Welding Inspection Technology PDF
Document Details
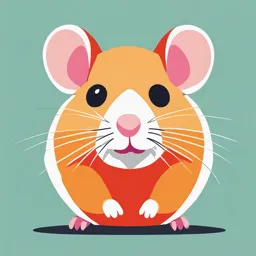
Uploaded by BestPerformingPlateau
Tags
Summary
This document provides information on welding inspection technology, specifically focusing on chapter 3: Metal Joining and Cutting Processes. It details various welding techniques such as submerged arc welding (SAW) and includes examples.
Full Transcript
WELDING INSPECTION TECHNOLOGY CHAPTER 3—METAL JOINING AND CUTTING PROCESSES Indicates flux Indicates the minimum tensile strength, in increments of 10 000 psi [69 MPa] of weld metal made in accordance with the welding conditions given, and using the flux being classified and the specific classific...
WELDING INSPECTION TECHNOLOGY CHAPTER 3—METAL JOINING AND CUTTING PROCESSES Indicates flux Indicates the minimum tensile strength, in increments of 10 000 psi [69 MPa] of weld metal made in accordance with the welding conditions given, and using the flux being classified and the specific classification of electrode indicated. Designates the condition of heat treatment in which the tests were conducted: •A• for aswelded and •P• for postweld heat treated. The time and temperature of the PWHT are as specified. Indicates the lowest temperature at which the impact strength of the weld metal referred to above meets or exceeds 20 ft·lb [27J]. E indicates a solid electrode; EC indicates a composite electrode. L (low), M (medium), or H (high) manganese content, or C (composite electrode) FXXX-EXXX Classification of the electrode used in producing the weld referred to above. Examples F7A6-EM12K is a complete designation. It refers to a flux that will produce weld metal which, in the as-welded condition, will have a tensile strength no lower than 70 000 psi [480 MPa] and Charpy V-notch impact strength of a least 20 ft·lb [27 J] at –60°F [–51°C] when produced with an EM12K electrode under the conditions called for in this specification. F7A4-EC1 is a complete designation for a flux when the trade name of the electrode used in the classification is indicated as well. It refers to a flux that will produce weld metal with that electrode, which in the as-welded condition, will have a tensile strength no lower than 70 000 psi [480 MPa] and Charpy V-notch of at least 20 ft·lb [27 J] at –40°F [–40°C] under the conditions called for in this specification. Figure 3.25—SAW Filler Metal Identification System Figure 3.26—Submerged Arc Welding Equipment 3-21 CHAPTER 3—METAL JOINING AND CUTTING PROCESSES WELDING INSPECTION TECHNOLOGY rate of weld metal deposition, it has shown to be quite effective for overlaying or building up material surfaces. In situations where a surface needs improved corrosion or wear resistance, it is often more economical to cover a susceptible base metal with a resistant weld overlay. If this application can be mechanized, submerged arc welding is an excellent choice. Probably the biggest advantage of SAW is its high deposition rate. It can typically deposit weld metal more efficiently than any of the more common processes. The submerged arc welding process also has high operator appeal because of the lack of a visible arc which allows the operator to control the welding without the need for a filter lens and other heavy protective clothing. The other beneficial feature is that there is less smoke generated than with some of the other processes. Another feature of the process which makes it desirable for many applications is its ability to penetrate deeply. The major limitation of SAW is that it can only be done in a position where the flux can be supported in the weld joint. When welding in a position other than the normally-used flat or horizontal fillet positions, some device is required to hold the flux in place so it can perform its job. Another limitation is that, like most mechanized processes, there may be a need for extensive fixturing and positioning equipment. As with other processes using a flux, finished welds have a layer of solidified slag which must be removed. If welding parameters are improper, weld contours could be such that this job of slag removal is even more difficult. Figure 3.27—Semiautomatic Submerged Arc Welding Equipment The final disadvantage relates to the flux which covers the arc during welding. While it does a good job of protecting the welder from the arc, it also prevents the welder from seeing exactly where the arc is positioned with respect to the joint. With a mechanized setup, it is advisable to track the entire length of the joint without the arc or the flux to check for alignment. If the arc is not properly directed, incomplete fusion can result. The flux must be moved to the weld zone; for mechanized systems, the flux is generally placed into a hopper above the welding torch and fed by gravity so that it is distributed either slightly ahead of the arc or around the arc from a nozzle surrounding the contact tip. In the case of semiautomatic submerged arc welding, the flux is forced to the gun using compressed air which “fluidizes” the granular flux, causing it to flow easily, or there is a hopper connected directly to the hand-held gun. There are some inherent problems related to SAW. The first has to do with the granular flux. Just as with low hydrogen SMAW electrodes, it is necessary to protect the submerged arc welding flux from moisture. It may be necessary to store the flux in heated containers prior to use. If the flux becomes wet, porosity