15 Essential Concepts for AP II_2402 (4) PDF
Document Details
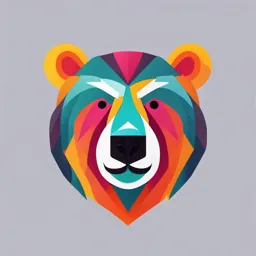
Uploaded by WondrousMahoganyObsidian
Tags
Summary
This document reviews essential concepts of homeostasis, including homeostasis, extracellular fluid, and its components. It also covers feedback loops, negative and positive, with examples like childbirth and high blood pressure. Finally, the review tackles diffusion across a membrane and osmosis.
Full Transcript
Essential Concepts Review Homeostasis Homeostasis – the maintenance of a relatively stable internal environment Homeostasis within the body is essential for survival and function of all cells Most cells are not in direct contact with the external environment Each cell contributes to homeostasis,...
Essential Concepts Review Homeostasis Homeostasis – the maintenance of a relatively stable internal environment Homeostasis within the body is essential for survival and function of all cells Most cells are not in direct contact with the external environment Each cell contributes to homeostasis, although some more than others Homeostasis Extracellular fluid (ECF) – the fluid environment in which the cells live (fluid outside the cells) s Plas ma Two components: of ECF: Plasma – the liquid portion of blood Blood Interstitial fluid Interstitial fluid (ISF)– the fluid between cells Cells Intracellular fluid (ICF) – the fluid contained within all body cells contain intracellular fluid Extracellular fluid Body cells are contained in a watery internal environment through which life-sustaining exchanges are made RBC Balancing the Internal and External Environment Cells, the fundamental units of life, exchange nutrients and wastes with their surroundings: The intracellular fluid (ICF) is conditioned by the interstitial fluid (ISF), which is conditioned by the plasma, which is conditioned by the organ that it passes through Extracellular fluid External environment Organs Plasma Interstitial Fluid (ISF) Internal Environment Intracellular Fluid (ICF) Homeostasis Homeostasis involves dynamic mechanisms that detect and respond to deviations in physiological variables from their “set point” values by initiating effector responses that restore those variables to the optimal physiological range. The two primary systems that maintain homeostasis 1. Nervous system 2. Endocrine system Maintenance of Homeostasis Nervous system Neurons control and coordinate bodily activities that require rapid responses Detects and initiates reactions to changes in external environment Endocrine system Secreting endocrine glands regulate activities that require duration rather than speed Controls concentration of nutrients and, by adjusting kidney function, controls internal environment’s volume and electrolyte concentration Homeostasis Factors homeostatically regulated include: Concentration of nutrient molecules Concentration of water, salt, and other electrolytes Concentration of waste products Partial pressures of gases: O2 = 100 mmHg CO2 = 40 mmHg pH = 7.35 – 7.45 Blood volume = 4 – 6 Liters Blood pressure ≈ 120/80 mmHg Temperature = 37° C Control of Homeostasis Homeostasis is continually being disrupted by: External stimuli Heat, cold, lack of oxygen, pathogens, toxins Internal stimuli Body temperature Blood pressure Concentration of water, glucose, salts, oxygen, etc. Physical and psychological distresses Disruptions can be mild to severe. Pathophysiological states develop when body systems fail to maintain an optimal internal environment Serious homeostatic disruption leads to death Homeostatic Control Systems Networks of body components working together to maintain a given factor in the internal environment directed at an optimal set level In order to maintain homeostasis, control system must be able to: Detect deviations from normal in the internal environment that need to be held within narrow limits Integrate this information with other relevant information Make appropriate adjustments in order to restore factor to its desired value Homeostatic Control Systems Control systems are grouped into two classes: Intrinsic controls Local controls that are inherent within an organ Involves detecting deviations and making corrections within the organ, often called autoregulation Tends to primarily benefit the organ Extrinsic controls Regulatory mechanisms initiated