Introduction to Metabolism PDF
Document Details
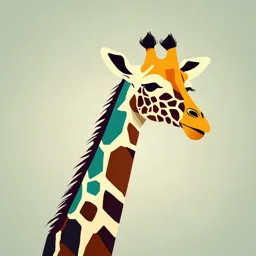
Uploaded by CharismaticMridangam
Griffith University
Sandra Ramos
Tags
Summary
This document from Griffith University provides an introduction to metabolism, covering catabolism, anabolism, and glucose homeostasis. It discusses different aspects, including the fates of glucose, glycogenolysis, gluconeogenesis, and the citric acid cycle.
Full Transcript
Introduction to Metabolism Sandra Ramos (Dr. Dianne Moses reviewed) [email protected] Metabolism = catabolism + anabolism Metabolism has 4 functions 1. Obtain energy in a chemical form by the degradation of nutrients 2. Convert wide range of nutrients precursors required to build proteins, CHO...
Introduction to Metabolism Sandra Ramos (Dr. Dianne Moses reviewed) [email protected] Metabolism = catabolism + anabolism Metabolism has 4 functions 1. Obtain energy in a chemical form by the degradation of nutrients 2. Convert wide range of nutrients precursors required to build proteins, CHO, lipids, DNA 3. Synthesise cell molecules 4. Produce or modify biomolecules necessary for specific functions in cells Catabolism is broken into 3 stages STAGE 1 Nutrient molecules broken down to amino acids, fatty acids, monosaccharides and glycerol STAGE 2 Conversion of products into one simple molecule Acetyl-s coenzyme A (Acetyl CoA) Monosaccharides → 3C Pyruvate → 2C Acetyl CoA Fatty acids and most amino acids are converted directly into Acetyl CoA. STAGE 3 Acetyl CoA enters the citric acid cycle Oxidised to CO2 + H2O Releases NADH and FADH2, ATP NADH and FADH2 are oxidized by the electron transport chain Reduction of O2 → H2O Generates of ATP ATP – energy currency Ion transport Muscle contraction Nerve impulse propagation Substrate phosphorylation Chemical synthesis Glucose homeostasis Glucose must be maintained at 3.9 – 5.8 mM Erythrocytes, brain Regular supply of glucose through dietary intake about every 4-6 hours in humans Management of internal glucose stores Glycogen metabolism Gluconeogenesis Glycolysis Fates of glucose Intracellular glucose - Catabolised via glycolysis Stored as glycogen in liver and skeletal muscle (liver stores ~ 30% of total glycogen and muscle stores ~ 150g for use during exercise); once glycogen stores are full excess glucose may be converted to fat - Pentose phosphate pathway When no carbohydrates are available glucose can be made from other substrates Gluconeogenesis Glucose catabolism Complex carbohydrates are broken down to monosaccharides Most important is glucose Glycolysis is a series of 10 reactions that changes the 6C glucose to 2 molecules of the 3C pyruvate Takes place in the cytosol of cells Energy investment 2 ATP is required Energy generation 4 ATP + 2 NADH Overall NET Energy Yield 2ATP + 2 NADH Summary of glycolysis What do you need to know? Intermediates Enzymes Cofactors How it works! Regulation of glycolysis The process is regulated by controlling the rate of three enzymes: - hexokinase (HK) - phosphofructokinase (PFK-1) - pyruvate kinase (PK) Inhibited by ATP – regulated by demand for ATP Glycolysis is also regulated by: 1) Supply of glucose 2) Removal of pyruvate 3) Need for metabolic intermediates 4) Activity of Citric Acid Cycle 5) Amount of fat metabolism 6) Physiological actions of hormones Clinical Significance Hereditary Hemolytic Anemia May be associated with a genetic defect of adult erythrocyte Pyruvate Kinase that catalyses Step 10 of Glycolysis Kinase is virtually inactive Erythrocytes of affected individuals have a greatly reduced capacity to make ATP Reduced ability to perform activities such as ion pumping and the maintenance of osmotic balance Erythrocytes have a short half-life and readily lyse Treatment blood transfusions, medicines, plasmapheresis, surgery (splenectomy), blood and marrow stem cell transplants Glycogenolysis Liver Glucose-1-Phosphate converted to glucose Release free glucose into blood keep levels at approx. 