Physiology Module 1 PDF
Document Details
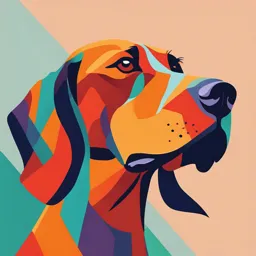
Uploaded by ObservantHeliodor4462
Queen's University
Tags
Summary
This document provides details on the fundamental concepts of homeostasis and excitable cells, exploring the structure and function of the plasma membrane. The text delves into various aspects, including homeostatic control systems, regulation, and different types. It references important biological processes and concepts.
Full Transcript
Module 1 Homeostasis and the Basis for Excitable Cells Section 1: Homeostasis - is the ability of the body to maintain internal environment regardless of the exterior environment Set point - Is the range or point where a variable in the body (example body temperature) tends to stabilize Three ste...
Module 1 Homeostasis and the Basis for Excitable Cells Section 1: Homeostasis - is the ability of the body to maintain internal environment regardless of the exterior environment Set point - Is the range or point where a variable in the body (example body temperature) tends to stabilize Three steps in the homeostatic control system: Sensor - is responsible for detecting the variable in the environment - Temperature monitoring nerve cells Integrator - Compares of variable being detected to the set point - thermoregulation center in the brain Effector - responsible for initiating the changes to restore variables back to the set point - skeletal muscles and smooth muscle in blood vessels Two types of homeostatic regulation: Intrinsically controlled - the sensor integrator and effector are all located within the tissue so the tissue can regulate its own internal environment - an example of this is an exercising skeletal muscle that needs lots of oxygen to produce ATP. when the local oxygen concentration drops blood vessels within the muscles will then dilate (get bigger) increasing the amount of oxygen that can be delivered extrinsically controlled - the regulatory mechanisms are outside of the tissue or the organ (Body temperature) - the majority of homeostatic control systems in the body are dependent upon this control Feedback loops negative feedback - Majority of Homeostatic control systems operate on negative feedback - This is a change in an environmental parameter causing the effector to initiate a response in the opposite direction restoring the parameter to the set point. once the set point is achieved the system will stop signaling Example Blood glucose is regulated through negative feedback mechanisms 1. Homeostasis - the glucose in the blood 2. Imbalance - after you eat something your blood glucose rises 3. Response - insulin is released by the pancreas in response to the high blood glucose levels. Insulin lowers blood glucose by increasing the ability of the body cells to uptake the glucose from the blood 4. Effector - insulin also up regulates the liver's ability to convert the glucose and store it as glycogen Positive feedback - this occurs when the effector causes changes that amplify the initial signals - example decreased body temperature. a positive feedback loop effector would further decrease the body's temperature which would then further activate the sensor - Positive feedback is not homeostatic Example Childbirth 1. The brain stimulates the pituitary gland to secrete oxytocin 2. Oxytocin is carried through the bloodstream to the uterus 3. Oxytocin stimulates uterus contractions which pushes the baby towards the cervix 4. The head of the baby is pushed against the cervix 5. The nerve impulses from the cervix are transmitted to the brain Section 2: Plasma membrane (cell membrane) - The barrier that separates the cells internal and external environments. It does not only serve as a protective barrier for a cell but it also allows cells to maintain an internal fluid composition that is different from the external fluid composition Roles of the plasma membrane 1. Regulates the internal fluid composition as a controls what molecules can move in or out of the cell 2. Allows for nutrients to enter the cell and waste products to leave 3. Permits chemical signals released from other cells in the body to influence a cell allowing cell to cell communication 4. Participates in joining cells together to form tissues and organs Three primary functions of the plasma membrane 1. Ensure cell survival 2. Maintain homeostasis 3. Function cooperatively and coordination with surrounding cells Different components in the plasma membrane Phospholipids - The lipid bilayer is comprised of phospholipids. It has a polar head with a negatively charged phosphate group and two nonpolar fatty acid tails. the head is hydrophilic and the tail is hydrophobic Cholesterol - is found tucked between phospholipids. They prevent the fatty acid change from packing tightly together and forming rigid structures. Cholesterol keeps the membrane fluid Membrane proteins - They are inserted into phospholipid membranes. Can be associated with the inner side, the outer side or pass through the membrane. Proteins function to maintain cell structure, regulate cell function, allow transfer across cell membrane, and facilitate