outside an organ Accomplished by nervous and endocrine systems Responses of an organ that are triggered by factors external to the organ or systems. Can over-ride intrinsic regulation Tends to benefit the organism, not necessarily individual organs Homeostatic Control Systems Feedforward – a term used for responses made in anticipation of a change Example: the smell of food initiates gastric secretions and motility in anticipation of a meal Feedback - refers to responses made after change has been detected Two Types of Feedback: Negative Feedback Loop (NFbL) Changes that move a value away from the reference value are attenuated (reduced) Original stimulus is alleviated Most feedback systems in the body are negative Used for conditions that need frequent adjustment Positive Feedback Loop (PFbL) Changes that move a value away from the reference value are enhanced Original stimulus is intensified Far less common; Seen during normal childbirth and lactation Components of a Negative Feedback System Internal or external change Negative feedback loops consist of: Stimulus – a change Receptor Receptor – structures that monitor a controlled condition and detect the change Control Center (Integration Center) – determines next action Efferent Pathway – the means to send a signal from the integration center to the effector organ Effector – the organ or tissue that receives directions from the control center and produces Negative Feedback Afferent Pathway – the means to communicate information about the change from the receptor to the control center; can be neurons or blood Afferent path: Neuron Integrating Center: CNS or Endocrine Efferent path: Neuron or blood Effectors: Muscles or glands Response – the output that removes the original stimulus Response * Dotted lines typically represent an inhibitory pathway Components of a Positive Feedback System Internal or external change Positive feedback loops consist of: Stimulus – a change Receptor Receptor – structures that monitor a controlled condition and detect the change Control Center (Integration Center) - determines next action Efferent Pathway – the means to send a signal from the integration center to the effector organ Effector – the organ or tissue that receives directions from the control center and produces Response – the output that increases the original stimulus Positive Feedback Afferent Pathway – the means to communicate information about the change from the receptor to the control center; can be neurons or blood Afferent path: Neuron Integrating Center: CNS or Endocrine Efferent path: Neuron or blood Effectors: Muscles or glands Response Thyroid Hormone NFbL Stimulus: Low serum T3, T4 Receptors: T3, T4 binding sites on Hypothalamus Neural Integrating Center: Hypothalamus releases TRH Negative Feedback Efferent Pathway: Hypothalamic-Pituitary Portal Veins Endocrine Integrating Center: Anterior Pituitary releases TSH Efferent Pathway: Bloodstream Endocrine Integrating Center: Thyroid Gland (releasing T3, T4) Efferent Pathway: Bloodstream Effector: Various body tissues Response: Increased serum sT3, T4, relieves original stimulus High Blood Pressure NFbL Stimulus: ↑ BP (hypertension) Receptors: Baroreceptors in walls of blood vessels detect ↑BP Integrating Center: CNS (medulla oblongata) Efferent Pathway: Vagus nerve (motor) Effector: Cardiac muscle of the heart Response: ↓HR Feedback: ↓ BP, relieving initial stimulus Negative Feedback Afferent Pathway: Vagus nerve (sensory) Childbirth PFbL Stimulus: Amniotic sac breaks, fetus drops lower in uterus Receptors: Stretch receptors in walls of uterus Afferent Pathway: Vagus nerve (sensory) to Hypothalamus Efferent Pathway: Hypothalamo-neurohypophyseal nerve tract Integrating Center: Neurohypophysis releases oxytocin Efferent Pathway: Bloodstream to uterine smooth muscle Effector: Uterine contractions cause cervix to dilate & fetus to descend in birth canal Response: More stretch… more oxytocin release… more contractions…. repeat PFbL ends with birth of the baby & ↓ uterine stretch Positive Feedback Integrating Center: Hypothalamus Diffusion Diffusion – the net movement of a substance from an area of high concentration to an area of low concentration; down its concentration gradient Caused by the constant random motion of all atoms and molecules Lump of sugar Random movement leads to net