of different species. The sperm receptor of the mouse egg is a protein called ZP3 (zona pellucida protein 3) and females that lack a functional Zp3 gene are infertile. Contact between the sperm and zona pellucida induces the acrosome reaction, whereby the acrosome (located at the tip of the sperm) releases enzymes that digest a hole in the zona pellucida that allows the sperm to penetrate through to the plasmamembrane. The sperm now fuses with the plasmamembrane causing a localised increase in cytosolic calcium that quickly spreads throughout the egg. This calcium increase causes cortical granules, lying just beneath the surface of the egg, to fuse with the plasma membrane (cortical reaction) and release their contents into the extracellular space. The enzymes they contain modify the sperm receptor (ZP3) such that sperm can no longer bind or penetrate the zona pellucida. In this way, the mammalian egg prevents polyspermy, which would otherwise result in faulty segregation of chromosomes during mitosis. Polyspermy normally results in developmental arrest in mammals, so it is important that it does not occur26. Many species of egg respond to fertilisation by reversing the charge of the plasmamembrane, which prevents further sperm from fusing with the egg. This is much more rapid than the cortical reaction and is known as the “fast block” to polyspermy. It has not been described in mammalian eggs. It is also worth noting that polyspermy is quite common is some species, chick eggs for example. These eggs must use poorly understood mechanisms for selecting only one of the male nuclei for fusion with the female nucleus, the rest being destroyed. Cleavage Divisions Following fertilisation, the embryo undergoes a period of rapid, normally synchronous cell divisions called cleavage divisions. These cell divisions divide the egg without accompanying growth, creating progressively smaller cells that are usually known as blastomeres. Embryos played a hugely important role in the earliest studies of cell division (in the late 19th Century) and the development of cell theory. All the different stages of M-phase were first described in embryonic cleavage divisions. This is because embryos were readily available and cleavage divisions are both frequent and synchronous. We now know that much of the process of mitosis in embryos is identical to that of cultured cells, including the role of cyclins and cdk27. Cytokinesis, for example, is driven by a contractile ring of actin and myosin filaments that form beneath the plasma membrane, in the same plane that was previously occupied by the metaphase plate. As the actin and myosin filaments slide by one another, the ring contracts and pinches the 2 cells apart. The first cleavage division usually takes longer than subsequent divisions, because the male and female haploid nuclei have to find each other and then fuse to form the diploid zygotic nucleus28. Once this has occurred cleavage divisions can be very rapid, taking just 8 minutes in D. melanogaster, 20-40 minutes in C. elegans, 15-20 minutes in zebrafish, and 25-30 minutes in Xenopus laevis29. Speed is achieved by modifying the mitotic cell cycle so that it only includes S- phase (DNA replication) and M-phase (mitosis/ cytokinesis). The gap phases (G1 and G2) are deleted and even S-phase has to be modified to achieve the fantastic rates of DNA synthesis required for rapid cell division. Frog cleavage stage nuclei, for example, have many more replication origins than nuclei at later stages of development30. Since cleavage nuclei are almost entirely devoted to DNA synthesis, gene transcription is very difficult31. This is one reason why the mother must supply the egg with a large number of maternal RNAs. Cleavage divisions in mammals proceed at a much more leisurely pace and may retain both gap phases32. The number of cleavage divisions (or at least those in which the zygotic genome is almost silent) varies between different animals, but is usually around 12 (but is only 1 or 2 cell divisions in chicks, mice and worms). Cell cycle length may be controlled by the ratio of DNA to cytoplasm, with a critical ratio triggering a slowing of the cell cycle (from 25 minutes to 2-4 hours in Xenopus laevis), the introduction of both G1 and G2 phases, and the onset of embryonic (zygotic) transcription. Cell division is now no longer synchronous and cells may become motile. Developmental biologists recognise two major types of cleavage division, which are called holoblastic and meroblastic. (1) Holoblastic cleavage is found in isolecithal and some mesolecithal eggs and involves the complete cleavage of the egg. It is observed in animals such as sea urchins, ascidians, Caenorhabditis, Xenopus, and mammals. In mammals, cleavage divisions are quite slow (~20 hours) and quickly become asynchronous such that an odd number of blastomeres are often found. At the 16- to 32-cell stage they undergo the process of compaction whereby blastomeres maximise their contacts with each other. The outer layer forms a simple epithelium called the trophoblast and begins to pump water into the extracellular space in the centre of the embryo, creating a cavity called the blastocoel. A small population of non-trophoblast cells is found at one