Transepithelial Transport in Kidney PDF
Document Details
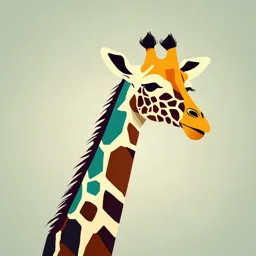
Uploaded by StableTheory
University of Cape Town
Prof Geoff Rogers
Tags
Summary
This document provides an overview of renal tubular epithelium cell processes, illustrating fundamental cellular mechanisms involved in transport systems. An emphasis is given to renal sodium handling.
Full Transcript
Stratified - variable in thickness acording to location. 2-3 layers in minor calyces 5-6 in bladder. The cells can stretch and shift over each other and flatten out to accommodate a full bladder. A full bladder may appear to have only 2-3 layers of flat cells. While in an empty bladder the transitio...
Stratified - variable in thickness acording to location. 2-3 layers in minor calyces 5-6 in bladder. The cells can stretch and shift over each other and flatten out to accommodate a full bladder. A full bladder may appear to have only 2-3 layers of flat cells. While in an empty bladder the transitional epithelium appears as a cuboidal basal layer, polygonal middle layer and a convex often binucleate tall upper layer. The surface has plaques -modified areas of plasma membrane which unfold to allow stretching of the organ. The umbrella cells of the surface layer are sometimes binucleate and have a refractile border. Ureters Lined by a transitional epithelium with a lamina propria consisting of fibroblasts and connective tissue. Two spirally arranged muscle layers with a third layer at the lower end towards the urinary bladder and an adventitia and serosa. Urinary Bladder Transitional epithelium with 3 spiraled muscle layers, longitudinal, circular, longitudinal. An internal sphincter is formed at the urethra. Sympathetic fibres are found in the adventitia and parasympathetic fibres are found in the adventitia and muscle. These fibres form the efferent fibres of the micturation reflex. Sensory fibres from the bladder muscle to the sacral portion of the spinal cord form the afferent fibres of the micturation reflex. Female urethra Lined with stratified squamous epithelium and a lamina propria which is vascular containing mucous secreting glands. The external sphincter is of striated muscle from the mid portion of urethra, which passes through the striated muscles of the pelvic floor Male urethra 20-25cm long. It has 2 functions, void urine and the final conduit of male reproductive system, and 3 segments Prostatic from the neck of the bladder through the prostate where it receives the openings of the ejaculatory ducts - many peri urethral glands open into it. It is very thin here. Membranous urethra 1cm runs through the pelvic floor- has external sphincter of striated muscle Penile urethra - small mucous glands open into it Lined with transitional epithelium, stratified columnar, stratified cuboidal and stratified squamous epithelium progressively. REFERENCES MH Ross, W Pawlina; Histology: A Text and Atlas, 5th ed Juncquiera’s Basic Histology: Text and Atlas. 12 th ed. Available via the library e-books portal. Physiology LT 05.01.02 Renal Function and Transport Processes - Author: Prof Geoff Rogers Aim To provide an overview of the processes by which renal tubular epithelium cells modify the fluid delivered into the lumen by glomerular filtration. The fundamental cellular mechanisms involved in these transport systems will be illustrated. Particular emphasis will be on the renal handling of sodium. 10 Delivery objectives 1. Describe the principles of transepithelial transport systems for water, electrolytes and organic solutes. 2. Describe the transport systems in key nephron segments: proximal tubule, thick ascending limb of Henle’s loop, distal tubule and collecting duct. 3. Explain the regulation of tubular transport processes: physical factors, neural mechanisms and hormonal control. Content RENAL TRANSPORT PROCESSES 1. Main Renal Functions I. Sodium and water reabsorption II. Electrolyte balance III. Acid base balance 2. Basic Elements of the Renal Transport Process I. The key element and the primary active process in renal cellular transport is mediated by sodium (Na), potassium (K) – ATPase situated at the baso- lateral membrane. II. In the process 3Na+ are exchanged for 2K+ within the cell and additionally some K+ filters back into the interstitial space resulting in a net negative charge (-70mv) inside the cell. III. The sodium, potassium pump maintains a decreased cellular sodium concentration resulting in a favourable gradient for the movement of sodium from the tubular fluid into the cell 3. Proximal Tubule 3.1 First half of proximal tubule i) Sodium reabsorption is coupled with H+ (antiport mechanism) (Fig 1) or organic solutes mainly glucose and amino acids (symport mechanism) (Fig 1). The accompanying filtered anion HCO3- has no direct transporter and requires a different process, involving carbonic anhydrase (CA), for its effective reabsorption. Figure 1: Reabsorption of sodium using symport and antiport mechanisms. 11 ii) The coupled process for Na reabsorption is associated with specific transport proteins (facilitated transport) along concentration and electrochemical gradients (secondary active process) iii) The transport proteins operate secondarily to Na,K- ATPase situated at the basolateral membrane of the cell which is the primary source of energy for renal transport processes. In the reabsorption of of sodium by the kidney, Na,K- ATPase is responsible for setting up concentration and electrochemical gradients which favour the reabsorption of sodium at the luminal surface of the renal tubule. 