and underbead cracking may result. Another equipment variation is the choice of alternating or direct current, either polarity. The type of welding current will affect both penetration and weld bead contour. For some applications, multiple electrodes can be used. The electrodes may be energized by a single power source, or multiple power sources may be necessary. The use of multiple electrodes provides even more versatility for the process. Another characteristic problem of SAW is solidification cracking. This results when the welding conditions produce a weld bead having an extreme width-to-depth ratio. That is, if the bead width is much greater than its depth, or vice versa, centerline shrinkage cracking can occur during solidification. Figure 3.28 shows some conditions which can cause cracking. SAW has found acceptance in many industries, and it can be performed on numerous metals. Due to the high 3-22 WELDING INSPECTION TECHNOLOGY CHAPTER 3—METAL JOINING AND CUTTING PROCESSES (B) (A) (D) (C) (E) Figure 3.28—Solidification Cracking Because of Weld Profile Plasma Arc Welding (PAW) torches and the resulting difference in the amount of heating, and therefore penetration, which will occur. The next process to be discussed is plasma arc welding. Plasma is defined as an ionized gas. With any process using an arc, plasma is created. However, PAW is so named because of the intensity of this plasma region. At first glance, PAW could be easily mistaken for GTAW because the equipment required is quite similar. A typical setup is shown in Figure 3.29. Both the PAW and GTAW torches use a tungsten electrode for the creation of the arc. However, with the PAW torch, there is a copper orifice within the ceramic nozzle. There is a “plasma” gas which is forced through this orifice and past the welding arc resulting in the constriction of the arc. This constriction, or squeezing, of the arc causes it to be more concentrated, and therefore more intense. One way to illustrate the difference in arc intensity between GTAW and PAW would be to use the analogy of an Both GTAW and PAW use the same type of power source. However, when we look closely at the torch itself, the difference becomes more obvious. Figure 3.30 shows a graphic comparison of the two types of welding 3-23 CHAPTER 3—METAL JOINING AND CUTTING PROCESSES WELDING INSPECTION TECHNOLOGY Constricting Nozzle Orifice Gas - Shielding Gas + Work + Transferred Nontransferred Figure 3.31—Transferred and Nontransferred PAW Comparison Figure 3.29—Plasma Arc Welding ferred arc, on the other hand, occurs between the tungsten electrode and the copper orifice. The transferred arc is generally used for both welding and cutting of conductive materials, because it results in the greatest amount of heating of the workpiece. The nontransferred arc is preferred for the cutting of nonconductive materials and for welding of materials when the amount of heating of the workpiece must be minimized. The similarities between GTAW and PAW extend to the equipment as well. The power sources are identical in most respects. However, as shown in Figure 3.32, there are some additional elements necessary, including the plasma control console and a source of plasma gas. Figure 3.30—Comparison of GTAW and PAW Torches The torch, as discussed above, does differ slightly; however, a careful check of the internal configuration must be made to be certain. Figure 3.33 illustrates some of the internal structure of a typical PAW torch for manual welding. adjustable water hose nozzle. The GTAW arc would be comparable to the gentle mist setting, while the PAW arc would behave more like the setting which provides a concentrated stream of water having a greater force. As indicated, two separate gases are required: the shielding gas and the orifice (or plasma) gas. Argon is most commonly employed for both types of gas. However, welding of various metals might warrant the use of helium or combinations of argon/helium or argon/hydrogen for one of the other gases. There are two categories of plasma arc operation, the transferred and nontransferred arc. They are shown in Figure 3.31. The primary applications for PAW are similar to those for GTAW. PAW is used for the same materials and thicknesses. PAW becomes the choice where applications warrant the use of a more localized heat source. It is With the transferred arc, the arc is created between the tungsten electrode and the workpiece. The nontrans- 3-24 WELDING INSPECTION TECHNOLOGY CHAPTER 3—METAL JOINING AND CUTTING PROCESSES Figure 3.32—Plasma Arc Welding Equipment, Including Control Console Back Cap Gasket + Pilot-Arc and Transfer-Arc Cable Coolant Outlet Orifice-Gas Inlet Torch Body Coolant Inlet − Pilot-Arc Cable Shielding Gas Inlet Tungsten Electrode Outer Shield Cup (Ceramic) Orifice Body (Copper) Figure 3.33—Internal Structure of a Typical Manual PAW Torch used extensively for full penetration welds in material up to 1/2 in thick by employing a technique referred to as “keyhole welding.” Figure 3.34 shows the typical appearance of a keyhole weld. Keyhole welding is performed on a square butt joint with no root opening. The concentrated heat of the arc penetrates through the material thickness to form a small keyhole. As welding progresses, the keyhole moves along Figure 3.34—Keyhole Technique for Plasma Arc Welding (Face—Top and Root—Bottom) 3-25 CHAPTER 3—METAL JOINING AND CUTTING PROCESSES WELDING INSPECTION TECHNOLOGY