BIOL 200 Building Blocks and Linear Biopolymers Lecture Aug 30, 2024 PDF
Document Details
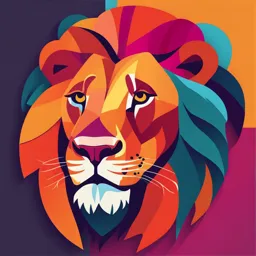
Uploaded by UnparalleledPlutonium8242
2024
Ken Hastings
Tags
Summary
This is a lecture on building blocks and linear biopolymers from BIOL 200. The lecture covers information about monomers of DNA, RNA, and proteins. The lecture is given by Ken Hastings.
Full Transcript
BIOL 200 Aug 30, 2024 Ken Hastings Building blocks and linear biopolymers Lodish Chapter 2.2, 5.1 9th ed pp 44 - 50, 179-185 DNA, RNA and protein: informational biopolymers Polymer = covalent bond-linked chain of monomers Informational polymers ha...
BIOL 200 Aug 30, 2024 Ken Hastings Building blocks and linear biopolymers Lodish Chapter 2.2, 5.1 9th ed pp 44 - 50, 179-185 DNA, RNA and protein: informational biopolymers Polymer = covalent bond-linked chain of monomers Informational polymers have more than one kind of monomer. The order of the different kinds of monomer in the polymer chain (the “sequence”) IS the information In the case of DNA, RNA and protein the information IS the DNA sequence, the RNA sequence, the protein sequence. The informational biopolymer monomers have a common generic structure: a common element, shared by all the different monomers for that class of informational biopolymer. A “characteristic” element that makes each monomer different from the others characteristic element common element The informational biopolymer monomers have a common generic structure: the common element forms the polymer “backbone” by covalent bonding between monomers the characteristic elements form “side-chains” that protrude from the polymer backbone. before polymerization after polymerization covalent bonds The information in an informational biopolymer is the order of the monomer units….the sequence Sequence = Yellow-Red-Green Sequence = Red-Red-Green different sequence….different information Polymer structure arises from monomer chemistry If the monomer has ONE joining site in the common element, at most two monomer units can be joined together. Cannot make polymer. Joining site No joining sites exposed at ends. No further chain growth. If the monomer has TWO joining sites in the common element, then one can make LINEAR polymers of potentially infinite length Joining sites exposed at ends. Further chain growth possible If the monomer has THREE joining sites in the common element, then one can make BRANCHED polymers. Cells CAN make branched polymers, e.g. complex carbohydrates BUT informational biopolymers are NOT branched. They are LINEAR. Packaging and handling of linear molecules may be more efficient than of branched molecules. This is a good thing for scientific progress because it would have been much more difficult to determine the structures if they had been branched. In most cases, linear informational biopolymers have two ends. But in some cases the two ends can be joined, giving a circular (but unbranched) molecule. For example the genomic DNA molecules of bacteria e.g. E.coli and some viruses is a circle. Electron micrograph of a circular DNA molecule. Similar to Fig 5‐8 b in Lodish et al 9th ed Informational biopolymers are made from asymmetric monomers. There are two joining sites per monomer but the two sites are DIFFERENT. two sites the same two sites different A B Let’s call them A and B. A can only join with B, and B can only join with A A BA BA B A BA BA B The asymmetry of the monomers directly drives an asymmetry of the polymer. There is an A end and there is a B end and they are chemically distinct. For all informational biopolymers, DNA, RNA and protein, growth of the chain occurs only at one end. Polymer growth is unidirectional. The convention when showing biopolymer representations on a sheet of paper is to depict the orientation in which unidirectional polymer chain growth occurs In the rightward direction. New monomers are added at the right end. Two major types of informational biopolymer monomer units: nucleotides and amino acids Polymer Monomer Typical chain lengths nucleic acids nucleotides DNA DNA ~10 to ~10 RNA RNA ~20 to ~10 protein amino acids ~100 to ~10 Nucleotides The characteristic element is a heterocyclic base. base this one is adenine pentose phosphate The common element that forms the polymer backbone is a pentose sugar phosphate. pentose = a 5-carbon sugar, carbons numbered 1’, 2’, 3’, 4’ and 5’ (example, ribose, found in RNA) The two joining sites on the common element are: the 5’ phosphate. The acid in “nucleic acid”. Negative charge. and the 3’ OH (hydroxyl) This monomer polarity is reflected in the polymer, so we speak of the 5’-end and the 3’ end of a nucleic acid chain. Nucleic acid polymer growth is ALWAYS by addition of monomers to the 3’ end. DNA and RNA nucleotides are similar, but differ in the pentose sugar. Deoxyribose is missing the 2’ hydroxyl of ribose. Found in RNA Found in DNA Absence of 2’‐OH makes DNA much more resistant to chain cleavage