3.2 Second half of proximal tubule i) In association with Na, K- ATPase, sodium enters the cell luminal membrane via a parallel operation involving a Na+-H+ antiporter and a Cl—Anion antiporter (Fig 2). The secreted H+ and anion (OH- , HCO2- , HCO3- etc) combine in the tubular fluid and re-enter the cell by diffusion. The net result is uptake of NaCl. Figure 2: Reabsorption of sodium via a parallel operation (second half proximal tubule). ii) At the basolateral membrane, sodium leaves the cell via Na, K- ATPase and chloride leaves via a K, Cl- symporter. iii) NaCl is also reabsorbed via a paracellular route. Because effective HCO 3- reabsorption is extensive in the first half of the proximal tubule, tubular fluid in the second half of the proximal tubule has high concentrations of Cl -. The high concentration of Cl- in the tubular fluid results in a concentration gradient with Cl - in the interstitial space and the movement of Cl - out of the tubular fluid. With the loss of Cl- the tubular fluid becomes positively charged (relative to the ISF) facilitating the movement of Na+ out of the tubular fluid. 3.3 About 67% of the filtered NaCl is reabsorbed by the proximal tubule. About two thirds of this is reabsorbed via a transcellular route and about one third via the paracellular route. 3.4 Water reabsorption by proximal tubule 12 I. About 67% of the water in the filtrate is reabsorbed in the proximal tubule. II. The driving force for water reabsorption is the transtubular osmotic gradient established by solute reabsorption (NaCl, glucose, HCO 3-). Reabsorption of these substances into the interstitial space results in increased osmolality of the interstitial fluid and decreased osmolality of the tubular fluid. Water moves along the osmotic gradient into the interstitial space mostly via the paracellular route (Fig 3). The reabsorption of water is isosmotic so that the osmolality of the tubular fluid remains the same as plasma in the proximal tubule. Figure 3: Reabsorption of water in proximal tubule. iii) Once the water has accumulated in the interstitial space by osmosis, it moves by filtration into the blood vessels dependant on the filtration forces. Interstitial fluid hydrostatic pressure: 6mmHg Colloid osmotic pressure peritubular capillaries: 32mmHg Force moving filtrate into capillaries: 38mmHg Peritubular capillary hydrostatic pressure: 13mmHg Interstitial fluid colloid osmotic pressure: 15mmHg Force apposing moving filtrate into capillaries: 28mmHg Net force moving filtrate into capillaries: 10mmHg 4. Loop of Henle 4.1 Descending limb of the loop of Henle i) Reabsorption of water but not sodium occurs resulting in an increasing sodium concentration within the tubule up to its loop. 4.2 Ascending limb of the loop of Henle 4.2.1 Thin ascending limb i) In the thin ascending limb of the loop of Henle, sodium diffuses out along a concentration gradient and it is impermeable to water resulting in a decrease in the osmolality of the tubular fluid. 4.2.2 Thick ascending limb (TAL) 13 i) In the thick ascending limb of the loop of Henle (TAL), Na,K- ATPase plays the same role in the reabsorption of sodium as ocurrs in the proximal tubule (primary active process). ii) Sodium reabsorption at the apical (luminal) membrane is mediated by the Na+- 2Cl- - K+ symporter which is the carrier protein inhibited by loop diuretics (Fig 4). Figure 4: Reabsoption of sodium by the thick ascending limb of the loop of Henle. iii) Also present in TAL cells is a Na+ - H+ antiporter situated at the apical membrane which facilitates the reabsorption of sodium and the secretion of H + by a secondary active transport process (Fig 4). Associated with this process is also the reabsorption of HCO3- (Fig 4). iv) Potassium and chloride leave the cell at the basolateral membrane by facilitated diffusion. v) The tubular fluid in TAL is positively charged because of the increased Cl- transport in the second half of the proximal tubule and also within TAL. The positive charge in the tubular fluid causes the reabsorption of cations, mainly Na +, but also Ca++, K+ and Mg++ across the paracellular pathway. vi) TAL is also not permeable to water. 5. Distal Tubule and Collecting Ducts 5.1 Early distal tubule i) Na,K- ATPase has the same action as in the other renal cells. In the case of the early distal tubule, its actions result in the reabsorption of Na + and Cl- at the apical membrane mediated by a Na+- Cl- symporter (Fig 5). 14 Figure 5: Reabsorption of sodium by the early distal tubule. ii) Sodium as usual leaves the cell at the basolateral membrane via the action of Na,K- ATPase. Chloride leaves the cell by diffusion through chloride channels. iii) The Na+- Cl- symporter is inhibited by thiazide diuretics. iv) The early distal tubule is also impermeable to water resulting in the continuation of tubular fluid dilution. 