Welding Inspection Technology PDF
Document Details
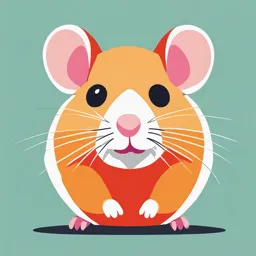
Uploaded by BestPerformingPlateau
Tags
Summary
This document discusses various metal joining and cutting processes, focusing on brazing techniques, including furnace, induction, resistance, dip, and infrared brazing. It also explores oxyfuel gas cutting and plasma arc cutting, their applications, and considerations for different metals. It includes tables and figures.
Full Transcript
WELDING INSPECTION TECHNOLOGY CHAPTER 3—METAL JOINING AND CUTTING PROCESSES Table 3.4 AWS Brazing Filler Metal Classifications Designation Primary Element BAlSi BCuP BAg BAu BCu RBCuZn BMg BNi Aluminum-Silicon Copper-Phosphorus Silver Gold Copper Copper-Zinc Magnesium Nickel heated. The most f...
WELDING INSPECTION TECHNOLOGY CHAPTER 3—METAL JOINING AND CUTTING PROCESSES Table 3.4 AWS Brazing Filler Metal Classifications Designation Primary Element BAlSi BCuP BAg BAu BCu RBCuZn BMg BNi Aluminum-Silicon Copper-Phosphorus Silver Gold Copper Copper-Zinc Magnesium Nickel heated. The most familiar method is referred to as torch brazing (TB) where heating is accomplished using an oxyfuel flame. It can be done either manually, mechanically or automatically. Other common heating methods include furnace, induction, resistance, dip, and infrared. Furnace brazing (FB) is performed in a furnace, often with a controlled atmosphere. The braze filler material and flux are preplaced at or near the joint and the parts to be joined are then placed in a furnace which heats them in a very controlled manner. FB can be used to produce numerous braze joints simultaneously once the assembly is brought to the brazing temperature. Figure 3.54—Placement of Brazing Preforms in Braze Joints (Red Shows Resulting Braze Zone) Induction brazing (IB) relies on the heat produced in a metal when placed within an induction coil. The induction coil is simply a coil through which a high frequency electric current is passed. This flow of electricity will produce substantial heating of a piece of metal placed inside the coil. Resistance brazing (RB) is accomplished by heating the base metal using its own inherent electrical resistance. When an electric current is passed through the base metals on either side of the braze joint, resistance heating occurs and melts the braze filler metal placed in the joint. Dip brazing (DB) differs from the other types in that the parts to be joined are immersed in some type of molten bath to provide the necessary heating. This bath can be either molten braze filler metal or some type of molten chemical, such as chemical salts. Infrared brazing (IRB) relies on heating provided by radiant energy. That is, the joint to be brazed is heated using a high intensity infrared light source. Brazing is used in many industries, especially aerospace and heating and air conditioning. It can be applied to join virtually all metals, and can also be used to join metals to nonmetals. Figure 3.55—Location of Braze Filler Material in Joint After the Application of Heat (Red Shows Resulting Braze Zone) 3-37 CHAPTER 3—METAL JOINING AND CUTTING PROCESSES WELDING INSPECTION TECHNOLOGY Table 3.5 AWS Brazing Flux Identification System (Partial Listing) Activity Temperature Range Classification Form Filler Metal Type FB1-A Powder BAISi Fluorides Chlorides For torch or furnace brazing. FB2-A Powder BMg Fluorides Chlorides FB3-A Paste BAg and BCuP Borates Fluorides FB4-A Paste Typical Ingredients BAg and Fluorides BCuP Chlorides Borates Application Recommended Base Metals °F °C 1080–1140 560–615 All brazeable aluminum alloys. Because of very limited use of brazing to join magnesium, a detailed classification of brazing fluxes for magnesium is not included. 900–1150 480–620 Magnesium alloys whose designators start with AZ. General purpose flux for most ferrous and nonferrous alloys. (Notable exception Al bronze, etc. See Flux 4A. 1050–1600 565–870 All brazeable ferrous and nonferrous metal except those with aluminum or magnesium as a constituent. Also used to braze carbides General purpose flux for many alloys containing metals that form refractory oxides 1100–1600 595–870 Brazeable base metals containing up to 9% aluminum (aluminum brass, aluminum bronze, Monel K500). May also have application when minor amounts of Ti, or other metals are present, which form refractory oxides. Note: The selection of a flux designation for a specific type of work may be based on the filler metal type and the description above, but the information here is generally not adequate for flux selection. The above chart represents a partial listing of Table 4.1 Brazing Fluxes from the AWS Brazing Handbook. Source: AWS Brazing Handbook © 1991. areas within the joint. These can result from insufficient cleaning or improper heating of the parts. Another problem occurs when too much localized heat is applied to the base metal, resulting in melting and erosion of the base metal. This is normally associated with torch brazing where the combination of the flames’ heat and its mechanical action will wear away the base metal adjacent to the braze joint. Another concern is the corrosion of the base metal by some of the