signaling Ion channels - these are specialized membrane proteins which span the entire lipid membrane and permit the entry and exit of ions Carbohydrate chains - Short chains of carbohydrates are attached to the protein or the bilayer itself to form glycoproteins and glycolipids. they are involved in stabilizing the membrane structure, act as a cell surface receptors, participate in transportation across the cell membrane Disorders of the plasma membrane Cystic fibrosis - This is caused by defects in particular chloride ion channels. This channel involves controlling the amount of fluid and mucus within the lungs. People with cystic fibrosis experience a buildup of fluid in the lungs making it difficult to breathe Alzheimer's - Results in oxidative stress and leads to the alteration and degradation of phospholipids within brain cells (neurons) - the reduction of phospholipids affects the Integrity of the membrane therefore impacting the neural function resulting in memory loss and reduced cognitive function Fluid mosaic model - describes the structure of the animal plasma membrane What makes membrane fluid? This is due to the fact the phospholipid bilayer is viscous and the individual phospholipids can move. it is lipid bilayer that provides the membrane with fluidity and elasticity What makes a membrane mosaic? This is due to the fact that it is embedded with proteins and other molecules that perform many functions important to proper cell functioning Cell to cell adhesion Extracellular Matrix (ECM) - A network of fibrous proteins embedded in a gel like mixture of carbohydrates - Surrounds all cells in tissues and keeps them in place even if they're not physically contacting their neighbor - watery gel allows for diffusion of nutrients from the blood and the removal of waste from the cell and is often referred to as interstitial fluid and is comprised of three protein fibers - Collagen: Forms cable like fibers that gives the ECM tensile strength - Elastin: A rubber like protein that allows tissues to be stretched and then recoiled after the stretching force is removed - Fibronectin: promotes cell adhesion Cell adhesion molecules (CAM) - These are usually transmembrane proteins - The intracellular side of the protein interacts with the cytoskeleton, while the extracellular side interacts with the extracellular matrix from other cells - CAM’s are involved in protein protein interactions as they bind with other cells or with the ECM. CAMs help sell stick to each other and they're surroundings Cell Junctions Desmosomes (adherens junctions): - Used to Anchor together to adjacent cells that are not in direct contact. they are composed of plaques that are connected by glycoprotein fragments containing cadherins to attach neighboring plaques together - In a cell other cytoskeletal anchoring proteins attach to their plaques. combined this allows for a network of strong fibers extending through tissues and through cells. This allows desmosome containing cells to have the ability to stretch Tight junctions (impermeable junction) - This creates a tight seal between cells preventing movement of molecules from cell to cell. Long strings of junctional proteins in the plasma membrane between them bring cells aligned and adhere to form a tight junction. - The area where opposing junctional proteins from neighboring cells meet is called a kiss site - This type of junction is found in epithelial tissue which allows epithelial sheets to form high selective barriers between compartments with different chemical composition - Example: the epithelial lining of the digestive tract which prevents blood vessels from being directly exposed to the digestive juices and enzymes. nutrients absorbed by epithelial cells are then transported directly to the blood and the tight junctions prevent the movement of substances between adjacent epithelial cells Gap Junctions (communicating junctions) : - Within a plasma membrane, six connexin protein subunits form one half of a gap junction (connexon). When this aligns with the connection of the adjacent cells it forms a tunnel that connects their intracellular spaces and allows them to communicate directly. - Because they're diameter is very narrow, only small water soluble substances can pass through the Junctions. tunnels can be opened and closed as needed to control cell to cell communication. - Gap junctions are common in cardiac and smooth muscle cells that rely on these junctions to spread the wave of excitation throughout the entire tissue. - Gap Junctions allow the spread of secondary messengers between connected cells which provides mechanisms for cells to act in a cooperative manner Membrane Permeability Permeable - A substance can freely move across the membrane Impermeable - A substance cannot cross the membrane Semipermeable - Some of the substance can pass freely while others cannot What determines membrane permeability? Size Small substances like ions can enter through the membrane spanning ion channels while larger substances such as glucose require a transport protein to move it across the membrane - GLUT -1 Is one of many glucose transport