Prokaryotic DNA-binding Proteins PDF
Document Details
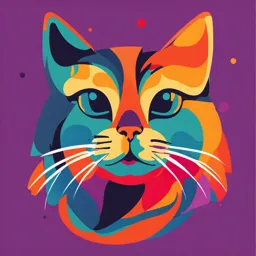
Uploaded by HumaneOcean
Friedrich-Alexander-Universität, Medizinische Fakultät
2024
Annika Gromann, Elischka Schumacher
Tags
Summary
This presentation is about prokaryotic DNA-binding proteins. It covers the architecture of biopolymers and how DNA is recognized in prokaryotes by helix-turn-helix motifs. It goes on to examine the structure and function of important proteins like lambda Cro and repressor proteins.
Full Transcript
Prokaryotic DNA- binding Proteins Seminar Architecture of Biopolymers Annika Gromann, Elischka Schumacher 11.11.2024 Part 1: DNA Recognition in Procaryotes by Helix-Turn-Helix Motifs Annika Gromann https://biodraws.com/helix-loop-helix-motif/ M...
Prokaryotic DNA- binding Proteins Seminar Architecture of Biopolymers Annika Gromann, Elischka Schumacher 11.11.2024 Part 1: DNA Recognition in Procaryotes by Helix-Turn-Helix Motifs Annika Gromann https://biodraws.com/helix-loop-helix-motif/ Medizinische Fakultät 11. November 2024 2 Prokaryotic DNA-Binding Proteins and Gene Regulation Key regulators of gene expression in procaryotes: Recognize specific DNA sequences to control gene activity Structural motifs, like helix-turn-helix (HTH), enable DNA binding Lambda phage genetic switch as a model for DNA recognition genetic switch https://www.researchgate.net/publication/225987979_Mutual_mobile_membranes_with_objects_on_surface/figures?lo=1 Medizinische Fakultät 11. November 2024 3 The Genetic Switch in Bacteriophage Lambda Regulatory Regions in the Lambda Phage Genetic Switch gene for repressor operator region gene for Cro DNA OR3 OR2 OR1 repressor promoter Cro promoter Created with BioRender Medizinische Fakultät 11. November 2024 4 The Genetic Switch in Bacteriophage Lambda Switch Between Lysogenic and Lytic Cycle Lysogenic state RNA-Polymerase OR3 OR2 repressor gene Cro gene OR1 RNA repressor protein Created with BioRender Medizinische Fakultät 11. November 2024 5 The Genetic Switch in Bacteriophage Lambda Switch Between Lysogenic and Lytic Cycle Lysogenic state RNA-Polymerase OR3 OR2 repressor gene Cro gene OR1 RNA repressor protein Lytic state RNA-Polymerase repressor gene Cro gene OR3 OR2 OR1 Cro protein RNA Created with BioRender Medizinische Fakultät 11. November 2024 6 Structure of Lambda Cro Protein Cro Subunit: Consists of a 66 amino acid long single polypeptide chain Folds into by 3 α-helices and 3 β-sheets Loop is formed between β-strands 1 and 2 is formed by α-helices Helix-turn-helix motif is formed by α-helix 2, 16 amino acids and α-helix 3 Cro Dimer: Cro molecules form dimers in solution and crystal structure Monomers are connected by their β-sheets The 3 β-strands of each subunit align and form a 6-stranded antiparallel β-sheet Medizinische Fakultät 11. November 2024 7 Structure of Lamda Repressor Protein Repressor subunit: Consists of 236 amino acids N-terminal domain binds DNA and consists of 5 α-helices connected by loops Helix-turn-helix motif is formed by α-helix 2, 19 amino acids residues and α-helix 3 C-terminal domain is formed by α-helix 5 and responsible for dimer formation by connecting the subunits Repressor dimer: UV light induces proteolytic cleavage of the C-terminal domain from the N-terminal domain Separation of the domains initiates genetic switch Medizinische Fakultät 11. November 2024 8 The Helix-Turn-Helix (HTH) Motif in DNA Recognition Helix-Turn-helix Motif = α-helical region followed by a sharp β-turn and then another α-helical region Helix-turn-helix motif provides recognition α-helix and stabilization helix Recognition α-helices of subunits are separated by 34Å Recognition α-helices bind in the major groove of the DNA N stabilization 34Å helix helix C helix turn recognition helix https://encrypted-tbn0.gstatic.com/images?q=tbn:ANd9GcQ0aqpK5uHBwDnP__5ECtIhYazHc_KcALcz4w&s, modified Medizinische Fakultät 11. November 2024 9 DNA Binding Mechanism of the