movement down a concentration gradient Water No net movement at equilibrium Diffusion Across A Membrane The membrane has pores large enough for the molecules to pass through. Random movement of the molecules will cause some to pass through the pores; this will happen more often on the side with more molecules. The molecule diffuses from where it is more concentrated to where it is less concentrated This leads to a dynamic equilibrium – the solute molecules continue to cross the membrane, but at equal rates in both directions. Net diffusion Net diffusion Equilibrium Diffusion Across A Membrane Two different solutes are separated by a membrane that is permeable to both Each solute diffuses down its own concentration gradient. There will be a net diffusion of the purple molecules toward the left, even though the total solute concentration was initially greater on the left side Net diffusion Net diffusion Equilibrium Net diffusion Net diffusion Equilibrium Permeability of the Lipid Bilayer 4 Permeability Factors Lipid solubility Hydrophobic (non-polar) molecules are lipid soluble and can pass through the phospholipid membrane easily Size Small molecules pass through much more easily than large molecules Charge Charged molecules do not cross the membrane as easily as neutral molecules Presence of carriers and channels Transport proteins allow passage of hydrophilic, large, or charged substances across the membrane Passive Transport Processes Three types of passive transport: Dialysis - selective diffusion of solutes Lipid-soluble materials Small molecules that can pass through membrane pores unassisted Osmosis – diffusion of water Facilitated diffusion – substances require a protein carrier or channel for passive transport High concentration Low concentration Facilitated diffusion through Protein Carriers Facilitated diffusion through Protein Channels Diffusion through the lipid bilayer Certain molecules bind to a protein, triggering a change in protein shape that transports the molecule across the membrane. Glucose typically enters cells by this method. Some polar and charged molecules diffuse through protein channels that span the membrane. Water is a typical example. Lipid-soluble molecules such as O2 and CO2 diffuse freely through the plasma membrane Osmosis Osmosis – the diffusion of water across a semipermeable membrane from an area of low solute concentration to an area of high solute concentration In some circumstances, the solvent may be something other than water. However, in living systems the solvent is always water, so biologists generally define osmosis as the diffusion of water across a semipermeable membrane. Semipermeable membrane: - permits the passage of water, not solutes. Remember: Water always flows toward the higher concentration of solutes. RBC 0.9% 5% NACL RBC 0.9% RBC 0.5% 5% NACL 0.01% NACL Tonicity Tonicity – describes a solution and how that solution would effect cell volume Hypertonic solutions have a relatively higher [solute]. A cell placed in the solution will lose water and shrink (crenate). Hypotonic solutions have a relatively lower [solute]. A cell placed in the solution will gain water, swell, and burst (lyse). Isotonic solutions have equal [solute]. A cell placed in the solution will not change in size. Hypotonic a Equ er Few H2 e Mor Lysed (Hemolysi Hypertonic Isotonic l e Mor H2 H2 a Equ l Normal (No Water always rushes toward the higher concentration of solutes H2 er Few Shriveled (Crenatio = Facilitated Diffusion Facilitated Diffusion uses membrane proteins to transport large, polar molecules that are not usually permeable to the phospholipid bilayer. Two types of transport proteins: 1) Channel proteins – provide a narrow channel for the substance to pass through. Mostly for water and ions 2) Carrier proteins – physically bind to the substance on one side of membrane, and change conformation in order to release it on the other Ideal for small organic molecules, such as glucose and amino acids, that are too large to pass through channels. Active Transport Active transport uses energy from ATP to move a substance against its concentration gradient Requires the use of carrier proteins, not channel proteins 2 types of Active Transport: Membrane pump (protein-mediated active transport) Coupled transport (co-transport) 3 NA+ The Na+/K+ Pump 2k+ Most important