4.5mM Muscle Does not contain Glucose-6-phosphatase Cannot export glucose to the blood Glucose used for energy Lactate/pyruvate can be exported for gluconeogenesis in liver a(1-4) bonds broken by Phosphorylase-b releases Glucose-1-P (approx 90%) a(1-6) bonds broken by Glucosidase releases Glucose (approx 10%) Glycogenolysis Regulation Hormonal inhibition of glycogen synthase Glucagon Adrenaline (muscle) Non hormonal Low energy = Accumulation of AMP Stimulates glycogen phosphorylase b Increases glycogen breakdown Gluconeogenesis 3 Synthesis of new glucose 2 The liver is the major site for glucose synthesis, but kidney also involved Bypass 1 Pyruvate is carboxylated to oxaloacetate by pyruvate carboxylase Only mitochondria have this enzyme so this step occurs in mitochondria Bypass 2 Oxaloacetate leaves mitochondria as malate The phosphate group is removed by fructose-1,6-bisphosphatase. Bypass 3 The removal of the phosphate group from glucose-6-phosphate (smooth endoplasmic reticulum) 1 Substrates for gluconeogenesis Glycerol Release of stored triglycerides Fatty acids for energy Glycerol for gluconeogenesis Lactate Anaerobic metabolism Cori cycle Amino Acids Glucogenic amino acids Deaminated/transaminated Propionyl CoA 3 Carbon remnant from odd chained fatty acid oxidation Pyruvate The fate of pyruvate depends on the oxidation state of the cell – During aerobic glycolysis when O2 is plentiful Pyruvate enters mitochondria Oxidised to Acetyl CoA by pyruvate dehydrogenase citric acid cycle NADH is oxidised by the electron transport chain to yield ATP Pyruvate + NAD+ + CoA-SH → Acetyl CoA + CO2 + NADH + H+ – During anaerobic glycolysis when O2 is limited NADH can not be oxidised in mitochondria (shuts down Pyruvate Dehydrogenase) NADH used to drive the reduction of pyruvate to lactate Catalysed by lactate dehydrogenase Glucose + 2ATP + 2NAD+ → 2 Pyruvate + 4ATP + 2 NADH 2 Pyruvate + 2 NADH → 2 Lactate + 2 NAD+ NET ENERGY = 2 ATP Lactate and the Cori Cycle Anaerobic glycolysis produces lactate In muscle Released into blood Taken up by liver Converted back to glucose Released back into blood Taken up by muscle Clinical significance Lactic acidosis: Most common cause is impairment of oxidative metabolism e.g. respiratory failure, insufficient oxygen transport, toxins preventing oxidative phosphorylation Without oxygen mitochondria cannot oxidise NADH to NAD+ The accumulating NADH makes LDH reaction irreversible in direction of lactate formation Other causes: Drowning, severe anaemia, carbon monoxide poisoning, cyanide poisoning, alcohol intoxication, Von Gierke Disease, Pyruvate dehydrogenase deficiency, Leukemia, metastatic carcinoma Production of Acetyl CoA Acetyl CoA is the common intermediate in aerobic respiration is obtained from: 1. Triglyceride metabolism Beta Oxidation of Fatty Acids Producing Acetyl CoA 2. Protein Metabolism Transamination of Amino Acids Producing Acetyl CoA directly or other intermediates such as Pyruvate 3. Carbohydrate metabolism Glycolysis Producing Pyruvate Citric acid cycle – krebs cycle Occurs in the matrix of the mitochondria 2 carbons in the form Acetyl CoA enter the citric acid cycle, 2 Carbons leave as CO2 Produces: 3 NADH + 1 FADH + 1 GTP (ATP) Oxaloacetate is regenerated to begin another turn of the cycle. The cycle will make two turns for each molecule of glucose as each glucose molecule produces 2 acetyl CoA Inhibited by: NADH, H+, ATP Stimulated by: NAD+, ADP, AMP Clinical significance Deficiencies of citric acid cycle enzymes are rare Fumarase Deficiency – Characteristics appear soon after birth Severe neurological impairment Encephalomyopathy Dystonia – Urine analysis indicates Elevated fumarate, succinate, α-ketoglutarate and citrate – Autosomal recessive disorder Parents asymptomatic with half normal enzyme activity Energy yield so far Electron transport chain Oxidation of NADH results in the formation of ATP – previously ATP was generated by substrate phosphorylation Stored electrons in the form of NADH or FADH2 pass through sequence of enzymes and cofactors in the inner mitochondrial membrane – Finally react with O2 to form H2O During electron transport, a proton gradient forms across the inner mitochondrial membrane – Drives ATP synthesis 1 x NADH produces 2.5 ATP 1 x FADH2 produces 1.5 ATP The coupling of electron