Lecture 6 BIO3147 (2024) PDF
Document Details
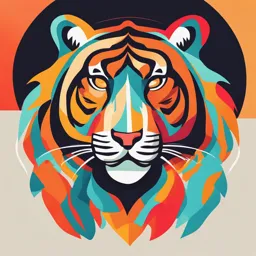
Uploaded by SafeMahoganyObsidian
null
2024
Tags
Summary
Lecture 6 BIO3147 (2024) is a lecture on developmental biology, covering various topics including the model of ribosomal heterogeneity in mice, maternal contributions to DNA replication, and RNA interference.
Full Transcript
Figure 3.26 Model of ribosomal heterogeneity in mice (12th edition) (A) Ribosomes have slightly different proteins depending on the tissue in which they reside. Ribosomal protein Rpl38 (i.e., protein 38 of the large ribosomal subunit) is concentrated in those ribosomes found in the somites that give...
Figure 3.26 Model of ribosomal heterogeneity in mice (12th edition) (A) Ribosomes have slightly different proteins depending on the tissue in which they reside. Ribosomal protein Rpl38 (i.e., protein 38 of the large ribosomal subunit) is concentrated in those ribosomes found in the somites that give rise to the vertebrae. (B) A wild-type embryo (left) has normal vertebrae and normal Hox gene translation. Mice deficient in Rpl38 have reduced Hox gene translation and an extra pair of vertebrae. Figure 3.26 Maternal contributions to DNA replication in the zebrafish blastula (A) Wild-type blastulae show BrdUlabeled nuclei (blue) in all cells. (B) Although the correct number of cells is present in futile cycle mutants, they consistently show only 2 labeled nuclei, indicating that these mutants fail to undergo pronuclear fusion. Even in the absence of any zygotic DNA, early cleavages progress perfectly well due to the presence of maternal contributions. However, futile cycle mutant embryos arrest at the onset of gastrulation. Figure 3.28 Model for RNA interference from double-stranded RNA (dsRNA) and miRNA Double-stranded RNA (dsRNA) that is added to a cell or produced through transcription (miRNA). miRNAs are processed by the Drosha RNAase in the cell and then expoxted into the cytoplasm where it will interact with the RNA-induced silencing complex (RISC), made up primarily of Dicer and Argonaute, that prepares the RNA to be used as a guide for targeted mechanisms of interference. Figure 3.29 The role of miR430 during the maternal-to-zygotic transition in zebrafish (A) Numerous mRNAs derived from maternal contributions fuel development during the cleavage stages, but transitioning into the gastrula requires active transcription of the zygotic genome. miRNAs play a major role in clearing these maternally derived transcripts during this transition. (B) miR430 plays a major role in the interference of a majority of maternal transcripts in the zebrafish blastula as it transitions to zygotic control during gastrulation. In this graph, the different curves denote the reduction in three specific transcripts, two genes of which (purple and red) are differentially degraded by miR430 (green). Figure 3.30 Localization of mRNAs (12th edition) (A) Diffusion and local anchoring. nanos mRNA diffuses through the Drosophila egg and is bound (in part by the Oskar protein) at the posterior end of the oocyte. This anchoring allows the nanos mRNA to be translated. (B) Localized protection. The mRNA for Drosophila heat shock protein (Hsp83) will be degraded unless it binds to a protector protein (in this case, also at the posterior end of the oocyte). (C) Active transport on the cytoskeleton, causing the accumulation of mRNA at a particular site. Here, bicoid mRNA is transported along microtubules by the motor protein dynein to the anterior of the oocyte. Meanwhile, oskar mRNA is brought to the posterior pole by the motor protein kinesin along microtubules. Figure 3.31 In situ hybridization (12th edition) (A) Whole mount in situ hybridization for odd-skipped mRNA (blue) in a stage-9 Drosophila embryo. (B) Antisense RNA probe with uridine triphosphate conjugated to digoxigenin (DIG). (C) Illustration of two cells at the border of the odd-skipped expression pattern seen in the box in (A). The cell on the left is not expressing odd-skipped, whereas the cell on the right is. The antisense DIGlabeled RNA probe with complementarity to the oddskipped gene becomes hybridized to any cell expressing oddskipped transcripts. Figure 3.31 In situ hybridization (12th edition) Following probe hybridization, samples are treated with anti-DIG antibodies conjugated to the enzyme alkaline phosphatase. When nitroblue tetrazolium chloride (NBT) and 5-Bromo-4-chloro-3indolyl-phosphate (BICIP) are then added to the sample, alkaline phosphatase converts them to a blue precipitate. Only those cells expressing odd-skipped turn blue. Figure 3.32 Chromatin immunoprecipitation-sequencing (ChIP-Seq) (12th edition) Chromatin is isolated from the cell nuclei. The chromatin proteins are crosslinked to their DNA-binding sites, and the DNA, bound to its proteins, is fragmented into small pieces. Antibodies bind to specific chromatin proteins, and the antibodies—with whatever is bound to them—are precipitated out of solution. The DNA fragments associated with the precipitated complexes are purified from the proteins and sequenced. These sequences can be compared with the genome maps to discover the precise locations of the genes these proteins may be regulating. Figure 3.33 RNA sequencing: RNA-Seq (Part 1) (12th edition) Begin with specific tissues, often comparing different conditions, such