proteins. When glucose enters channel protein changes configuration allowing glucose to enter the cell Solubility A prime factor determining membrane permeability is how lipophilic the substance is. Uncharged or nonpolar molecules are very lipophilic and can easily cross the plasma membrane. Charged or polar substances are lipophobic and cannot pass through the plasma membrane unaided Crossing the Membrane Substances that can freely penetrate and cross the membrane on their own are driven by two primary forces: Concentration gradient, electrical gradient Diffusion down concentration gradient: - Molecules in solutions are constantly moving. When their density is high they frequently collide and bounce off each other. This causes molecules to spread from areas of high density to areas of low density or down the concentration gradient - This process is known as diffusion and will continue until the concentration gradient no longer exists. When this occurs the movement of substances are in a steady state or dynamic equilibrium with no movement Diffusion in the presence of a membrane: membrane permeable - if the membrane is permeable to the substance then there will be net diffusion of the substance passively down its concentration gradient across the membrane until equilibrium has been achieved membrane impermeable - If the membrane is not permeable to the substance then no diffusion will occur in the concentration gradient will remain in place Osmosis - water molecules are small enough that they can cross the plasma membrane by moving between the phospholipid molecules. Many cells have specific membrane proteins called aquaporins. since water molecules can move across the plasma membrane water moves down its concentration gradient by diffusion and this net diffusion of water is known as osmosis - - diffusion refers to the concentration of a substance in a solution. As water is also a substance it has a concentration. - In pure water 100% of the solution is water. when you add a substance water is displaced and it's concentration decreases Unequal Solutions and penetrating solute - Osmosis occurs when the membrane separates unequal Solutions. it can occur simultaneously with a diffusion of a penetrating solute Since the membrane is permeable to the substance it will move by diffusion down the concentration gradient and the water will move by osmosis down the concentration gradient. the net results is that both the substance and water will be equally distributed across the membrane Unequal Solutions and non-penetrating solute - This membrane is not permeable to the substance. the substance will remain inside to but the water will move by osmosis down the concentration gradient. the net rest is the water moves to the side to dilute the substance until the concentration of the substance and water are the same for both sides of the membrane. - it is important to note though the volumes are not the same on both sides membrane - this movement of fluid occurs in cells and can cause cells to either shrink or swell depending on which way the net movement of water occurs Pure water and non penetrating solute - Osmosis occurs when a membrane separates pure water from a solute of a non-penetrating solute Since the membrane is not permeable to the substance the substance will remain inside side 2 and water will move by osmosis down a concentration gradient increasing the volume inside side 2. however since there is no substance in side one a concentration equilibrium cannot be achieved but this doesn't mean that all the water will eventually be on side two EXPLAINED Osmotic pressure The underlying force that was watered down its concentration gradient is called osmotic pressure. the greater the gradient the greater the osmotic pressure trying to move water across the membrane Hydrostatic pressure hydrostatic pressure is a force created by a given volume of water. the greater the volume the greater the hydrostatic pressure. in the above example at steady state we have not achieved an equilibrium of a substance concentration but we have achieved an equilibrium of pressures At steady state the osmotic pressure in side one is equal to the net hydrostatic pressure in side two. this results in no net movement of water even though a concentration gradient still exists Carrier mediated transport Facilitated diffusion - Does not require energy and uses a carrier to assist in the transfer of a substance down its concentration gradient - Example: transporting glucose into cells - Glucose is a vital substrate for the production of cellular energy in the form of ATP however the plasma membrane is impermeable to glucose - glucose carrier proteins bind to extracellular glucose and transport it into the cell. because glucose is constantly being consumed to produce ATP as cell maintains the concentration gradient to move glucose inside How can solutes enter the cell via carrier mediated transport? 