extremely reactive fluxes; flux residue must be removed to avoid subsequent corrosion of the joint or base metal. One of the biggest advantages of brazing is that it can be used to join dissimilar metals. This is possible since brazing does not melt the base metals to produce a hybrid alloy which may not have desirable properties. It is also well-suited for the joining of metals which simply don’t lend themselves to welding of any type. Another advantage of brazing is the equipment can be relatively inexpensive. Since brazing uses lower temperatures than welding, thin metals are readily joined without as much fear of melt-through or distortion. The primary limitation is that the parts must be extremely clean prior to brazing. Another limitation is that the joint design must provide sufficient surface area to develop the required strength. Some configurations do not provide such a situation. Cutting Processes So far the discussion has involved only those methods used for joining metals together. Also of importance in metal fabrication are those processes used to cut or There are several inherent problems associated with brazing. The first is the formation of voids or unbonded 3-38 WELDING INSPECTION TECHNOLOGY CHAPTER 3—METAL JOINING AND CUTTING PROCESSES remove metal. These processes are often required prior to welding to produce proper part shapes or make specific joint preparations. During or after welding, some of these same processes can also be employed to remove defective areas of welds or to produce a specific configuration if the as-welded shape is not satisfactory for the intended purpose of the part. Oxyfuel Gas Cutting (OFC) The first of these cutting processes is oxyfuel gas cutting. Here, we use an oxyfuel flame to heat the metal to a temperature at which it will readily oxidize, or burn. The temperature needed is referred to as the kindling temperature, and for steels, it is about 1700°F [925°C]. Once that temperature has been achieved, a high pressure stream of cutting oxygen is directed on the heated surface to produce an oxidation reaction. This stream of oxygen also tends to remove the slag and oxide residue which is produced by this oxidation reaction. Therefore, OFC can be thought of as a type of chemical cutting process. Figure 3.56 shows the basic placement of the cutting torch necessary to achieve a cut in carbon steel. The equipment used for OFC is essentially the same as that for OAW except that, instead of a welding tip, there is now a cutting attachment which includes an additional lever or valve to turn on the cutting oxygen. Figure 3.57 shows a typical OFC equipment setup found in most welding and fabrication shops. Figure 3.57—Oxyfuel Cutting Equipment The cutting operation also requires a special cutting tip which is attached to the end of the torch. It consists of a series of small holes arranged in a circle around the outside edge of the end of the cutting tip. This is where the oxyfuel gas mixture flows to provide the preheat for cutting. Located in the center of these holes is a single cut- ting-oxygen passage. Cross-sectional views of typical cutting tips are shown in Figure 3.58, and torches used for manual and machine cutting are shown in Figure 3.59. It should be noted that OFC can be accomplished using several different types of fuel gases, such as acetylene, methane (natural gas), propane, gasoline, and methylacetylene-propadiene (MPS). Each provides various degrees of efficiency and may require slightly modified cutting tips. Other factors which should be considered when selecting the proper fuel gas include preheating time required, cutting speeds, cost, availability, amount of oxygen required to burn gas efficiently, and ease and safety of transporting fuel containers. CUTTING OXYGEN PREHEATING OXYGEN SHORT GAP ACETYLENE TR AV EL PREHEATING FLAMES Cutting is accomplished by applying heat to the part using the preheat flame which is an oxyfuel mixture. Once the metal has been heated to its oxidation temperature, the cutting oxygen is turned on to oxidize the hot metal. The oxidation of the metal produces a tremendous amount of heat. This exothermic chemical reaction provides the necessary heat to rapidly melt the metal and simultaneously blow the oxidation products from the PREHEAT TO CHERRY RED BEFORE STARTING TO CUT Figure 3.56—Oxyfuel Cutting 3-39 CHAPTER 3—METAL JOINING AND CUTTING PROCESSES WELDING INSPECTION TECHNOLOGY joint. The width of the cut produced is referred to as the kerf, as shown in Figure 3.60. Also shown is the drag, which is the amount of offset between the cut entry and exit points, measured along the cut edge. Although OFC is used extensively by most industries, it is usually limited to the cutting of carbon and low alloy steels. As the amounts of various alloying elements increase, one of two things can happen; either they make the steel more difficult to cut or they may give rise to hardened or heat-checked cut surfaces, or both. The effects of various alloying elements are summarized in Table 3.6. (A) One Piece Tip (B) Two Piece Tip As can be seen, in most cases, the addition of certain amounts of alloying elements may prevent conventional OFC. In