done in a single pass such that the progression is from the bottom to the top of the joint, without interruption. Even though the welding progresses vertically up the joint, the position of welding is considered flat due to the location of the electrode with respect to the puddle. During welding, the molten metal is supported on two sides by water cooled copper shoes (see Figure 3.35). the joint melting the edges of the base metal which then flow together and solidify after the welding arc passes. This creates a high quality weld, with no elaborate joint preparation and fast travel speeds compared to GTAW. One advantage of PAW, which was mentioned before, is that it provides a very localized heat source. This allows for faster welding speeds and therefore less distortion. Since the standoff used between the torch end and the workpiece is typically quite long, the welder has better visibility of the weld being made. Also, since the tungsten electrode is recessed within the torch, the welder is less likely to contact the molten metal and produce a tungsten inclusion. An interesting feature of ESW is that it is not considered to be an arc welding process. It relies on heating from the electrical resistance of the molten flux to melt the base and filler metals. The process does use an arc to initiate the operation; however, that arc is extinguished once there is sufficient flux melted to provide the heating to maintain the welding operation as it progresses upward along the joint. When the flux becomes molten, the current supplied to the wire causes resistance heating of the slag that makes it hot enough to melt the sidewalls. The filler wire melts off and combines with the side walls to make a weld. The slag, being lighter than the weld metal, stays on top of the weld and continues to melt the sidewalls as it progresses upward along the joint. The slag has to be replenished as the weld progresses. Consumable guide tubes that have flux on them can be used, or the operator can continually add flux to the top of the “melt.” The ability to use this process in a keyhole mode is also desirable. The keyhole is a positive indication of complete penetration and weld uniformity. This weld uniformity is in part due to the fact that plasma arc welding is less sensitive to changes in arc length. The presence of its collimated arc will permit relatively large changes in torch-to-work distance without any change in its melting capacity. PAW is limited to the effective joining of materials 1 in or less in thickness. The initial cost of the equipment is slightly greater than that for GTAW, primarily because there is additional apparatus required. Finally, the use of PAW may require greater operator skill than would be the case for GTAW due to the more complex equipment setup. ESW is used when very heavy sections are being joined. It is essentially limited to the welding of carbon steels in thickness greater than 3/4 in. So, only industries dealing with heavy weldments have any real interest in ESW. Figure 3.36 shows an ESW equipment setup. Among the problems that may be encountered with this process are two types of metal inclusions. Tungsten inclusions may result from too-high current levels; however, the fact that the tungsten is recessed helps to prevent this occurrence. Too-high current could also result in the copper orifice melting and being deposited in the weld metal. Another problem that may be encountered when keyhole welding is being done is referred to as tunneling. This occurs when the keyhole is not completely filled at the end of the weld, leaving a cylindrical void which may extend entirely through the throat of the weld. When using the keyhole technique, there is also a possibility of getting incomplete fusion since the arc and joint are so narrow. As a result, even small amounts of mistracking can produce incomplete fusion along the joint. Electroslag Welding (ESW) The next process of interest is electroslag welding, but it is not nearly as commonly used as the processes mentioned previously. It typically exhibits the highest deposition rate of any of the welding processes. ESW is characterized by the joining of members who are placed edge to edge so that the joint is vertical. The welding is Figure 3.35—Electroslag Welding 3-26 WELDING INSPECTION TECHNOLOGY CHAPTER 3—METAL JOINING AND CUTTING PROCESSES centerline cracks due to weld metal shrinkage. Also, due to the tremendous amount of heating, there is a tendency for grain growth in the weld metal. These large grains may result in degradation of the weldments’ mechanical properties. Control Panel Power Source Wire Reel - + Oxyacetylene Welding (OAW) Electrode Wire Feed Drive Electrode Lead The next process is oxyacetylene welding. While the term “oxyfuel welding” is also used, acetylene is the only fuel gas capable of producing high enough temperatures for effective welding of steel. With OAW, the energy for welding is created by a flame, so this process is considered to be a chemical welding method. Just as the heat is provided by a chemical reaction, the shielding for oxyacetylene welding is accomplished by this flame as well. Therefore, no flux or external shielding is necessary. Figure 3.37 illustrates the process being applied with filler metal added from some external source. Oscillation Workpiece Consumable Guide Molten Slag Bath Molten Weld Pool Workpiece Lead Solidified Weld Metal Retaining Shoe Water In