by hydrolysis....greater stability! based on Fig 2‐16 of Lodish et al 9th ed. The heterocyclic bases of nucleotides N‐glycosidic bond based on Figs 2‐16 and 2‐17 Lodish 9th ed. The heterocyclic bases of nucleotides Presence of T instead of U in DNA makes some chemical Found in Found damage easier to repair. RNA in DNA A very short (3 nucleotides) DNA molecule. Note polarity…a 5’ end and a 3’ end The link between adjacent nucleotides is a phosphodiester bond. A diester because the phosphate is joined in an ester linkage to the 5’ OH of the downstream nucleotide and in another ester linkage to the 3’ OH of the upstream nucleotide. Question: if this were a growing DNA chain, to which end would the next nucleotide be added? Now let’s turn to proteins, and their monomers, the amino acids The characteristic element is the amino acid side chain (R) R | H N - C - COOH | H The common element that forms the polymer backbone is a Only the L (not the D) stereoisomers carbon (the alpha carbon) linked of amino acids are used in protein to a COOH (carboxyl) group and synthesis. a NH (amino group). The two joining sites on the common element are: the amino (NH ) group R | H N - C - COOH | and the carboxyl (COOH) group) H This monomer polarity is reflected in the polymer, so we speak of the amino terminus and the carboxyl terminus of a protein. Protein polymer growth is ALWAYS by addition of monomers to the carboxyl (COOH) end. The side chains of the amino acids There are 20 different amino R acid side chains. | Their chemical properties define H N - C - COOH 2 three main classes of amino | acid H 1. Hydrophobic (8 amino acids) 2. Hydrophilic (includes acidic and basic side chains) (9 amino acids) See Fig 2‐14 Lodish et al 9th ed 3. Special (3 amino acids) For structures of amino acid side chains A very short (5 amino acids) protein molecule. 1 2 3 4 5 Based on Fig 3‐3b Lodish et al 9th ed Note polarity…an amino end and a carboxyl end. The link between adjacent amino acids is a peptide bond. Question: if this were a growing protein chain, to which end would the next amino acid be added? Monomers are “energized” in order to be incorporated into the growing polymer chain Nucleotide monomers are in the form of high-energy nucleoside triphosphates (NTPs) ribo ATP CTP GTP UTP deoxyribo dATP nucleoside monophosphate dCTP nucleoside diphosphate nucleoside triphosphate dGTP see Lodish 9th ed. Table 2‐3 for terminology dTTP The outer two phosphates are “kicked out” when the NTP is incorporated Into a growing nucleic acid chain. Monomers are “energized” in order to be incorporated into the growing polymer chain Amino acid monomers are in the form of high-energy amino acyl-tRNA esters. The tRNA molecule is “kicked out” when the next amino acid is Incorporated at the end of a growing protein chain. From Fig 5‐31 Lodish et al 9th ed Even energized monomers cannot join a growing chain by themselves. The linkage reaction is catalyzed by a specific enzyme. The enzyme is associated with a template biopolymer that directs the enzyme to incorporate the correct flavor monomer. biopolymer template enzyme DNA DNA DNA polymerase RNA DNA RNA polymerase protein mRNA ribosome RNA and protein usually exist as single polymer chains but DNA is usually double-stranded (duplex DNA) 3’ 5’ DNA strands held together by H-bonds between complementary bases. = Watson-Crick base pairs. DNA is generally in a right-handed helix termed “B” DNA The two DNA strands are antiparallel Fig 5‐3 Lodish et al 9th ed 5’ 3’ Base-pairs in interior of DNA structure can be “read” by DNA-binding proteins binding to grooves Sugar-phosphate backbones on outside. Base-pairs stacked on inside. DNA-binding proteins can make contact with base-pairs at the major or minor grooves and identify specific sequences without having to separate the strands. Duplex DNA strands can be separated and re-associated DNA strands held together by weak H-bonds. Breaking H-bonds (e.g., by heat) allows strands to separate. DNA strand separation = melting = denaturation Denatured DNA strands can accurately re-form base-paired duplex DNA by formation of H-bonds between complementary base-pair sequences. = renaturation DNA denaturation and renaturation is important during biological processes such as DNA replication and transcription. It is also exploited in many experimental techniques in molecular biology and genomics. DNA denaturation and Tm the temperature at which the 1.0 DNA is one-half melted is the Tm. The Tm of DNA depends on its base composition. 0.5 DNA with a higher proportion of G-C base pairs has a higher Tm. modified from Fig 5.7 Lodish et al 9th ed changed X‐axis Tm is a function of G-C base-pair content G-C pair 3 H-bonds A-T pair 2 H-bonds It takes less energy to separate an A-T base-pair than to separate a G-C base-pair DNA can bend about its long axis Important in DNA-protein interactions and in the folding of DNA into compact condensed structures Fig 5‐5 Lodish et al 9th ed