many cases, these elements are oxidation resistant types. In order for an oxyfuel cut to be effectively accomplished, the material must comply with the following criteria: (1) it must have the capability of burning in a stream of oxygen, (2) its ignition temperature for burning must be lower than its melting temperature, (3) its heat conductivity should be relatively low, (4) the metal oxide produced must melt at some temperature below the melting point of the metal, and (5) the formed slag must be of low viscosity. Therefore, in order to cut cast iron or stainless steel with this process, special techniques involving additional equipment are necessary. These techniques include torch oscillation, use of a waster plate, wire feeding, powder cutting, and flux cutting. Figure 3.58—Cross Section Through Cutting Tips OFC’s advantages include its relatively inexpensive and portable equipment, making it feasible for use in both shop and field applications. Cuts can be made on thin or thick sections; ease of cutting usually increases with thickness. When mechanized (Figure 3.61), OFC can produce cuts of reasonable accuracy. When compared to mechanical cutting methods, oxyfuel cutting of steels is more economical. To improve this efficiency even more, multiple torch systems or stack cutting can be used to cut several layers at once. Figure 3.59—OFC Torches for Machine and Manual Oxyfuel Cutting One of the limitations of OFC is that the finished cut may require additional cleaning or grinding to prepare it for welding. Another important limitation is that since it requires high temperatures, there may be a heat-affected zone produced having a very high hardness. This is especially important if there is a need for machining of this surface. Employment of preheat and postheat will aid in the alleviation of this problem. Also, even though cuts can be reasonably accurate, they still don’t compare to the accuracy possible from mechanical cutting methods. Finally, the flame and hot slag produced result in safety hazards for personnel near the cutting operation. DRAG KERF Figure 3.60—Illustration of Kerf and Drag in Oxyfuel Cutting 3-40 WELDING INSPECTION TECHNOLOGY CHAPTER 3—METAL JOINING AND CUTTING PROCESSES Table 3.6 Effects of Chemical Elements on Oxyfuel Cutting Element Effect of Element on Oxygen Cutting Carbon Steels up to 0.25% carbon can be cut without difficulty. Higher carbon steels should be preheated to prevent hardening and cracking. Graphite and cementite (Fe2C) are detrimental but cast irons containing 4% carbon can be cut by special techniques. Manganese Steels of about 14% manganese and 1.5% carbon are difficult to cut and should be preheated for best results. Silicon Silicon, in amounts usually present, has no effect. Transformer irons containing as much as 4% silicon are being cut. Silicon steel containing large amounts of carbon and manganese must be carefully preheated and post-annealed to avoid air hardening and possible surface fissures. Chromium Steels up to 5% chromium are cut without much difficulty when the surface is clean. Higher chromium steels, such as 10% chromium steels, require special techniques and the cuts are rough when the usual oxyacetylene cutting process is used. In general, carburizing preheat flames are desirable when cutting this type of steel. The flux injection and iron powder cutting processes enable cuts to be readily made in the usual straight chromium irons and steels as well as stainless steel. Nickel Steels containing up to 3% nickel may be cut by the normal oxygen cutting processes; up to about 7% nickel content, cuts are very satisfactory. Cuts of excellent quality may be made in the usual engineering alloys of the stainless steels (18–8 to about 35–15 as the upper limit) by the flux injection or iron powder cutting processes. Molybdenum This element affects cutting about the same as chromium. Aircraft quality chrome-molybdenum steel offers no difficulties. High molybdenum-tungsten steels, however, may be cut only by special techniques. Tungsten The usual alloys with up to 14% may be cut very readily, but cutting is difficult with the higher percentage of tungsten. The limit seems to be about 20% tungsten. Copper In amounts up to about 2%, copper has no effect. Aluminum Unless present in large amounts (about 10%) the effect of aluminum is not appreciable Phosphorus This element has no effect in amounts usually tolerated in steel. Sulfur Small amounts, such as are present in steels, have no effect. With high percentages of sulfur, the rate of cutting is reduced and sulfur dioxide fumes are noticeable. Vanadium In the amounts usually found in steels, this alloy may improve rather than interfere with cutting. Air Carbon Arc Cutting (CAC-A) the stream of compressed air is initiated and blows away the molten metal to produce a gouge or cut. Another very effective cutting process is air carbon arc cutting. This process uses a carbon electrode to create an arc for heating, along with a high pressure stream of compressed air to mechanically remove the molten metal. Figure 3.62 shows the process in use. The electrode holder is attached to a power source as well as a source of compressed