Action Experience Drives Visual-Processing Biases Near the Hands PDF
Document Details
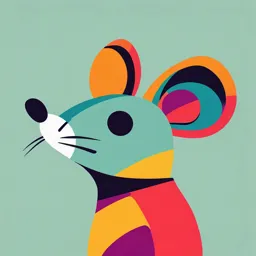
Uploaded by WellEducatedNeodymium
University of Europe for Applied Sciences Berlin
Laura E. Thomas
Tags
Summary
This article investigates the hypothesis that altered vision near the hands is a result of experience-driven plasticity. Participants performed visual tasks with hands near or far, in various postures, before and after learning novel grasping practices. Results showed enhanced visual sensitivity tied to learned grasp methods.
Full Transcript
678189 research-article2016 PSSXXX10.1177/0956797616678189ThomasAction Experience Biases Processing Research Article...
678189 research-article2016 PSSXXX10.1177/0956797616678189ThomasAction Experience Biases Processing Research Article Psychological Science Action Experience Drives Visual-Processing 2017, Vol. 28(1) 124–131 © The Author(s) 2016 Reprints and permissions: Biases Near the Hands sagepub.com/journalsPermissions.nav DOI: 10.1177/0956797616678189 journals.sagepub.com/home/pss Laura E. Thomas Center for Visual and Cognitive Neuroscience, Department of Psychology, North Dakota State University Abstract Observers experience affordance-specific biases in visual processing for objects within the hands’ grasping space, but the mechanism that tunes visual cognition to facilitate action remains unknown. I investigated the hypothesis that altered vision near the hands is a result of experience-driven plasticity. Participants performed motion-detection and form-perception tasks—while their hands were either near the display, in atypical grasping postures, or positioned in their laps—both before and after learning novel grasp affordances. Participants showed enhanced temporal sensitivity for stimuli viewed near the backs of the hands after training to execute a power grasp using the backs of their hands (Experiment 1), but showed enhanced spatial sensitivity for stimuli viewed near the tips of their little fingers after training to use their little fingers to execute a precision grasp (Experiment 2). These results show that visual biases near the hands are plastic, facilitating processing of information relevant to learned grasp affordances. Keywords visual processing, plasticity, action affordances, open data Received 7/15/16; Revision accepted 10/17/16 A decade of research has documented a variety of within reach afford immediate interaction, and by priori- changes in performance on visual cognition tasks when tizing processing of action-relevant information, the observers view information within rather than outside of visual system could give observers an edge in producing their hands’ grasping space. The observed differences effective actions. include alterations in perception (Cosman & Vecera, A recent study supports the idea that action affor- 2010), attention (e.g., Abrams, Davoli, Du, Knapp, & dances have an impact on visual processing (Thomas, Paull, 2008; Reed, Grubb, & Steele, 2006), and memory 2015). Participants in this study performed global-motion- (e.g., Kelly & Brockmole, 2014; Tseng & Bridgeman, detection and global-form-perception tasks designed to 2011; for reviews, see Brockmole, Davoli, Abrams, & measure temporal and spatial sensitivity, respectively, Witt, 2013; Tseng, Bridgeman, & Juan, 2012). Although a while adopting different hand postures. In some condi- popular theoretical account of altered vision near the tions, participants held their hands near the display in a hands posits that information presented in near-hand power-grasp posture—creating an affordance for a fast space receives enhanced processing in the high-tempo- and forceful action using the fingers as a unit (e.g., catch- ral-acuity magnocellular (M) visual pathway at the ing a ball). In other conditions, participants held their expense of the high-spatial-acuity parvocellular (P) path- hands near the display in a manner that afforded a preci- way (e.g., Abrams & Weidler, 2014; Gozli, West, & Pratt, sion grasp—ready to use their thumb and forefinger to 2012; for a review, see Taylor, Gozli, Chan, Huffman, & perform a more delicate and detail-oriented action (e.g., Pratt, 2015), recent work suggests that visual biases near threading a needle). Whereas the power-grasp posture the hands can shift depending on both task demands (Goodhew & Clarke, 2016) and the hands’ positioning Corresponding Author: (Bush & Vecera, 2014; Thomas, 2013). This flexibility in Laura E. Thomas, North Dakota State University, Department of visual biases within the hands’ grasping space may reflect Psychology, NDSU Dept. 2765, P. O. Box 6050, Fargo, ND 58108-6050 an adap tive sensitivity to behavioral contexts. Objects E-mail: [email protected] Action Experience Biases Processing 125 facilitated performance on the motion-detection task, the experience acting with the hands may instead introduce precision-grasp posture instead facilitated performance action-specific shifts in the way people process informa- on the form-perception task. The actions participants tion near their hands. were ready to perform when they viewed a stimulus led To examine this possibility, in two experiments, I to affordance-specific alterations in visual sensitivity: A asked participants to perform a global-motion-detection power-grasp posture biased processing in favor of tem- task and a global-form-perception task under hands-near poral sensitivity to aid fast action, but a precision-grasp and hands-far conditions both before and after a short posture led to a bias toward spatial sensitivity to facilitate training session designed to help them learn a novel detailed-oriented action. grasp affordance. Participants learned to execute a novel Although visual biases