5.2 Late distal tubule and collecting ducts 5.2.1 Principal cells (Fig. 6) Figure 6: Activity in the Principal and Intercalated cell of the late distal tubule and collecting duct. i) The activity of Na,K- ATPase is the same as in the other cells of the renal tubules. The late distal tubule and collecting duct reabsorb about 7% of filtered sodium and 8-17% of filtered water. ii) Sodium reabsorption generates a tubular lumen negative charge within the late distal tubule and collecting duct. The tubular negative charge is the driving force for chloride reabsorption across the paracellular route. iii) Sodium enters the cell at the apical membrane by diffusion along a concentration and electrochemical gradient through sodium selective channels (ENaC). Potassium leaves the cell at the apical membrane by passive diffusion along a concentration gradient into the tubular fluid via potassium channels. 15 5.2.2 Intercalated cells (Fig 6). i) These cells secrete H+ normally or HCO3- by modified intercalated cells in the event of a metabolic alkalosis. ii) Although not shown in Figure 6, Intercalated cells reabsorb K + through the activity of H+,K+- ATPase which is located at the apical membrane of the cell. The release of H+ into the tubular fluid to a greater or lesser extend depends on the acid- base status. 6. Aldosterone (Fig. 7) Figure 7: Action of Aldosterone. i) Secreted by the glomerulosa cells of the adrenal cortex. ii) It stimulates sodium reabsorption mostly in the Principal cells of the late distal tubule and collecting ducts by increasing the amount of Na,K-ATPase at the basolateral membrane and by increasing the expression and activation of the sodium channel (ENaC) at the apical (luminal) membrane. iii) For every Na+ reabsorbed a K+ is secreted. iv) Aldosterone secretion is stimulated by angiotensin II and hyperkalaemia and inhibited by hypervolaemia, hypokalaemia and natriuretic peptide. iv) Through its stimulation of sodium reabsorption, aldosterone also increases water reabsorption and plays a critical role in maintaining fluid balance. The reabsorption of water is isosmotic. 7. Reabsorption of Bicarbonate (Fig. 8) 16 Figure 8: Mechanisms in the reabsorption of bicarbonate by the kidney. i) Reabsorption of HCO3- requires secretion of H+. This H+ secretion is dependent on the actions of a Na+- H+ antiporter and a H+- ATPase at the apical membrane of the proximal tubular cells and of the Na+- H+ antiporter at the apical membrane of TAL tubular cells and also the H+, K+- antiporter and H+- ATPase at the apical membrane of the Intercalated cell found in the late distal tubules and the collecting ducts ii) In the process CO2 is produced in the tubular cell as part of normal metabolic processes or it diffuses into the tubular cell from the tubular fluid or the interstitium. iii) The CO2 combines with H2O catalysed by carbonic anhydrase (CA) to produce carbonic acid. The carbonic acid dissociates into H+ and HCO3- (Fig. 8). iv) In the tubules where it occurs, bicarbonate exits the cell across the basolateral membrane and returns to the peritubular blood. Its exit is mediated by a Na+- 3HCO3- symporter and a Cl- - HCO3- antiporter (Fig. 8). Both mechanisms are active in the proximal tubules (85% of filtered HCO 3-) and TAL (10% of filtered HCO3-). The Cl- - HCO3- antiporter is active at the basolateral membrane of the Intercalated cell located in the late distal tubules and collecting ducts (4.9% of filtered HCO 3-). About 0.1% of filtered HCO3- is excreted. 8. Urinary Buffering i) HCO3- reabsorption does not replenish HCO3- lost during buffering processes in body fluids accompanying metabolism. ii) New HCO3- needs to be produced to maintain acid-base balance. iii) In the collecting duct, tubular fluid contains little HCO3- because most of the HCO3- is reabsorbed in upstream segments of the nephron. The H + secreted into the tubular fluid of the collecting ducts combines with urinary buffer as a consequence. The HCO3- produced from endogenous CO2 involving the activity of carbonic anhydrase does not form part of the filtered HCO 3- and represents newly produced HCO3-. iv) Phosphate buffer (NaHPO4-) (Fig. 9) and ammonia buffer (NH4) (Fig. 10) contained within the tubular fluid, are the main systems involved in the production of new HCO3-. 17 Figure 9: Buffering of tubular fluid in collecting duct by phosphate buffer. Figure 10: Buffering of tubular fluid in the collecting duct by ammonia buffer. Ammonia is produced from glutamine found inside tubular cells involving the action of glutaminase. 9. Waste Removal i) Many cations and anions are removed by the kidney and in most cases they are filtered and/or secreted by tubules. ii) The main site of secretion is the proximal tubule and there are large number of different transporters for organic anions and cations, forming several closely related families of transporters. iii) Cations and anions enter the tubular cells from the blood via an active transporter and then exit into the lumen via an antiporter, which exchanges the cation/anion for something else. Examples of CATIONS excreted via the kidney include; acetylcholine, dopamine, morphine and atropine. Examples of ANIONS excreted via the kidney include; prostaglandins, glucuronate and sulphate conjugates, penicillin and furosemide. iv) Genetic disorders of organic ion transporters lead to renal malfunction, e.g. Bartters Syndrome FURTHER READING Guyton AC, Hall JE. Unit V: The Body Fluids and Kidneys (Chapters 25-31). Guyton & Hall Textbook of Medical Physiology, Twelfth Edition 2011 (International), Saunders Elsevier Philadelphia PA, pg 283-410. 18