as embryos of different ages (chick embryos, as shown here), isolated tissues (such as the eye; boxed regions) or even single cells, and samples from different genotypes or experimental manipulations Figure 3.33 RNA sequencing: RNA-Seq (Part 2) (12th edition) (1) RNA is isolated to obtain only those genes that are actively expressed. (2) These transcripts are then fragmented into smaller stretches and used to create cDNA with reverse transcriptase. (3) Specialized adaptors are ligated to the cDNA ends to enable PCR amplification and immobilization for: (4) Subsequent sequencing. Figure 3.34 CRISPR/Cas9-mediated gene editing (12th edition) The CRISPR/Cas9 system is used to cause targeted indel formation or insertional mutagenesis within a gene of interest. A gene-specific guide RNA (gRNA) is designed and introduced into cells together with the nuclease Cas9, for instance by co-injection into a newly fertilized zygote. The gRNA will bind to the genome with complementarity and will recruit Cas9 to this same location to induce a double-stranded break. Figure 3.34 CRISPR/Cas9-mediated gene editing (12th edition) Non-homologous end joining (NHEJ) is the cell’s DNA repair mechanism that often results in small insertions or deletions (approximately 2–30 base pairs; a 2 base-pair insertion is shown here), which can cause the establishment of a premature stop codon and potential loss of the protein’s function. In addition, plasmids carrying insertions with homology to regions surrounding the gRNA target sites are used to insert known sequences at the double-stranded break. Such methods are being explored as a way to repair mutations. Figure 3.35 Targeted expression of the Pax6 gene in a Drosophila non-eye imaginal disc (12th edition) A strain of Drosophila was constructed wherein the gene for the yeast GAL4 transcription factor was placed downstream from an enhancer sequence that normally stimulates gene expression in the imaginal discs for mouthparts. If crossed to a strain that contains a transgene that places GAL4-binding sites upstream of the Pax6 gene, in the progeny, the Pax6 gene will be expressed in whichever imaginal disc the GAL4 protein is made. (B) Drosophila ommatidia (compound eyes) emerging from the mouthparts of a fruit fly in which the Pax6 gene was expressed in the labial (mouthpart) discs. Figure 3.36 The Cre-lox technique for conditional mutagenesis, by which gene mutations can be generated in specific cells only (12th edition) Mice wild-type alleles (in this case, the genes encoding the Hnf4α transcription factor) have been replaced by alleles in which the second exon is flanked by loxP sequences. These mice are mated with mice having the gene for Cre-recombinase fused to a promoter that is active only in particular cells. In this case, the promoter is that of an albumin gene that functions early in liver development. Figure 3.36 The Cre-lox technique for conditional mutagenesis, by which gene mutations can be generated in specific cells only (12th edition) In mice with both of these altered alleles, Cre-recombinase is made only in the cells where that promoter is activated (i.e., in these cells synthesizing albumin). The Cre-recombinase binds to the loxP sequences flanking exon 2 and removes that exon. Thus, in the case depicted here, only the developing liver cells lack a functional Hnf4α gene. Figure 4.1 Local and long-range modes of cell-to-cell communication (A) Juxtracrine signaling: Local cell signaling is carried out via membrane receptors that bind to proteins in the extracellular matrix (ECM) or directly to receptors from a neighboring cell. (B) Paracrine signaling: Signaling across multiple cell distances, whereby one cell secretes a signaling protein (ligand) into the environment and across the distance of many cells. Only those cells expressing this ligand’s corresponding receptor can respond, either rapidly through chemical reactions in the cytosol, or more slowly through the process of gene and protein expression. (C) Endocrine Signaling: Long range glandular/interorgan signaling of hormones (D) Autocrine Signaling: A cell signaling to itself. Figure 4.3 Reaggregation of cells from amphibian neurulae Presumptive epidermal cells from pigmented embryos and neural plate cells from unpigmented embryos were dissociated and mixed together. The cells reaggregated so that one type (the presumptive epidermis) covered the other. Figure 4.4 Sorting out and reconstruction of spatial relationships in aggregates of embryonic amphibian cells Figure 4.5 Hierarchy of cell sorting of decreasing surface tensions (A) Simple schematic demonstrating a logic statement for the properties of differential cell adhesion. (B) The equilibrium configuration reflects the strength of cell cohesion, with the cell types having the greater cell cohesion segregating inside the cells with less cohesion. Figure 4.6 Simplified scheme of cadherin linkage to the cytoskeleton via catenins Figure 4.7 E-cadherin is required for epiboly in zebrafish (A) Wild-type embryos (right), and embryos heterozygous (center) and homozygous (left) for the Ecadherin mutation. During normal gastrulation, cells merge into a thinner but more expansive epiblast layer that envelops the entire yolk (the red arrowhead points to the location of final yolk enclosure in the wildtype). E-cadherin mutants fail to complete epiboly, which