1. carrier protein takes a confirmation in which the solute binding site is exposed to the region of higher solute concentration 2. Solute molecule binds to the carrier protein 3. Carrier protein changes conformation so that the binding site is exposed to a region of lower soil concentration 4. Transported solute is released and the carrier protein returns to confirmation as seen in step one Active transport - Also use the carrier protein to assist in the transfer of a substance but in this case it moves a substance against its concentration gradient - active transport counters the concentration dependent forces of diffusion therefore this form of movement requires energy in the form of ATP Example: the sodium potassium pump - the sodium concentration is much higher outside the cell compared to the inside whereas a concentration of potassium is much higher inside of the cell - these concentration gradients are maintained by the sodium potassium pump which transports sodium out of the cell and the same time brings potassium in How does the sodium potassium pump work? 1. The sodium potassium pump has three high affinity sites for Na and two affinity sites for K when exposed to intracellular fluid (ICF) 2. When three Na from the intercellular fluid binds to the pump it splits ATP into ADP and phosphate. the phosphate group binds to the pump 3. Phosphorylation causes the pump to change confirmation that the sodium binding sites are exposed to the opposite side of the membrane and three Na are released to the extracellular fluid (ECF)( high sodium concentration) as Affinity of sodium binding sites greatly decreases 4. Change in shape also exposes the pump's binding sites for potassium to extracellular fluid and greatly increases affinity of potassium sites 5. When two K from ECF (Where K concentration is low) bind to the pump it releases the phosphate group. Dephosphorylation cause of the pump to revert to its original confirmation 6. Two K are released to ICF (K concentration is high) as affinity of potassium binding sites decreases during change in shape. at the same time affinity of sodium binding sites greatly increases returning the process to step one Characteristics of carrier mediated transport Specificity: Each carrier protein is specialized to recognize and transport a specific substance. often structurally related substances may share the same transporter Saturation: For a given cell they're only so many of each type of carrier protein in the plasma membrane. Because of this there is a maximum amount of substance that can be transported at any given time. Once the transfer maximum has been reached no other forces can increase the amount of substance being transported. Competition: As mentioned with specificity, sometimes several related substances can be recognized by a carrier protein. when this occurs no individual substance will be able to achieve its own transport maximum while in the presence of the other substances competing for the same carrier Vesicular transport - Sometimes there are no specific mechanisms in place to move certain ions or large molecules in and out of cells. when this occurs cells are able to transport substances by wrapping them in a membrane and closed vessel - vascular transport requires the expenditure of energy and is considered a form of active transport Endocytosis which is classified in three ways Pinocytosis: The cell membrane engulfs and internalizes small droplets of extracellular fluid. membrane deforming cope proteins on the inner side of the plasma membrane cause the formation of an endocytic pouch that is pinched off by the protein dynamin. Results in an internalized vessel filled with extracellular fluid Receptor mediated endocytosis: is similar to pinocytosis except that the trigger to create a vessel is dependent upon The Binding of substance to a specific receptor on the cell surface Phagocytosis: involves the internalization of large multimolecular particles and only occurs in a few specialized cell types such as white blood cells Exocytosis: - This is the reverse of endocytosis in that a membrane enclosed vessel that was formed within the cell fuses with the plasma membrane and releases its content into the extracellular environment - materials produced by the endoplasmic reticulum and the Golgi complex which are destined for the plasma membrane used this process for two purposes 1. Provides a mechanism to release large polar molecules such as protein hormones that cannot cross the plasma membrane. often the release of the vessels is dependent on a cell receiving a particular signal to release the substances in the vessel 2. Enables a cell to move protein such as care proteins, ion channels, receptors. when the vessel membrane fuses with the plasma membrane any protein in the vessel membrane becomes part of the plasma membrane Steps in exocytosis: 1. secretion vessel formation - Recognition markers in the membrane of the outermost Golgi sack captures the appropriate cargo from the Golgi lumen by binding only with the Sorting signals of the protein molecules to be secreted. this causes the membrane to curve forming a bud 2. Budding from the Golgi - The membrane closes beneath the bud pinching off the secretory vessel 3. Uncoding - The Vessel loses it's coding exposing v-SNARE docking markers on the vessel surface 