HTH Motif Specific Interactions Between HTH Motifs and DNA Base Pairs Palindromic operator regions allow effective protein binding Each subunit binds to almost identical halves of the palindrome Identical bases in the first three base pairs create a general recognition site for ORs Operation regions of bacteriophage 434 Modified: 1999 Garland Publishing INC. Residues of recognition α-helix interact with DNA base pairs: Base Pair 1 (T14'-A1): GIn (Q) 28 forms hydrogen bonds with adenine Base Pair 2 (G13'-C2): Gln (Q) 29 forms a hydrogen bond with guanine Base Pair 3 (T12'-A3): Gln (Q) 29 forms a hydrogen bond with guanine and interacts with the methyl groups of Thr (T) 27 ➔ GIn 29 is essential for specificity as it interacts with base pairs 2 and 3 Medizinische Fakultät 11. November 2024 10 Differential DNA Binding by Repressor and Cro Proteins Protein-DNA interactions determine DNA conformation Binding of the protein dimer induces structural changes in B-DNA DNA sugar-phosphate interactions stabilize these changes Protein subunits anchor in the major groove through hydrogen bonds with DNA phosphate groups Protein loops extend into the minor groove, making additional contacts with phosphates Differential binding of Repressor and Cro Affinity for base pair 4: Repressor prefers A-T, Cro is indifferent to A-T or G-C Sequence of central base pairs: A -T pairs fit better in the narrowed minor groove than G-C pairs Medizinische Fakultät 11. November 2024 11 HTH Motifs in Prokaryotes Broad Impact on Gene Control Lac repressor molecule, binding into two successive major grooves of the DNA. Structure of the CAP-cyclic AMP-DNA complex. Medizinische Fakultät 11. November 2024 12 Take home messages DNA Recognition in Procaryotes by Helix-Turn-Helix Motifs https://dccdn.de/www.doccheck.com/data/mp/re/nq/v6/2t/bg/hom_odom_ne_md.jpg The helix-turn-helix motif includes a recognition α-helix that binds in the major groove of B-DNA and a stabilization α-helix, connected by a short turn. Subunit interactions ensure the correct distance of 34Å and orientation between the dimer's recognition α-helices, increasing affinity between protein and operator DNA Sequence-specific interaction between the recognition α-helix and DNA facilitate identification of the Helix-turn-helix motif, bound to DNA palindromic operator region. Hydrogen bonds between sugar phosphate backbone and the protein, along with DNA distortion, allow for close interaction between operator regions and DNA- binding proteins, influencing differential binding affinities for Cro and repressor Medizinische Fakultät 11. November 2024 13 Part 2: TetR – Structure and induction by tetracycline Comparison of TetR and LacI Elischka Schumacher Medizinische Fakultät 11. November 2024 14 Tetracycline induces resistance mechanism in gram- negative bacteria [MgTc]+: complex under physiological a conditions TetA: protein in cytoplasmic membrane → exports [MgTc]+ TetR: repressor protein tetO: operator DNA bound by TetR b a Chemical structure of the tetracycline-Mg2+ complex occuring under physiological conditions. b Sequence of the 15 base pair tet-operator used for co-crystallization. The boxes refer to the palindromic symmetry of tetO. Medizinische Fakultät 11. November 2024 15 Structure of TetR-tetO complex X-ray method, 2.5 Å resolution TetR: Helix-turn-helix Homodimer motif Blue: α1, α2, α3 → DNA-binding domain, HTH-motif (α2 and α3) Yellow: α5, α8, α10 → part of regulatory domain; “rigid scaffold“, tunnel-like ligand binding pocket Green: α4, α6, α7, α9 → part of regulatory domain; conformational change upon ligand binding α4 → linker between both domains tetO: Crystal structure of the TetR-tetO complex at 2.5 Å resolution. PDB accession code: 1QPI. Red: DNA-backbone, gray: bases Gray line: curvature of DNA Medizinische Fakultät 11. November 2024 16 Sequence specific interactions HTH-motif binds major groove of palindromic operator. All base pairs (except 0) contribute to