membrane pump in all animal cells Transport 3 Na+ out of the cell and 2 K+ into the cell for each ATP consumed Properly called “Na+/K+ ATPase” [Na+] high [K+] low Na+ 1. Cytoplasmic Na+ binds to the sodiumpotassium pump. K+ + NaEXTRACELLULAR FLUID [Na+] low Na+ [K+] high CYTOPLASM 6. is released and sites are receptive again; the cycle repeats. Na+ 2. Na+ binding stimulates phosphorylation by ATP. Na+ Na+ 3. Phosphorylation causes the protein to change its conformation, expelling Na+ to the outside. Na+ K+ P K+ 5. Loss of the phosphate restores the protein’s original conformation. Na+ Na+ Na+ ATP P ADP K+ 4. Extracellular K+ binds to the protein, triggering release of the Phosphate group. K+ K+ K+ Pi P Pi Coupled Transport 2 proteins involved: The initial carrier protein uses ATP to move substance 1 across the membrane against its concentration gradient, storing potential energy. Coupled transport protein (“cotransporter”) allows substance 1 to move back down its concentration gradient, using its stored energy to move substance 2 up its concentration gradient Na+ is pumped against its concentration gradient using ATP High [Na+] ATP Na+ Na+ Pump Low [Na+] Na+ Na+/Ca+ Cotransporter High [Ca+] Ca++ Low [Ca+] Na+ is then allowed to diffuse down its concentration gradient, pulling Ca2+ along with it Types of Cell to Cell Communication Gap junctions Receptor site Direct contact local, cell to cell communication via gap junctions or direct contact Target cell Paracrine signaling “para-” = near; “-crine” = secrete local communication between nearby cells via secretion of a chemical signal (cytokines) Neuron Synaptic signaling local secretion of neurotransmitters by a neuron into a synapse with another neuron or other target cell Endocrine signaling “endo-” = within; “-crine” = secrete also called hormone signaling long distance communication via secretion of hormones into the bloodstream Cytokines Secreting cells Neurotransmitter In synapse Target cell Endocrine cell Hormone Blood vessel Target cell Chemical Signaling Cells communicate chemically, via signal molecules known as ligands Ligands can be any chemical that binds to a specific protein receptor Examples: Hormones Ions Neurotransmitters Drugs Toxins Gases Chemical Signaling Ligands fall under two categories: Lipophilic and Lipophobic Lipophilic Ligand Lipophilic ligand Lipophilic ligands – can diffuse through the phospholipid bilayer of the cell membrane and bind to cytosolic or nuclear receptors to generate a response within the cell Cytosolic receptors can modify gene expression within the nucleus, or alter existing cytosolic proteins Nuclear receptors can turn genes on or off, regulating protein synthesis Cytosolic receptor Intracellular response Nuclear receptor Intranuclear response Note: Some lipophilic substances bind to membrane receptors Chemical Signaling Lipophobic ligands – are unable to diffuse through the phospholipid bilayer and, instead, bind to receptor proteins on the membrane, thereby transducing the signal and generating an intracellular response without entering the cell Ligand-gated (chemically-gated) ion channels Enzymatic receptors G protein-linked receptors Lipophobic Ligands Intracellular Response Enzy m Rece atic ptor Protein Channel Lipophobic ligands G-protein linked receptor Intracellular Response G-protein zy En me Intracellular Response Note: Some lipophilic substances bind to membrane receptors Chemically-Gated Ion Channels Chemically-Gated Ion Channels open or close when the ligand binds to the channel protein Found on the cell membrane, allowing ions in or out of the cell Also found on plasma membranes of organelles inside of the cell Most are neurotransmitter receptors on nerve and muscle cells Signal molecule (ligand) Gate Gate close Closed Ligand-gated ion channel receptor Ions Plasma Membrane Gate open Example: Acetylcholine, a neurotransmitter, is released from a neuron and binds to the acetylcholine receptors on target cell, which opens a channel, allowing Na+ to enter the cell, flowing down its electrochemical gradient. Net entry of Na+’s positive charge depolarizes the cell. Cellular response Gate close Enzymatic Receptors Enzyme-linked receptors are embedded in the plasma membrane, with their catalytic site exposed inside the cell A ligand binds to the receptor site, activating the