4. Docking at plasma membrane - the v-SNARE bind only with t-SNARE Docking marker acceptors of the targeted plasma membrane ensuring that secretory vessels empty their contents to the cells exterior 5. Exocytosis Section 3 Ohm’s Law: V=IR V= voltage (membrane potential) I= current (ions moving across the membrane) R= resistance (ability of the plasma membrane to resist ion movement across it) - Ions move across the membrane through ion channels Ion channels : Ion channels are large transmembrane proteins that open to allow ions to enter or exit the cell generally down the concentration gradient. there are four main iron channels: Voltage gated: these ion channels open and close in response to changes in the membrane potential. They can be closed at resting potential, open in response to nerve impulses, and inactivated for a brief period following activation Chemically gated: these ion channels open with a specific chemical messenger interaction. chemically gated ion channels can be found in dendrites of neurons Mechanically gated: these ion channels open in response to Mechanical deformation such as stretch. for example a mechanically gated ion channel are those which are found in the cochlea of the ear Thermally gated: These ion channels respond to changes in temperature. these are present and specialized neurons that project the skin to act as temperature detectors Voltage gated ion channels - Voltage gated ion channels are specialized to respond to changes in electrical potentials across the membrane. they are voltage gated because they contain voltage sensors which respond to changes in voltage and causes the Ion channel to undergo a conformational change - This opens up pores in the channel to allow ions to flow across the membrane. ion channels also have a selectivity filter that determines what kind of ion can pass through the poor and it is this selectivity that allows us to classify on channels. - Example of voltage-gated ion channel that is selected for k+ is called a voltage-gated k+ channel Voltage-gated ion channels and Ohm's law Step 1: start at a membrane potential (V) where the voltage sensor keeps the channels closed. when the channels are closed the ions cannot really move across the membrane meaning the membrane has a high resistance (R) to ion movement. applying Ohm's law ionic movement or current (I) is very low * When V is constant a large resistance means a small current Step 2: If the membrane potential changes to a voltage that causes the voltage sensors to open the channels the channel pores decrease the membrane's resistance to ion movement. resistance becomes smaller and applying Ohm's law current increases. * Once R decreases I increases as ions flow across the membrane Step 3: At any given voltage when resistance increases current decreases and when resistance decreases current increases Potassium's electrochemical gradient - Because ions are not evenly distributed across the membrane and electrical gradient is created due to the different concentrations of cations and anions. - Focusing on K plus which has an extracellular concentration of 5 mM and an intracellular concentration of 150mM. both electrical and concentration gradients act as driving forces for the movement of potassium but they do not always act together Concentration gradient Potassium has a concentration gradient across the membrane. The gradient increases a driving force as ions want to move down their concentration gradient. In this example the high intracellular fluid concentration of potassium pushes potassium out of the cell into extracellular fluid Electrical gradient When discussing excitable cells and ions we need to consider the effect of the ionic charge. The inside of cells is more negative due to the presence of non permeable ions such as charged proteins. This negative charge creates an inward electrical gradient or driving force as it tries to prevent positively charged k+ from leaving the cells. Electrochemical gradient This is a combination of the concentration and electrical gradients. These individual forces can work together or in opposition to each other. In the example the driving force from the concentration gradient is greater than the electrical gradient. This causes potassium to flow out of the cell. The Nernst equation This allows us to calculate the equilibrium potential (E) Also called the reversal potential which is the membrane potential of which there is no net flow of an ion across the membrane due to a balance between the electrical and chemical gradients. E = Equilibrium potential for an ion in mV R = universal gas constant T = absolute temperature (in K) z = valence of the ion (eg +1) F = Faraday constant C = concentration inside (Ci) and concentration outside (Co) the cell (mM) For K, Na, Cl all have valence that reduces to 61 Simplify to: E values Are important in that they determine for any given voltage if the net driving forces move ions into or out of a cell. The net flow of ions will always be in the direction which brings a member potential towards the E. the equilibrium potential of an ion can also be called reversal potential