end of the blastocoel and is known as the inner cell mass. The embryo is now known as a blastocyst. In Xenopus eggs the first cleavage is vertical (along the animal-vegetal axis), dividing the embryo into left and right halves. The second cleavage is also vertical but at right angles to the first, while the third cleavage is horizontal and divides the embryo into four animal and four vegetal blastomeres. The fourth cleavage is once again vertical and generates two tiers of eight cells, while the fifth cleavage is horizontal and generates four tiers of eight cells. At this stage, the embryo is referred to as a morula. Beyond this point the plane of cleavage becomes more variable. Water is also pumped into the centre of the Xenopus embryo to form a blastocoel and the embryo becomes known as a blastula. fi (2) Meroblastic cleavage usually occurs in telolecithal and centrolecithal eggs with large amounts of yolk, where complete cleavage is very difficult. We can further divide this class of egg into those with discoidal cleavage (telolecithal eggs) and those with superficial cleavage (centrolecithal eggs). In discoidal cleavage only a small, relatively yolk free, portion of the egg is cleaved, typically located at the animal pole. Organisms with this type of cleavage include zebrafish33 and the chick. In zebrafish embryos, the first three cleavages are vertical (along the animal-vegetal axis), giving a single layer of 8-cells sitting on top of the yolk. They are only partial, incompletely separating blastomeres from the large yolk cell. The 4th cleavage is horizontal and generates two layers of 8 cells each. Chick eggs are very large and very rich in yolk, with a very small yolk free region at the animal pole. Cleavage divisions start at the animal pole but do not progress very far, creating a layer of small cells on top of a huge uncleaved yolk filled area. This is known as the blastoderm and consists of about 60,000 cells when the egg is laid. Super cial cleavage is found in some insects, including the fruitfly Drosophila melanogaster34. Male and female haploid nuclei fuse deep within the yolky centre of the egg, where the first nine nuclear divisions will occur. Nuclear division is not accompanied by cytokinesis so cells are not formed, the nuclei remaining within a common cytoplasm (a syncytium). After the 8th division (90 minutes) most of the nuclei migrate to the periphery of the egg, to form the syncytial blastoderm. Four nuclear divisions later, plasma membrane grows between the nuclei to form cells, giving rise to the cellular blastoderm. The first cells to form are located at the posterior pole of the embryo and will form the germ cells, progenitors of the future gametes. When cleavage divisions are very rapid it is almost impossible to transcribe the genome so the embryo must depend on maternal products laid down during oogenesis. However, the embryo must begin to process of forming all of its many different cell-types and must move cells around to build anatomy. For this the embryo requires new gene products so the genome must start to be transcribed. This process begins at the midblastula stage in amphibians and at an equivalent stage in other embryos (such as Drosophila and zebrafish). To achieve this blastomeres increase the length of the cell-cycle by introducing G1 and G2 phases, a longer S-phase, and cytokinesis (in Drosophila). Cleavage divisions become asynchronous and blastomeres become motile. The switch from cell divisions without gap phases to ones with gap phases is usually referred to as the mid-blastula transition (MBT). Gap phases allow the zygotic/embryonic genome to be transcribed and to replace maternal transcripts, which are gradually destroyed. The initiation of zygotic transcription is known as the maternal-to-zygotic transition (MZT). Many genes are transcribed for the first time at the MZT, including a gene called brachyury. This gene is found in the mesoderm of all chordate embryos (including humans), at equivalent stages of development, and is used as a molecular marker for mesoderm at a time when no discernible differences can be detected down a microscope. Zygotic gene products like brachyury35 are utilised by the embryo to establish the earliest differences in cell fate (e.g. ectoderm, mesoderm, and endoderm) and regulate the next major phase of embryogenesis – gastrulation. An clear MBT/MZT is not found in either chick or mouse embryos (and presumably other amniote species, including humans), which transcribe their zygotic genomes from at least the 2-cell stage. It is also not found in C. elegans which begin zygotic transcription of some genes at the 4cell stage. Further Reading Scott Gilbert, Developmental Biology Lewis Wolpert, Principles of Development Gary Schoenwolf, Larsen’s Human Embryology All three books can be found in UCL Library and you don’t need the latest editions. Endnotes Aphids largely reproduce by parthenogenesis in the spring and summer, but sexually in the autumn. Parthenogenesis does not occur naturally in mammals and leads to early death of the embryo when produced experimentally. 1 Animals with three germ layers are known as triploblasts, while diploblasts have only ectoderm and endoderm. 