the sequence specific interactions between repressor and operator Each HTH-motif binds major groove of palindromic half-operator Kink at base pair 2 → widening of palindromic major groove Visualization of the interaction of one DNA-binding domain of TetR and the tetO half-operator. Medizinische Fakultät 11. November 2024 17 Sequence specific interactions High structural complementary at recognition interface. Kink due to hydrogen bonds → unstacking a Unstacking stabilized by sequence specific hydrogen bonds (G2-Arg28; A3-Gln38) b Bending of base pairs 3-6 towards TetR due to further hydrogen bonds → compensating kink No overall DNA-bending No H2O in the DNA-protein interface → high structural complementary and high entropy term a Schematic representation of the interactions between one DNA-binding domain of TetR and the tetO half-operator. b Electron density of specific interactions between bases of tetO and amino acids of the HTH-motif. Medizinische Fakultät 11. November 2024 18 Induction by tetracycline and Mg2+ Conformational change occurs thoughout the entire protein. Ring A hydrogen bonds certain amino acids → anchor residues Mg2+ displaces His100 and Thr103 (α6) In parallel: whole α6 is shifted in its C- terminal direction undergoing conformational change (forms β-turn) Inducer binding site with tetracycline and Mg2+ and respective conformational changes. Medizinische Fakultät 11. November 2024 19 Induction by tetracycline and Mg2+ Conformational change occurs thoughout the entire protein. Van-der-Waals contact between α6 and α4 → central part of α4 shifts in same direction BUT: C-terminus of α4 is fixed (His64 anchored to ring A) → only N-terminus swings in pendulum-like motion by 5° Relative separation of α3 and α3´ along major groove by 3 Å → Dissociation of TetR-tetO complex upon Tc binding Visualization of conformational changes upon inducer binding (changes occur blue to yellow). Medizinische Fakultät 11. November 2024 20 Comparison of TetR to LacI Similarities a b a a a Schematic representation of the LacI operon upon repression and induction. b Schematic representation of the TetR operon upon repression and induction. 1. Dimers (LacI tetramer; dimer of dimers) 2. Two separate domains for DNA-binding and inducer-binding 3. HTH-motif interacts with major grooves of symmetrical DNA sequence 4. Rigid region in ligand binding domain (“rigid scaffold“) → no conformational change upon ligand binding Medizinische Fakultät 11. November 2024 21 Comparison of TetR to LacI Differences a TetR LacI Structure of Kink with Hinge helices also operator DNA compensating insert in minor bending → no grooves → overall overall DNA DNA bending of 45° bending + water mediated contacts b Inducer binding Tunnel, Mg2+ Cleft between two sites necessary subdomains (β-1,6- allolactose) Conformational Conformational Rotation and change change throughout translation of N- entire protein subdomain Shifting apart of α3 Unfolding of hinge helices helices → free a Schematic representation of the DNA–bound forms of TetR and LacI. HTH-motif b Schematic representation of the inducer-bound forms of TetR and LacI. Medizinische Fakultät 11. November 2024 22 Comparison of TetR to LacI Structure-function correlation: structures define affinity that is required due to respective function a Different reductions in affinity upon inducer binding: TetR: ~109; LacI: ~105 Structural explanation: LacI hinge helices need folding and whole DNA bending occurs → lower binding energy compared to TetR b Functional explanation: Lethality of TetA vs. effective use of environmental ressources by lac enzymes (low level of transcription necessary) → Level of repression depends on function of regulated genes a Schematic representation of the DNA–bound forms of TetR and LacI. b Schematic representation of the inducer-bound forms of TetR and LacI. Medizinische Fakultät 11. November 2024 23 Take home messages DNA Recognition in Procaryotes by Helix-Turn-Helix