Calculating the resting membrane potential Rmp is dependent upon the types of ion channels found in membrane, the concentration of ions on both sides of the membrane, and the related permeabilities at that time Goldman's equation The related permeabilities for K, Na and Cl are 1, 0.04 and 0.45 Terminology for membrane potential changes: - When a membrane is not at 0mV it's said to be polarized and a neuron at rest is usually polarized to about -70mV Depolarization: the magnitude of this polarization decreases that is it moves towards 0mV the membrane is said to be depolarizing Repolarization: after either a depolarization or hyperpolarization once polarizing begins to return towards the RMP than the membrane is said to be repolarizing Hyperpolarization: if the magnitude of this polarization increases that is moves even more negative than the RMP than the membrane is said to be hyperpolarizing Graded potentials - These are local changes in the membrane potential that are used for short distance signaling. they tend to be small and magnitude but can change in magnitude or strength - Example: if the membrane changes from negative 70 to -60 it is a 10 graded potential. - Graded potentialS are different than action potentials Spread of depolarization - Graded potentials are caused by triggering events that make voltage-gated ion channels open. Even though a triggering event occurs at a very localized area of the plasma membrane this change in potential can actually spread along a membrane. this spreading of depolarization does not occur from charge movement across membrane but rather charge movement along the membrane - Example: if a triggering event causes a local 10 mV depolarization then the inside of the membrane in that area is - 60 mV while the surrounding membrane is still - 70 mV. this happened because positive charge across the membrane to cause the depolarization. these positive charges will now spread out inside the cell and cause a spreading of depolarization among the membrane Graded potentials and current loss As the wave of depolarization moves along the membrane there is a loss of charge. the magnitude of the graded potential decreases as it moves away from the site of the initial triggering event until it eventually disappears. Section 4: Action potential - Action potentials are caused by a triggering event or stimulus that can result in a localized depolarization however when action potential is triggered it will conduct or propagate throughout the entire membrane and does not diminish in strength - it can be used for long distance signaling I feel like I cover all - Action potential Begins by a triggered event - if it is not strong enough the membrane potential will quickly be polarized and the action potential does not occur. - in order for it to occur the triggering events magnitude has to be sufficient that it reaches a certain potential - this potential is called the threshold and once it is reached there is a rapid depolarization as the membrane potential becomes positive - just as quickly the membrane potential depolarizes to the resting membrane potential - In some cases this repolarization goes more negative than rmp and is called an after hyperpolarization before returning to the rmp What causes change in permeability? Voltage gated K channels and voltage gated Na channels change the membrane permeability of the respected ions The conformation of voltage gated ion channels Closed - When an ion channel is closed, the activation gate within the pore keeps the pore closed - The inactivation gate is inside the cell but does not interact with the pore. In this state the ion is said to be closed and no Na can enter the cell Open - Upon reaching the Threshold potential the voltage sensor allows the activation gate to open. sodium then rapidly enters the cell because the channel is the open state. this is the process of activation Inactive - Shortly after the channel is open the intracellular inactivation gate moves to block the inside of the poor to stop the na from entering the cell. it is important to note that the activation gate may still be open but because the channel is inactivated no current will flow. Structure of sodium and potassium ion channels - The structure of ion channels can vary quickly - voltage gated Na channels are one large transmembrane protein with a single activation gate - Voltage gated K channels have four subunits each which contribute to activation and inactivation of the channel as a whole Potassium channel Sodium Ion channels Action potential step by step At rest At rest the majority of the voltage gated Na and K channels are closed but they're capable of opening. incoming graded potentials can cause depolarizations which if strong enough can depolarize the membrane potential to the threshold Threshold and Rising phase when a triggering event reaches a threshold voltage gated na channels open. this opening results in a dynamic increase in Na permeability. because the permeability is now greater than that of K the memory potential rapidly approaches the na equilibrium potential. voltage-gated K channels are also open at