The equipment for oxyacetylene welding is relatively simple. A typical setup is shown in Figure 3.38. It consists of several parts: oxygen tank, acetylene tank, pressure regulators, torch, and connecting hoses. The oxygen cylinder is a hollow, high pressure container capable of withstanding a pressure of approximately 2200 psi. The acetylene cylinder on the other hand, is filled with a porous material similar to cement. Workpiece Water Out Figure 3.36—Electroslag Welding Equipment Acetylene exists in the cylinder dissolved in liquid acetone. Care must be taken since gaseous acetylene is extremely unstable at pressures exceeding 15 psi and an explosion could occur even without the presence of oxygen. Since the acetylene cylinder contains a liquid it is important that it remains upright to prevent drawing off the liquid. The major advantage of ESW is its high deposition rate. If single electrode welding is not fast enough, then multiple electrodes can be used. In fact, metal strip can be used instead of wire to increase the deposition rate even more. Another benefit is that there is no special joint preparation required. In fact, a rough, flame cut surface is satisfactory for this method. Since the entire thickness of the joint is fused in a single pass, there is no tendency for any angular distortion to occur during or after welding, so alignment is easily maintained. Each cylinder has attached to its top a pressure regulator which reduces the high internal tank pressure to working The primary limitation of ESW is the extensive time required to set up and get ready to weld. There is a tremendous amount of time and effort required to position the workpieces and guides before any welding takes place. That is why ESW is not economical for thinner sections, even though the deposition rate is quite high. The ESW process has associated with it several inherent problems. When these problems arise, they can be of major proportions. Gross porosity can occur due to wet flux or the presence of a leak in one of the water cooled shoes. Since electroslag welding resembles a casting process in many respects, there is a possibility of getting Figure 3.37—Oxyacetylene Welding 3-27 CHAPTER 3—METAL JOINING AND CUTTING PROCESSES WELDING INSPECTION TECHNOLOGY detachable tip. Tips are made in a variety of sizes to allow welding of different metal thicknesses. The filler material used for OAW on steel has a simple identification system. Two examples are RG-45 and RG60. The “R” designates it as a rod, “G” stands for gas and the 45 and 60 relate to the minimum tensile strength of the weld deposit in thousands of pounds per square inch (psi). So RG 45 designates a weld deposit having a tensile strength of at least 45 000 psi. and RG 65 designates a weld deposit having a tensile strength of at least 65 000 psi. Although not used as extensively as it once was, OAW still sees some usage. Its primary tasks include the welding of thin steel sheet and small diameter steel piping. It is also applied in many maintenance situations as well. The advantages of OAW include some desirable features of the equipment itself. First, it is relatively inexpensive and can be made very portable. This portability relates not only to the compact size but also to the fact that there is no electrical input required. Care should be taken when moving the equipment so that the primary valves on the cylinders are not damaged. If broken off, a cylinder can turn into a lethal missile. So, whenever transported, the regulators should be removed and the valves covered with special screw-on caps for protection from impact. The process also has certain limitations. For one, the flame does not provide as concentrated a heat source as can be achieved by an arc. Therefore, if a groove weld is being made, the joint preparation should exhibit a thin “feather edge” to assure that complete fusion is obtained at the root of the joint. This lower heat concentration also results in a relatively slow process, so we typically consider OAW best suited for thin section welding. As with any of the welding processes requiring the filler metal to be fed manually, OAW requires a substantial skill level for best results. Figure 3.38—Oxyacetylene Welding Equipment There are certain inherent problems associated with OAW. They are primarily related to either improper manipulation or adjustment of the flame. Since the heat source is not concentrated, care must be taken to direct the flame properly to assure adequate fusion. If the flame is adjusted such that an oxidizing flame or carburizing flame is produced, weld metal properties could be degraded, so it is important to have equipment capable of providing uniform gas flow. pressures. Hoses then connect these regulators to the torch. The torch includes a mixing section where the oxygen and acetylene combine to provide the necessary mixture. The ratio of these two gases can be altered by the adjustment of two separate control valves. Normally, for carbon steel welding, they are adjusted to provide a mixture which is referred to as a neutral flame. A higher amount of oxygen will create an oxidizing flame and a higher amount of acetylene will produce a carburizing flame. After the gases are mixed, they flow through a Stud Welding (SW) The next welding process to be discussed is stud welding. This method is used to weld studs, or attachments, to some metal surface. SW is considered to be an arc weld- 3-28