is most severely impaired in the homozygous mutant (red lines denote the leading edge of epiblast). Figure 4.7 E-cadherin is required for epiboly in zebrafish (B) Schematic of radial intercalating cell movements in the zebrafish epiblast over time during gastrulation. EVL, enveloping layer; HB, hypoblast; YSL, yolk syncytial layer. Cells move toward the superficial enveloping layer in relationship to increasing expression of E-cadherin. Ecadherin is expressed at higher levels in the more superficial layers of the epiblast, including the enveloping layer, and it is this differential expression (and consequently differential adhesion) that powers the radial movement of deep cells to the periphery. Figure 4.8 Importance of the amount of cadherin for correct morphogenesis (12th edition) Aggregate surface tension correlates with the number of cadherin molecules on the cell membranes. Sorting can occur based on cadherin number even if the two cells express different cadherin proteins Figure 4.9 Extracellular matrices in the developing embryo (A) Fluorescent antibodies to fibronectin show fibronectin deposition as a green band in the Xenopus embryo during gastrulation. The fibronectin will orient the movements of the mesodermal cells. Figure 4.10 Simplified diagram of the fibronectin receptor complex RGD, arginine-glycine-aspartate Integrins function as transmembrane linkers (or “integrators”), mediating the interactions between the cytoskeleton and the extracellular matrix that are required for cells to grip the matrix. The integrins of the complex can form heterodimerized membrane-spanning receptor proteins that bind fibronectin on the outside of the cell while interacting with actin cytoskeletonassociated proteins such as α-Actinin, Vinculin, and Talin on the inside of the cell. Figure 4.11 Epithelial-mesenchymal transition, or EMT (A) Normal epithelial cells are attached to one another through adherens junctions containing cadherin, catenins, and actin rings. They are attached to the basal lamina (extracellular matrix) through integrins. Paracrine factors can repress the expression of genes that encode these cellular components, causing the cell to lose polarity, lose attachment to the basal lamina, and lose cohesion with other epithelial cells. Cytoskeletal remodeling occurs, as well as the secretion of proteases that degrade the basal lamina and other extracellular matrix components of the basement membrane, enabling the migration of the newly formed mesenchymal cell. Figure 4.11 Epithelial-mesenchymal transition, or EMT (B,C) EMT is seen in vertebrate embryos during the normal formation of neural crest from the dorsal region of the neural tube (B) and during the formation of the mesoderm by mesenchymal cells delaminating from the epiblast (C). Figure 4.12 Ectodermal competence and the ability to respond to the optic vesicle inducer in the frog Xenopus The optic vesicle is able to induce lens formation in the anterior portion of the ectoderm (1) but not in the presumptive trunk and abdomen (2). (3) If the optic vesicle is removed, the surface ectoderm forms either an abnormal lens or no lens at all. (4) Most other tissues are not able to substitute for the optic vesicle. Figure 4.13 Sequence of amphibian lens induction postulated by experiments on embryos of the frog Xenopus laevis Unidentified inducers (possibly from the foregut endoderm and cardiac mesoderm) cause the synthesis of the Otx2 transcription factor in the head ectoderm during the late gastrula stage. As the neural folds rise, inducers from the anterior neural plate (including the region that will form the retina) induce Pax6 expression in the anterior ectoderm that can form lens tissue. Expression of Pax6 protein may constitute the competence of the surface ectoderm to respond to the optic vesicle during the late neurula stage. The optic vesicle secretes BMP and FGF family paracrine factors (see signals in higher magnification of boxed area) that induce the synthesis of the Sox transcription factors and initiate observable lens formation. Figure 4.14 The Pax6 gene is similarly required for eye development in frogs, rats, and humans (12th edition) (A) Loss of Pax6 in rats results in the failure to form eyes as well as significant reductions in nasal structures. (B) An analysis of lens induction following recombination experiments of the optic vesicle and surface ectoderm between wild-type and Pax6 null rat embryos. Pax6 is required only in the surface ectoderm for proper lens induction. (C) Mutations in the Pax6 gene in Xenopus and humans result in similar reductions in the iris of the eye as compared with wild-type individuals. This phenotype is characteristic of aniridia. Figure 4.16 Specification of uniform cells into three cell types by a morphogen gradient (12th edition) Morphogen (red dots): signaling molecules (proteins or otherwise) that act over long distances (paracrine) to induce responses in cells based on the concentration of morphogen that the cells interact with. Cells exposed to morphogen concentrations above threshold 1 (red) activate certain genes. Cells exposed to intermediate concentrations (pink) activate a different set of genes and also inhibit the genes induced at the higher concentrations. Those cells encountering low concentrations of morphogen (below threshold 2; blue) activate a third set of genes