catalytic site within the cell Most enzyme-linked receptors function as protein kinases, enzymes that phosphorylate proteins Phosphorylation is the process of activating a protein by the addition of a phosphate (PO43) group The phosphate group is donated by energy molecules such as ATP Adenosine Triphosphate = Adenosine Diphosphate + Phosphate group Signal molecule Receptor site Enzymelinked Receptor Catalytic site ATP (Phosphorylation) Inactiv e protein ADP Active phosphorylated protein Insuline Enzymatic Receptors - RTK 150mg/dl rtk normalBGL=70-110mg/dl h20 leaves when rTK Pancreas 90mg/dl The most common enzyme-linked receptor is the receptor tyrosine kinase (RTK) For example, Receptor Tyrosine-Kinases are used by: Insulin to initiate the insertion of GLUT4 proteins into the plasma membrane for the facilitated diffusion of glucose into the cell Growth factors to spur mitosis and cell division. Because of its role in cell division, excessive tyrosine kinase signaling is associated with many types of cancer Signal molecules Active proteins Tyr Tyr Tyr Tyr Tyr Tyr Receptor tyrosine kinase (inactive monomers) Tyr Tyr Tyr Tyr Tyr Tyr Dimer Tyr Tyr Tyr Tyr Tyr Tyr 6ATP Activated tyrosinekinase (unphosphorylated dimer) 6 ADP P Tyr P Tyr P Tyr Tyr P Tyr P Tyr P Activated tyrosinekinase (phosphorylated dimer) P Tyr P Tyr P Tyr Tyr P Tyr P Tyr P Inactive proteins Cellular response Cellular response Signal Transduction Three stages of Signal Transduction: 1) Reception – an extracellular ligand binds to and activates a membrane receptor 2) Transduction – the activated membrane receptor turns on its associated proteins and starts an intracellular cascade of second messengers 3) Response – the last second messenger in the cascade acts on intracellular targets to create a response Second Messengers Some enzymatic receptors and most G-protein-linked receptors relay their message into the cell by activating other molecules or ions inside the cell Extracellular signals are converted into intracellular messages, which create a response Second messengers – molecules & ions that transmit the message within the cell Most common second messengers: cAMP Ca2+ cAMP Second Messenger G protein signaling pathway First messenger (signal molecule such as epinephrine) 1. Signal molecule binds to surface receptor 2. Surface receptor activates a G protein 3. G protein activates the membrane-bound enzyme, adenylyl cyclase 4. Adenylyl cyclase catalyzes synthesis of cAMP, which binds to a protein kinase 5. Target protein initiates cellular change G protein G-protein-linked receptor Adenylyl cyclase GTP ATP Second cAMP messenger Protein kinase A Cellular responses Cyclic AMP cAMP recycling takes place in the mitochondria as a part of ATP synthesis NH2 N N O –O O O N N P O P O P O Ch2 O− O− O− O O P Pi CH2 Phoshodiesterase O P O− O OH Cyclic AMP N N N N Adenylyl cyclase O OH OH N N Pyrophosphate ATP NH2 NH2 N N O HO P O CH2 O− O H 2O OH OH AMP Cyclic AMP Pathway Amplification Signal Amplification Stimulation of Glycogen Breakdown in a Liver Cell by Epinephrine Reception Epinephrine binds to G-protein receptor which… Binding of epinephrine to G-protein-linked receptor (1 molecule) Transduction …activates 100 G-proteins which… …activates 100 adenylyl cyclase enzymes which… Inactive G protein Active G protein (102 molecules) Inactive adenylyl cyclase Active adenylyl cyclase (102) …activates 1000 cyclic AMP molecules which… …activates 1000 protein kinase enzymes which… …activates 100,000 phosphorylase kinase enzymes which… …activates 1,000,000 glycogen phosphorylase enzymes which… …convert chains of glycogen into 100,000,000 glucose molecules… ATP Cyclic AMP (104) Inactive protein kinase A Active protein kinase A (104) Inactive phosphorylase kinase Active phosphorylase kinase (105) Inactive glycogen phosphorylase Active glycogen phosphorylase (106) Response Glycogen Glucose-1-phosphate (108 molecules) Calcium and IP3 in Signaling Pathways 1 A signal molecule binds to a receptor, leading to activation of phospholipase C. 1) Signal molecule binds to surface receptor EXTRACELLULAR FLUID 2 Phospholipase C cleaves a plasma membrane phospholipid called PIP2 into DAG and IP3. 