this time but their contribution is minimal The falling phase After opening voltage-gated na channels inactivate as the inactivation gate swings to block the poor. This decreases permeability again but since voltage-gated K channels are still open Cape permeability is higher and the memory potential will now move towards the K equilibrium potential. resting membrane potential is restored Can a neuron have multiple action potentials? It depends on the state of the voltage-gated Na channels. The ability of a membrane to have an action potential depends on how many channels are inactive. after opening the inactivation gate block support and the channels become inactive. when the membrane repolarizes to the resting membrane and potential it takes time for the inactivation gate to move out of the poor and allow the any channels to move from “inactive” to “closed and ready to open” Section 5 The Neuron Neurons are the key cell type of the nervous system. There are four functional zones involved in the transmission of neural impulses 1. Input zone: Part where incoming signals are received a. Dendrites: Numerous projections from the cell body that receive electrical and chemical inputs b. Cell body: Location of the nucleus and other organelles 2. Trigger Zone: part where action potentials are initiated. a. Axon hillock: Where the axon leaves the cell body 3. Conducting Zone: Where action potentials are conducted to their Target locations. a. Axon: Tubular extensions that conducts the actual potential away from the body 4. Output Zone: Part that releases chemical Messengers. a. Axon terminals: Release chemical Messengers to communicate with other cells Transmission Down an Axon (Conduction) - When an action potential is triggered at the Axon hillock, nerve conduction or transmission of the action potential down the axon is initiated. This however is not just a single action potential which is transmitted down the entire Axon - conduction is the result of an action potential triggering a new action potential in an adjacent area - When an action potential is initiated the inside of the membrane becomes more positive. This positive charge influences adjacent areas of the membrane and brings them from resting to threshold potential therefore triggering a neighboring action potential. this new action potential will trigger another adjacent action potential and so on down the entire Axon. - because it is not one action potential spreading down the axon there is no signal Decay as the magnitude of the signal is refreshed with each new action potential One way propagation How can an axon ensure one way propagation? There's an absolute refractory period and related refractor appeared for Action potentials which is caused by sodium channels or meaning inactive after depolarization. this prevents an action potential from being generated in the opposite direction Refractory periods One Way propagation is insured by the concept of refractory periods. when the action potential is initiated in the action hillock and then triggers a subsequent series of action potentials. however when the net actually potential is triggered the previous action potential is still finishing and the voltage-gated na channels are still inactive. because they're inactive they cannot be immediately reopened and the way of excitation moves away from them - Refractory periods can be absolute where under no conditions another action potential can be triggered or relative where it is difficult but if the triggering stimulus is strong enough and acts potential may be triggered - Refractory periods maybe different from neuron to neuron and play a critical role in determining neuronal firing rates. this is important as the frequency of action potentials is key feature of neuronal communication An example of firing frequency increasing is when stimulation increases in intensity. firing amplitude does not change with signaling intensity The speed of conduction: once an action potential is initiated its rate of conduction down the axon is determined by whether or not the fiber is myelinated and the diameter of the fiber. myelination and axon diameter are the two factors which affect conduction speed Myelin - Milan is formed by specialized cells ( oligodendrocytes and schwann cells) In the peripheral nervous system and nerves leaving the spinal cord going to the body systems that physically wrap themselves around the nerve fiber. the fibers are not uniformly coated as there are regions of exposed fiber at regular intervals these are called the nodes of ranvier - Myelinated regions are very lipid-rich and provide extra insulation to prevent current leakage. In myelinated fibers Action potentials can only be generated in the nodes of ranvier where the fiber is exposed to the extracellular fluid. this region is also where na channels are densest Myelination and saltatory conduction - In non-myelinated fibers the wave of excitation moves down the entire Axon. in myelinated fibers the wave of excitation jumps from one node of ranvier to the next this is called saltatory conduction and allows myelinated axon to conduct impulses up