2 Urochordates, the closest living relatives of vertebrates which have larvae that are remarkably similar to frog tadpoles. 3 Drosophila is a long germ insect (all segments formed at the same time) and Trilbolium a short germ insect (head segments form rst then trunk followed by abdomen). 4 The last common ancestor of all bilateral animals is known as Urbilateria. Its anatomy has been reconstructed from features conserved in most of its descendants, including all protostomes and deuterostomes, but is still a matter of debate. However, it would have had all three germ layers and clearly de ned anterior-posterior, dorsal-ventral, and left-right axes. It is traditionally considered as a worm-like animal but other anatomies are feasible. 5 Gene names (DNA and RNA) are always spelt in italics, while protein names are always spelt in normal text. The convention for the use of italics varies between species but abbreviated name of human genes is always in capitals (e.g. PAX6), while only the rst letter is a capital in mice (e.g. Pax6) and only lower case is used in frogs and sh (e.g. pax6). 6 The eye eld of vertebrate neurulae will form the retina and iris of the eye, but not the lens or cornea. 7 8 In humans, PAX6 mutations are responsible for a defective eye condition known as aniridia. Many Drosophila genes were rst identi ed as mutations with clear anatomical defects and the tradition is to use the defect in the gene name. Thus, the white gene was originally identi ed as a mutation causing white eyes, eyeless as a mutation causing loss of eyes, and wingless as a mutation causing loss of wings. 9 10 Many Nobel Prizes have been awarded for work performed on mice. 11 For the rst 24 hours, the egg is in the oviduct and therefore inaccessible. Amniotes are vertebrates that have embryos with an amniotic membrane and include the reptiles, birds, and mammals. In contrast, anamniotes have embryos without an amniotic membrane, including the sh and amphibia. 12 Xenopus leavis was introduced into UK laboratories in the 1930’s and was quickly adopted as a pregnancy test, egg laying being induced by the high concentrations of Chorionic Gonadotrophin in the urine of pregnant women. 13 14 He shared the prize with Shinya Yanamaka, who discovered induced pluripotent stem cells. 15 Polar bodies are small cellular fragments containing the discarded chromosomes. 16 Ostrich eggs may be as large as 15 cm. Vertebrate yolk proteins are encoded by 3 vitellogenin genes. Human eggs don’t contain yolk proteins, but the vitellogenin genes can still be detected in the human genome, as highly mutagenised pseudogenes. 17 In vertebrates, yolk proteins are usually synthesized in the liver and transported to the ovary via the bloodstream. 18 Nurse cells are sister cells of the oocyte. They retain cytoplasmic connections with the oocyte through which RNA and protein are transported. 19 Human females produce about 6 million oocytes in the fetal ovary, a gure that drops to about 1-2 million at birth and about 40,000 at puberty. 20 Some insect sperm have such long tails (over 2 inches in some cases) that they are actually longer than the length of the egg. However, the volume of these sperm is always much less than that of the egg. fi fi fi fi fi fi fi fi fi fi fi 21 22 It is not known why human males produce so much abnormal sperm. Parthenogenesis is predominantly observed in plants and invertebrate animals (e.g. aphids, rotifers, nematodes), but it has also been observed in some sh, reptiles and birds. 23 Hydatidiform moles occur in approximately 1 in 500 pregnancies and are characterised by abnormal growth of placental tissues, particularly the trophoblast (it is also known as trophoblastic disease), producing swollen chorionic villi. Any associated embryo is not viable and the hydatidiform mole is evacuated from the uterus. Some may convert to a tumour called a choriocarcinoma. 24 The ampulla is that region of the fallopian tubes (also known as the oviduct) closest to the ovary. 25 Polyspermy is not uncommon and occurs in chickens for example. Mechanisms must exist to ensure that only one sperm nucleus fuses with the egg nucleus. 26 27 Cyclins were discovered by Sir Tim Hunt while studying sea urchin embryos. 28 Zygote is another name for the fertilised egg. In many cases the timing is temperature dependent. Xenopus cleavages take 25-30 minutes at 21°C but 80-90 minutes at 13°C. 29 30 Replication origins are speci c DNA sequences used to initiate DNA replication. 31 Most genes are also far too large to be fully transcribe in these short cell-cycle times. As a consequence, transcription from the zygotic genome may be initiated as early as the 2-cell stage. 32 33 Even though zebra sh embryos are smaller and less yolky than Xenopus embryos! 34 Super cial cleavage is not found in all insects and is most prominent in Diptera (true ies). Brachyury was rst identi ed as a mouse mutation with tail defects (the name means short tail) when heterozygous. Inhibiting its function causes tail defects in both zebra sh, Xenopus, and ascidians. Homozygous mutant mouse embryos die very early in development as they fail to gastrulate. Brachyury encodes a member of the T-box family of transcription factors, which has more that 20 members in the human genome. fl fi fi fi fi fi fi fi 35