3 DAG functions as a second messenger in other pathways. Signal molecule (first messenger) G protein 2) Surface receptor activates a G protein DAG GTP 3) G protein activates the membrane-bound enzyme, phospholipase C 4) Phospholipase C catalyzes synthesis of inositol triphosphate (IP3), which stimulates release of Ca2+ from ER 5) Released Ca2+ initiates cellular change G-protein-linked receptor Phospholipase C PIP2 IP3 (second messenger) IP3-gated calcium channel Endoplasmic reticulum (ER) CYTOSOL 4 Various proteins activated Ca2+ IP3 quickly diffuses through the cytosol and binds to an IP3– gated calcium channel in the ER membrane, causing it to open. Cellular responses Ca2+ (third messenger) 5 Calcium ions flow out of the ER (down their concentration gradient), raising the Ca2+ level in the cytosol. 6 The calcium ions activate the next protein in one or more signaling pathways. Neurophysiology Basic Concepts Review Ions – charged particles Anions – Negatively charged particles Cations – Positively charged particles Electrostatic forces Opposite charges attract, same charges repel Ions flow along their electrical gradient when they move toward an area of opposite charge Concentration forces Diffusion – movement of ions through semipermeable membrane Ions flow along their chemical gradient when they move from an area of high concentration to an area of low concentration Together, the electrical and chemical gradients constitute the electrochemical gradient Sodium-Potassium Pump Is an active transport system present in all cells Pumps 3 Na+ out of cell for every 2 K+ pumped into the cell, against their [C] gradients It is an electrogenic pump - generates the voltage across a membrane Establishes ion [C] gradients necessary for nerves to conduct electrical signals Membrane Potential Membrane potential is the voltage difference across a membrane Resting membrane potential is a -70mV difference between the inside and the outside when the cell is not depolarizing– the membrane is polarized When voltage-gated ion channels open, ions diffuse across the membrane following their electrochemical gradients This movement of charge is an electrical current and can create voltage (measure of potential energy) change across the membrane. Voltmeter Plasma membrane -70 mV Microelectrode outside cell Microelectrode inside cell Axon -55 mv = threshold Neuron Resting Membrane Potential The resting potential exists because ions are concentrated on different sides of the membrane by Na+/K+ Pump: Na+ and Cl- outside the cell K+ and impermeable anionic proteins inside the cell Leak channels allow some ions to sneak back down their concentration gradient Gated Ion Channels Gated channels control ion permeability Mechanically gated – respond to pressure or vibration Chemically gated – respond to chemical ligands, ie neurotransmitters, hormones, and ions Voltage gated – respond to direct change in membrane potential Threshold voltage varies from one channel type to another Graded Potentials vs action Short-lived change in membrane potential in dendrites or cell body Currents decrease in magnitude with distance Their magnitude varies directly with the strength of the stimulus – the stronger the stimulus the more the voltage changes and the farther the current goes Sufficiently strong graded potentials can initiate action potentials if they maintain threshold by the time they reach the trigger zone Trigger Zone Initiates Action Potentials A graded potential above threshold (above -55 mV) reaches the trigger zone and becomes an action potential Graded Potential fades before reaching threshold Graded Potential becomes Action Potential Action Potentials INTENSITY BASED UPON FREQ of AC, how many times The action potential is a brief reversal of membrane potential down a neuron’s axon When threshold voltage (-55mV) is reached at the trigger zone, voltage gated Na+ channels open Na+ enters the cell, down its electrochemical gradient, producing depolarizing currents down the axon Action Potentials do not decrease in strength with distance All-or-None phenomenon – action potentials either happen completely, or not at all Action Potentials Action potentials consist of three main phases: Depolarization Repolarization Hyperpolarization Ion Permeability during an AP Absolute Refractory Period Na+ channels close Dep ola riza tion Na + inf lux -55 Threshold -70 Resting Na+ channels open K 0 K+ channels open ion izat olar Rep + efflux Voltage (mV) +30 Relative Refractory Period Na+/K+ pump restores resting potential Hyperpolarization Slow-closing K+ channels close Sodium always goes into SODIUM CHANNEL, POTASSIUM ALWAYS WANTS TO GO OUT depolarization=sodium repolarize=potassium Time (msec) HYPERKALEMIA= Potassium in latin is kalium Sodium = natrium in latin NA,CA,CL = always higher outside NA and CA stimulate CL =inhibit Sodium-Potassium Pump Restores Ionic Gradients Repolarization restores the resting electrical conditions of the neuron, but does not restore the resting ionic conditions Ionic redistribution is accomplished by the Na+/K+ pump following repolarization 3 Na+ released into ECF Net positive charge 3 Na+ from ICF ATPase is phosphorylated with Pi from ATP 2 K+ from ECF Net negative charge 2 K+ released into ICF Potassium and Cell Excitability POTASSIUM AND CELL EXCITABILITY Membrane potential (mV) Normal plasma [K+] is 3.5 – 5 mM. 0 −55 Hyperkalemia depolarizes cells. 0 Threshold −70 Threshold Stimulus Time When blood K+ is in the normal range (normokalemia), a subthreshold graded potential does not fire an action potential. In normokalemia, a suprathreshold (abovethreshold) stimulus will fire an action potential. Threshold −55 −70 −70 Stimulus 0 0 −55 Hypokalemia hyperpolarizes cells. Stimulus −55 Threshold −70 Stimulus Hyperkalemia, increased blood K+ concentration, brings the membrane closer to the threshold. Now a stimulus that would normally be subthreshold can trigger an action potential. HYPERKALEMIA=HYPEREXCITABILITY Death and potassium 3 Hypokalemia, decreased blood K+ concentration, hyperpolarizes the membrane and makes the neuron less likely to fire an action potential in response to a stimulus that would normally be above the threshold. Neurotransmitter Release @ +30mv CA2+ channels open Neurotransmitter Removal NTs are removed from the synaptic cleft via: Reuptake (endocytosis) Enzymatic degradation (ACH erase) Diffusion EPSPs & IPSPs Typically, a single synaptic interaction will not create a graded depolarization strong enough to induce the firing of an AP Graded depolarizations will bring the membrane potential closer to threshold. This is referred to as an excitatory postsynaptic potential (EPSP). Graded hyperpolarizations bring the membrane potential farther away from threshold. This is referred to as inhibitory postsynaptic potentials (IPSP). Excitatory And Inhibitory Neurotransmitters RECOGNIZE ALL THESE NEURO TRANSMITTERS Excitatory - a neurotransmitter depolarizes the postsynaptic neuron, Chemical Acetylcholine Chemical Structure Neurocrines and Neurotransmitters Receptor Type Whether a neurotransmitter is excitatory or inhibitory depends on its receptor KEY: ICR = ion channel receptor GPLR = G Protein Linked Receptor Functional Class Cholinergic Type (Nicotinic and Muscarinic) Nicotinic Inhibitory – a neurotransmitter hyperpolarizes the post-synaptic neuron Receptor Location Muscarinic ICR (Na+, K+) Skeletal muscles, autonomic neurons, CNS GPLR Smooth and cardiac muscle, endocrine and exocrine glands, CNS Excitatory Excitatory or Inhibitory Norepinephrine, Epinephrine Adrenergic (α, β) GPLR Smooth and cardiac muscle, endocrine and exocrine glands, CNS Excitatory or Inhibitory Dopamine Dopamine GPLR CNS Serotonin Serotonergic ICR (Na+, K+) CNS Generally excitatory Generally inhibitory GABA GABA ICR (Cl-) CNS Inhibitory Glutamate Glutaminergic inotropic (iGluR) NMDA and AMPA ICR (Na+, K+, CNS Ca+) Excitatory Substance P NK1 GPLR Excitatory CNS Excitatory And Inhibitory Neurotransmitters Glutamate is excitatory because its receptor is a ligand-gated Na+ channel GABA is inhibitory because its receptor is a ligand-gated Clchannel Other transmitters (e.g. acetylcholine, norepi/epi) have Gprotein-linked receptors Can be excitatory or inhibitory, depending on the signal transduction pathway and cell type Summation Temporal Summation One EPSP is usually not strong enough to cause an AP However, EPSPs may be summed Temporal summation The same presynaptic neuron stimulates the postsynaptic neuron multiple times in a brief period. The depolarization resulting from the combination of all the EPSPs may be able to cause an AP Spatial summation Multiple neurons all stimulate a postsynaptic neuron resulting in a combination of EPSPs which may yield an AP Spatial Summation (Convergence) Autonomic Nervous System Autonomic Nervous System GANGLIA =CELL