Motifs https://dccdn.de/www.doccheck.com/data/mp/re/nq/v6/2t/bg/hom_odom_ne_md.jpg The helix-turn-helix motif includes a recognition α-helix that binds in the major groove of B-DNA and a stabilization α-helix, connected by a short turn. Subunit interactions ensure the correct distance of 34Å and orientation between the dimer's recognition α-helices, increasing affinity between protein and operator DNA Sequence-specific interaction between the recognition α-helix and DNA facilitate identification of the Helix-turn-helix motif, bound to DNA palindromic operator region. Hydrogen bonds between sugar phosphate backbone and the protein, along with DNA distortion, allow for close interaction between operator regions and DNA- binding proteins, influencing differential binding affinities for Cro and repressor Medizinische Fakultät 11. November 2024 24 Take-home messages Tetracycline induces expression of tetA by binding to TetR and causing conformational change Structure of TetR Homodimer; 10 α-helices per polypeptide chain Two separate domains: DNA-binding domain (with HTH-motif) and regulatory domain ([MgTc]+ binding pocket) TetR-tetO interactions are sequence specific and of high structural complementary Interactions due to specific hydrogen bonds between TetR and tetO; no H2O in DNA-protein interafce No overall DNA bending (kink at base pair 2 is compensated) Medizinische Fakultät 11. November 2024 25 Take-home messages Induction by tetracycline and Mg2+ leads to the dissociation of the TetR-tetO complex Conformational changes thoughout the entire protein α4 swings in a pendulum-like motion → distance between recognition helices (α3 and α3´) is widened by ca. 3 Å → Disruption of the DNA-binding domain-half operator contacts → dissociation of TetR-tetO complex Shared features of TetR and LacI repressors Dimers (LacI dimer of dimers) separate domains for DNA- and inducer-binding: HTH-motif interacts with symmetrical DNA sequence, rigid region in ligand-binding domain Distinct LacI features Overall DNA-bending (45°) upon repression Induction by β-1,6-allolactose: unfolding of hinge helices → free HTH-motif Lower affinity to operator DNA compared to TetR Medizinische Fakultät 11. November 2024 26 Structure of TetR Crystal structure of TetR homodimer bound to the operator DNA tetO (resolution: 2.5 Å; PDB accession code: 1QPI). Blue: DNA-binding domains (α1-α3). Yellow: Rigid scaffold of regulatory domains (α5, α8, α10). Green: Helices of regulatory domains undergoing conformational change upon induction (α4, α6, α7, α9). Red: DNA-backbone. Gray: bases. Gray line: Curvature of DNA. Highlighted by the red circles are the helix-turn-helix-motifs (α2-α3) directly interacting with the respective major grooves of the palindromic sequences within tetO. Medizinische Fakultät 11. November 2024 27 References Branden, C.I., & Tooze, J. (1998). Introduction to Protein Structure (2nd ed.). Garland Science. https://doi.org/10.1201/9781136969898 Orth, P et al. “Structural basis of gene regulation by the tetracycline inducible Tet repressor- operator system.” Nature structural biology vol. 7,3 (2000): 215-9. doi:10.1038/73324. Matthews, K S et al. “Relieving repression.” Nature structural biology vol. 7,3 (2000): 184-7. doi:10.1038/73274. LaPlante, Kerry L et al. “Re-establishing the utility of tetracycline-class antibiotics for current challenges with antibiotic resistance.” Annals of medicine vol. 54,1 (2022): 1686-1700. doi:10.1080/07853890.2022.2085881. Maher, L James 3rd. “DNA kinks available...if needed.” Structure (London, England : 1993) vol. 14,10 (2006): 1479-80. doi:10.1016/j.str.2006.09.001. Marbach, Anja, and Katja Bettenbrock. “lac operon induction in Escherichia coli: Systematic comparison of IPTG and TMG induction and influence of the transacetylase LacA.” Journal of biotechnology vol. 157,1 (2012): 82-8. doi:10.1016/j.jbiotec.2011.10.009. Medizinische Fakultät 11. November 2024 28 Thank you for your attention!