to 50 times faster than the unmyelinated axons Diameter and conduction velocity - When the diameter of a fiber is increased the resistance to progenating local currents decreases and the fibers can conduct at a faster rate - Axons have a maximum diameter they can reach. size alone is not the only determining factor in Faster signaling. fast reflexes such as those involved in reflexes would have a well-myelinated neuron with a large diameter activating the fastest rate of propagation Synapses: This is the junction between a presynaptic neuron and a postsynaptic neuron and includes the synaptic cleft between the two neurons Presynaptic neuron: The neuron from which the action potential is coming from Neurotransmitters: chemicals released into the synaptic cleft which activate ion channels on the postsynaptic neurons membrane Synaptic cleft: the area of extracellular fluid between the pre and postsynaptic neurons Postsynaptic neuron: The neuron to which the action potential travels Synaptic vessels: Vessels which contain neurotransmitters. These vessels released in neurotransmitters into the synaptic cleft - Synaptic transmissions are not electrical but rather chemical in nature. this is because chemicals called neurotransmitters across the synapse instead of an electrical current Mechanism for synaptic transmission 1. When electrical action potential reaches the axon terminal in the presynaptic neuron the charge in membrane potential opens a class of voltage-gated ion channels Called the voltage-gated ca2+ Channel. once opened it flows down its concentration gradient into the cell 2. Calcium flows into the axon terminal triggering exocytosis of synaptic vessels containing neurotransmitters 3. Neurotransmitters then diffuse across the synaptic cleft and interact with specific receptors on the postsynaptic neuron. The Binding to receptors opens chemically gated ion channels through which ions flow into the cell and can modulate the postsynaptic membrane potential Postsynaptic potentials Neurotransmitters interact with chemically gated ion channels embedded in the membrane of the postsynaptic cell. This creates a graded potential which if strong will travel to the axon hillock. Graded potentials can either stimulate or inhibit an action potential and are called excitatory postsynaptic potential (EPSP) and inhibitory postsynaptic potentials (IPSP) Excitatory Synapses The interaction of a neurotransmitter with its receptor opens nonselective cation channels that allow the postsynaptic movement of both Na and K. although K will likely exit the cell down its concentration gradient, the next flux of cations is inwards which results in a slight depolarization. This depolarization is a graded depolarization and generally will not result in an action potential firing. This slight depolarization brings the membrane potential closer to its threshold and therefore called an excitatory postsynaptic potential (EPSP) Inhibitory Synapses - Inhibitory synapses generally results when the neurotransmitter interacts with its receptor to activate either Cl channels or K channels - Increasing K permeability allows for K to move down its concentration gradient and flow out of the cell, while increasing Cl permeability allows Cl to move down its concentration gradient and enter the cell. Both of these events causes the inside of the cell to become slightly more negative - These small hyperpolarizations move the membrane potential away from the threshold and therefore called inhibitory postsynaptic potentials (IPSP) As it would now take a stronger excitatory input to trigger an action potential. Summation - Graded potentials will diffuse towards the axon hillock where they impact the membrane potential. Unless at rest the axon hillcox membrane potential will be the summation of all arriving or recently arrived graded potentials - The net effect on the postsynaptic neuron is the sum of those inputs on the membrane potential. if the EPSP outweighs IPSP and is sufficient to reach threshold then the postsynaptic neuron will fire and transmit the signal. if I PSP is predominant than the signal will not propagate Temporal summation This is the summing of several EPSP occurring close together in time due to the repetitive firing of a simple presynaptic neuron. each EPSP has an additive effect before the membrane return to the resting membrane potential In the diagram how a single AP from the presynaptic neuron was not strong enough to create an action potential In the postsynaptic neuron. however when it was stimulated multiple times and action potential formed Spatial summation This is the summation of EPSP and IPSP originated from several different presynaptic inputs having simultaneous effects on the membrane potential. this is demonstrated in the diagram. EPSP from both excitatory presynaptic neurons alone were not strong enough to cause the Axon hillock to reach the threshold potential however when they occurred simultaneously threshold was reached and AP occurred. an excitatory and inhibitory PSP occurred at the same time they canceled each other out and they were no change in membrane potential