transport and ATP synthesis is mandatory Chemiosmotic theory The pumping of H+ at complexes I, III and IV establishes a 1. pH gradient pH outside the membrane becomes lower than the pH inside the matrix 2. Electrical potential across the membrane These conditions attract protons back into the matrix The protons re-enter the matrix through Complex V ATP synthase Flow of protons down the electrochemical gradient is energetically favourable causing the synthesis of ATP Energy yield 2 CO2 4 CO2 28 ATP ETC regulation Inhibited by: – Lack of ADP as P acceptor (acceptor control) – Lack of O2 as terminal electron acceptor http://au.youtube.com/watch?v=StXlo1W3Gvg&feature=related – Lack of reducing equivalents NADH & FADH2 – Reduction in the integrity of the inner mitochondrial membrane Prevents electrochemical gradient to be established Accumulation of NADH as a consequence of inactive electron transport will inhibit TCA and the formation of Acetyl CoA Clinical significance The electron transport chain and ATP synthase must be coupled Inhibitor of complex I: Rotenone (inhibits reduction of Coenzyme Q) Inhibitors of Complex IV: CO: competitively binds to reduced heme in cytochrome a3, preventing electron transfer to O2 Cyanide: binds cytochrome a3 and prevents electron transfer to O2 Inherited mitochondrial disorders: Should be strongly suspected in patients with muscle disease and involvement of 2 additional systems OR CNS disease and involvement of 2 additional systems OR Multisystem disease which may include muscle and CNS E.g. Alpers syndrome, MELAS syndrome, Kearns-Sayre syndrome Uncoupling of ETC Agents disrupt the tight coupling of the electron transport and ATP synthesis – Act by displacing the proton gradient across the inner mitochondrial membrane. Carry protons back across the membrane into the matrix Leakage disrupts the gradient as pumping is not sufficient to overcome the leakage ATP synthesis STOPS Uncouplers of ETC https://www.sciencephoto.com/media/610064/view/dinitrophenol-inhibiting-atp-production Natural uncoupler called thermogenin found in brown fat deposits is important in maintaining body temperature Summary of lipid catabolism Lipids are digested and adsorbed differently due to hydrophobicity Lipids are transported to tissues as lipoproteins Fatty acids are transported to cells with albumin Fatty acids are activated and transported to mitochondrial matrix 𝛽-Oxidation Ketogenesis 𝛽-oxidation – energy produced Lipogenesis Synthesis of fatty acids occurs primarily in the cytosol of liver & adipocytes – superficially resembles the reverse of β oxidation – carbon from glucose converted to triglycerides – packaged in very low density lipoproteins (VLDL) for transport to adipose tissue Insulin increases the uptake of circulating fatty acids by adipocytes for storage as triacylglycerol Self-study for details Protein metabolism - turnover Proteins must be broken down and replaced before they deteriorate (400g/day). Functional and structural roles 1. Amino acids are recycled from broken down body protein 2. Ingested proteins are used to replace tissue proteins (~ 100g/day) Liver primary site for uptake following meal (stimulated by insulin) then join the general circulation – some promptly recycled into other proteins – excess is stripped of nitrogen & used for energy or converted to fat Protein metabolism - summary Nitrogen Balance: – body maintains the same amount of protein in its tissues day to day – excess nitrogen must be excreted Protein metabolism - summary All then end up in the citric acid cycle Ketogenic amino acids Produce ketone bodies Degraded to acetoacetyl CoA or acetyl CoA Oxidized in the citric acid cycle Leucine and Lysine Glucogenic amino acids Degraded to pyruvate, α-ketoglutarate, succinyl CoA, fumarate or oxaloacetate Oxidized in the citric acid cycle Used for glucose synthesis Used for synthesis of other metabolites All AA can be oxidized to generate energy; NOT all used for gluconeogenesis Principles of Biochemistry – Lehninger, Fifth edition Clinical relevance Congenital disorder due to Phenylalanine transaminase deficiency: 1) Phenylketonuria (PKU) Amboss