BODIES OUTSIDE CNS NUCLEUS =INSIDE THE CNS Talk about the pathway and then describe the response Compare the similarities and the difference between the sympathetic and the PNS=. SIMILARITY PREGANGLIA ARE INSIDE CNS AND MYELINATED, RELEASE ACH, POSTGANLION IS UNMYLINATED THE DIFFERENCES: PARASYMPATHETIC GANGLION IS LONGER IN PNS, PNS IS ACH , SYMPATHEIC NEUROTRANSMITTER IS NE, Efferent Reflex Pathways Preganglionic neuron synapses at sympathetic chain ganglion Postganglionic neuron releases NT (NE/ACh) at varicosities onto viscera/ glands Involuntary Autonomic Motor Reflex (Ex: Sympathetic Chain Ganglia) Efferent Pathways: Somatic vs Autonomic ANS- CHOLINERGIC AND ADRENERGIC RECPTORS CHOLINERGIC =ACH ACH NICOTINIC RECEPTOR NA+ MUSCARINIC M1-M5 GPLR ANS- CHOLINERGIC AND ADRENERGIC RECPTORS CHOLINERGIC =ACH ACH NICOTINIC RECEPTOR NA+ MUSCARINIC M1-M5 GPLR Similarities: Sympathetic vs Parasympathetic Both have preganglionic neurons that originate in CNS Both have postganglionic neurons that originate outside of the CNS in ganglia Both release Acetylcholine (Ach) from preganglionic fibers T1-L2 FOR SYMPTAHETIC PARASYMPATHETIC= CN 3,7,9, 10, S2,S3,S4 Figure 9-6 Differences: Sympathetic vs Parasympathetic PNS SNS Fibers originate from cranial and sacral areas of CNS CN 3, 7, 9, 10 Sacral 2, 3, 4 Preganglionic fibers are longer Very short postganglionic fibers Postganglionic fibers release acetylcholine (ACh) Fibers originate in thoracic and lumbar regions of spinal cord T1 – L2 Most preganglionic fibers are short Long postganglionic fibers Most postganglionic fibers release norepinephrine (noradrenaline, NE) Responses to Cholinergic Stimulation Nicotinic receptors (ligand-gated) open a Na+/K+ channel; excitatory only Muscarinic receptors involve G-proteins; excitatory or inhibitory M1 receptor – common in exocrine glands and in CNS; IP3 pathway M2 receptor – receptors are located in the heart, cause a decrease in cAMP in the cell, inhibition of voltage-gated Ca2+ channels, and efflux of K+ , (inhibitory) M3 receptor – smooth muscle in bronchioles. In blood vessels it causes increased synthesis of nitric oxide by endothelial cells, causing vasodilation, stimulates secretion in many glands; IP3 pathway M4 receptor – found in the CNS; decrease cAMP and produce generally inhibitory locomotor effects M5 receptor – location is not well known Cholinergic Stimulation Muscarinic ACh receptors Nicotinic ACh receptors Ligand-gated ion channels Excitatory only Located on post-synaptic membrane of: All autonomic ganglia All neuromuscular junctions Some CNS pathways Na+ ACh K+ G protein-coupled receptors (influence nearby ion channels) Excitatory or inhibitory Produces parasympathetic effects in the heart, smooth muscles, and glands ACh ACh K+ Na+ Ca2+ close or open or open d K+ open Depolarization Hyperpolarization Depolarization Excitation Inhibition Excitation Excites Postganglionic Neuron Slows heart rate; Vasodilation at GI tract Contracts GI tract smooth muscle Responses to Adrenergic Stimulation Effects can be excitatory or inhibitory All act through G-proteins Alpha adrenergic responses: α1 receptors - vasoconstriction in GI tract, skin, kidney – IP3, DAG, Ca2+ α2 receptors - sphincter constriction in GI tract, inhibit insulin release – reduced cAMP Beta adrenergic responses: β1 receptors - increases HR and force of contraction, increase renin secretion - cAMP β2 receptors - bronchiole dilation, vasodilation skeketal muscle , decrease motility GI tract– cAMP β3 receptors - stimulates lipolysis Blood Vessels α receptor Vasoconstriction Epinephrine Vasodilation β receptor Responses to Adrenergic Stimulation Levels of Autonomic Control The hypothalamus is the main integration center of ANS activity Subconscious cerebral input via limbic lobe influences hypothalamic function Other controls come from the cerebral cortex, the reticular formation, and the spinal cord Functions of Hypothalamus Receives indirect inputs from all sensory systems Controls sympathetic and parasympathetic nervous systems Sends both neural and hormonal outputs to pituitary Coordinates activities of the endocrine and nervous systems Induces emotions and behavioral drives Coordinates voluntary and autonomic functions Regulates body temperature Coordinates circadian cycles 4Fs: feeding, fighting, fleeing, and reproductive behavior