near the hands seem to be tied power grasp using the backs of their hands (Experiment to an adaptation that enables more effective action pro- 1) or a novel precision grasp using the tips of their little duction, the driving force behind these biases remains fingers (Experiment 2). Performance in the hands-near unclear. Do short-term learning experiences contribute to and hands-far conditions did not differ before training. altered vision near the hands? Visual biases near the However, following training with the novel grasp affor- hands may be an adaptation specific to the hands’ most dances, positioning the backs of the hands near the dis- common actions—the overlearned power and precision play facilitated motion detection, whereas positioning the grasps that constitute the majority of observers’ interac- tips of the little fingers near the display instead enhanced tions in gripping nearby objects (Napier, 1956). However, form perception. The results of these experiments show the uses to which observers put their hands in the short that action drives visual biases in perihand space and term may also shape the manner in which they process point to the flexibility and adaptability of the visual information near their hands. Is the visual system system in weighting processing on the basis of recent informed not only by the actions people are prepared to experience. take, but also by their recent action experiences? The literature on peripersonal space and vision pro- vides intriguing evidence supporting short-term, experi- Method ence-induced changes in visual processing near used tools (Reed, Betz, Garza, & Roberts, 2010) and the hands Overview of the visual-processing tasks of other actors (Sun & Thomas, 2013). These changes All participants performed both a global-motion-detec- may reflect a temporary incorporation of these external tion and a global-form-perception task. The tasks were objects into an observer’s own body schema (e.g., Iriki, presented with MATLAB and Psychophysics Toolbox Tanaka, & Iwamura, 1996; Soliman, Ferguson, Dexheimer, (Brainard, 1997; Kleiner, Brainard, & Pelli, 2007; Pelli, & Glenberg, 2015). When observers begin to represent 2007) on a 20.1-in. monitor set to a resolution of 1,024 × external objects as extensions of their own hands, this 768 pixels at a refresh rate of 60 Hz. The computer moni- incorporation may induce a fixed set of visual-processing tor rested on a desk face up (see Fig. 1), and participants biases associated with the hands’ most common action looked down at the stimuli. For each task, participants affordances. That is, although representations of peri- viewed arrays of 100 one-pixel black dots presented hand space are subject to action-driven changes, visual- in a 150- × 150-pixel area in the center of a white processing biases associated with the space near the background. hands may be tied to standard power- and precision- In the motion-detection task, participants viewed an grasp affordances. However, I hypothesized that recent eight-frame motion sequence of dots randomly positioned Fig. 1. Participants’ position in (a) the backs-of-hands-near condition, (b) the little-fingers-near condition, and (c) the hands-far condition. 126 Thomas within the central area. Each frame appeared for 50 ms, remaining dots, the noise dots, were placed at random for a total viewing time of 400 ms per trial. On each within the presentation window. Following each presen- frame transition, a portion of the dots, the signal dots, tation, participants verbally indicated whether they shifted 1 pixel in the same direction. This signal direc- perceived a radial or concentric global pattern. An exper- tion—either left or right—was randomly determined on imenter entered these responses with a keyboard. Form- each trial. The remaining portion of the dots, the noise perception thresholds were determined using the same dots, also shifted 1 pixel. The direction of each noise three-down/one-up staircase procedure employed for dot’s motion was randomly determined at the start of the global-motion task. each trial. The locations of the individual dots were ran- domly determined every two frames in order to minimize Experiment 1 local motion cues; this prevented participants from track- ing individual dots across an entire trial. If the pro- Sixty volunteers1 from North Dakota State University’s grammed displacement moved a dot outside of the Psychology Department participant pool participated for presentation window, the dot was repositioned on the course credit. All participants had normal or corrected-to- opposite side of the window for the next frame. normal vision. They performed the global-motion-detec- After participants viewed a motion sequence, they ver- tion and global-form-perception tasks while holding their bally indicated whether they had perceived primary hands in two different postures: In the backs-of-hands- global motion to the left or right. An experimenter sitting near condition, the backs of their hands rested against next to the participants but unable to see the display the sides of the display (see Fig. 1a), and in the hands-far entered these responses using a keyboard. Participants’ condition, their hands remained in their laps (see Fig. global-motion thresholds were determined using a stair- 1c).2 In addition to performing these visual-processing case procedure. A block began with 80% signal dots. The tasks, participants took part in a training session in which percentage of signal dots decreased following three cor- they repeatedly transferred a small plunger between 10 rect responses and increased following a single incorrect possible target locations using the backs of both hands response. Initially, the percentage of signal dots