air. Any nonflammable compressed gas could be used, but compressed air is by far the least expensive, if available. The entire system for air carbon arc cutting is shown in Figure 3.64. The equipment used for CAC-A consists of a special electrode holder which is attached to a constant current power source and a compressed air supply. This special holder, shown in Figure 3.63, grasps the carbon electrode in copper jaws, one of which has a series of holes through which the compressed air passes. CAC-A has applications in most industries. Even though it will cut most metals, there are other considerations that may require other cutting methods for particular alloys. Table 3.7 shows the current type and polarity for CAC-A cutting of several metals and alloys. While we tend to think of its application to remove defective areas of the weld or base metal, it is important To achieve a cut, the carbon electrode is brought close to the work to create an arc. Once the arc melts the metal, 3-41 CHAPTER 3—METAL JOINING AND CUTTING PROCESSES WELDING INSPECTION TECHNOLOGY Figure 3.63—Air Carbon Arc Cutting Electrode Holder Figure 3.61—Machine OFC Cutting Figure 3.64—Air Carbon Arc Cutting Equipment Figure 3.62—Air Carbon Arc Cutting to realize that it can be used quite effectively as a weld joint preparation tool. For example, two pieces to be butt welded can be aligned with their square-cut edges touching. The CAC-A process can then be employed to produce a uniform U-groove preparation, as shown in Figure 3.65. CAC-A is also used for rough machining of large, complex parts, as well as backgouging of a doublesided complete joint penetration weld. One of the basic advantages of CAC-A is that it is a relatively efficient method for removal of metal. Since it uses the same power sources as those used for some 3-42 WELDING INSPECTION TECHNOLOGY CHAPTER 3—METAL JOINING AND CUTTING PROCESSES types of welding, the equipment costs are minimal. All that is necessary is the purchase of the special electrode holder which is attached to an existing power source and a compressed air supply. Table 3.7 CAC-A Electrical Requirements for Various Metals Metal Aluminum Copper and alloys Iron, cast, malleable, etc. Magnesium Nickel and alloys Carbon steels Stainless steels Current Type Electrode Polarity DC AC DC DC AC DC DC Positive NA Negative Positive NA Positive Positive The primary disadvantage of the process is safetyrelated. It is inherently a very noisy and dirty process. The use of ear protection to reduce the noise level, and respirators to eliminate the inhaling of the metal particles produced is required. A fire watch may also be required to make sure the gouged metal droplets do not create a fire hazard. Another limitation is that the finished cut may require some cleanup prior to additional welding. Carburization of the cut may occur. Figure 3.65—Illustration of Joint Preparation Using Mechanized (left) and Manual (right) Air Carbon Arc Cutting 3-43 CHAPTER 3—METAL JOINING AND CUTTING PROCESSES WELDING INSPECTION TECHNOLOGY Plasma Arc Cutting (PAC) For mechanized PAC cutting, not only is the torch watercooled internally, but the actual cutting may take place under water or oil to reduce noise and particulate levels. The final thermal cutting method for discussion is plasma arc cutting. This process is similar in most respects to PAW except that now the purpose is to remove metal rather than join pieces together. The equipment requirements are similar except that the power required may be much higher than that used for welding. The transferred arc type torch is used because of the increased heating of the base metal. Typical PAC torches for manual and machine cutting are shown in Figure 3.66, and a PAC equipment setup is shown in Figure 3.67. While its primary application is for the cutting of nonferrous metals, PAC is also useful for the cutting of carbon steels. Advantages include the ability to cut metals which cannot be cut with OFC, the resulting high quality cut, and increased cutting speeds for carbon steel. One limitation is that the kerf is generally quite large and the cut edges may not be square. Special techniques, such as water injection, can be used to improve this edge configuration if desired. Another limitation is the higher cost of equipment as compared to oxyfuel cutting. Mechanical Cutting Finally, brief mention of mechanical cutting methods used in conjunction with welding will be presented. These methods can include shearing, sawing, grinding, milling, turning, shaping, drilling, planing, and chipping. They are used for joint preparation, weld contouring, parts preparation, surface cleaning, and removal of defective welds (see Figure 3.68). Figure 3.66—Typical Manual and Machine Plasma Arc Cutting Torches A welding inspector should understand how these methods are used. Their misapplication may have a degrading effect on final weld quality. For example, many of these methods use cutting fluid to aid their operation. If the fluids are not completely removed from material surfaces prior to welding, problems such as porosity and cracking may result. Figure 3.67—Plasma Arc Cutting Equipment Figure 3.68—Mechanical Grinder 3-44