changed (see Fig. 2a). Previous work had shown that tasks involv- by 8%. After three reversals, the percentage of signal dots ing transfer of a small plunger elicit natural power-grasp changed by 4%, and following the fifth reversal, the per- actions (e.g., Rosenbaum, Halloran, & Cohen, 2006), so centage of signal dots increased or decreased by 2%. A performing this task with the backs of the hands taught block of motion-detection trials terminated after eight participants that they could use this atypical posture to reversals. I determined the motion-coherence threshold successfully carry out a power-grasp action goal. An for a block by calculating the mean percentage of signal experimenter sat next to the participants and read from a dots present for the final four reversals in that block. list of randomly generated target locations. After hearing In the form-perception task, participants viewed a a target location, the participants secured the handle of static array of dots positioned within the central presenta- the plunger between the backs of their hands, lifted the tion window for 400 ms. On each trial, pairs of dots were plunger, placed it on the target location, and then let go placed at random locations and oriented to form either a of the plunger. The participants then placed their hands radial or a concentric Glass pattern (Glass, 1969). Whether in their lap, at which time the experimenter read the next these signal dots were arranged into a radial or concen- target location. Participants spent 6 min engaged in this tric pattern was determined randomly across trials. The grasp-training task. Fig. 2. Grasp training for (a) the novel power grasp (Experiment 1) and (b) the novel precision grasp (Experiment 2). Action Experience Biases Processing 127 Participants performed eight blocks of the global- task and hand-posture condition. Figure 3 displays the motion-detection task and eight blocks of the global- means across participants. These scores were submitted form-perception task before grasp training and an to a 2 (time: before vs. after training) × 2 (task: motion additional eight blocks of each of these tasks after grasp detection vs. form perception) × 2 (hand posture: backs training in a counterbalanced order. They adopted the of hands near vs. hands far) analysis of variance (ANOVA). backs-of-hands-near posture for half of the blocks and the This analysis revealed significant main effects of time, F(1, hands-far posture for the other half of the blocks. Written 59) = 8.21, p <.01, ηp2 =.122, and task, F(1, 59) = 17.52, instructions at the beginning of each block indicated p <.01, ηp2 =.229; significant interactions between time which posture participants should adopt, and the experi- and hand posture, F(1, 59) = 4.51, p =.038, ηp2 =.071, and menter collecting responses ensured that participants between task and hand posture, F(1, 59) = 6.78, p =.012, maintained the appropriate posture throughout each ηp2 =.103; and, most crucially, a significant three-way block. The order of postures was randomized across interaction of time, task, and hand posture, F(1, 59) = blocks. The first block for each posture in a task served as 7.13, p =.010, ηp2 =.108 (all other ps >.1). Bonferroni- practice, and data from these blocks were not analyzed. adjusted post hoc pairwise comparisons examined perfor- mance differences between the two hand-posture Experiment 2 conditions separately for the motion and form tasks both before and after training. These tests indicated that perfor- An additional 60 volunteers from the same participant mance differed significantly depending on the hands’ pool participated in Experiment 2 for course credit. All positioning only on the posttraining motion-detection had normal or corrected-to-normal vision, and none had task, t(59) = 3.75, p <.01 (all other adjusted ps >.1). taken part in Experiment 1. The procedure of Experiment To better understand this three-way interaction and 2 was identical to that of Experiment 1 with the following visualize how grasp training changed participants’ per- exceptions: Instead of adopting a hands-near posture in formance, I calculated each participant’s change in which the backs of the hands rested near the display, participants in the second experiment adopted a little- fingers-near posture in which they extended the little fin- ger of each hand and rested the tips of these fingers on Before Training the sides of the display (see Fig. 1b). These participants Backs-of-Hands-Near Hands-Far also trained with a different grasp task, repeatedly using the tips of their little fingers to pluck a small bean from a 55 at Threshold (%) Signal Dots container full of beans and then drop the bean into a cup resting on one of the 10 target locations (see Fig. 2b). 45 This type of task would normally involve using the thumb and forefinger in a typical precision grasp (e.g., Toibana, 35 Ishikawa, & Sakakibara, 2002), so performing this task with the tips of the little fingers taught participants that 25 they could use this atypical posture to successfully carry Motion-Detection Task Form-Perception Task out a precision-grasp action goal. After transferring a bean, participants rested their hands in their laps before After Training the experimenter indicated the next target location. The Backs-of-Hands-Near Hands-Far grasp training lasted 6 min. As in Experiment 1, partici- pants in Experiment 2 performed eight blocks each of 55 at Threshold (%) the motion and form tasks before grasp training and an Signal Dots additional eight blocks of each task following grasp train- 45 ing. Participants performed half of all blocks in the little- fingers-near posture and half in the same hands-far 35 control posture employed in Experiment 1. 25 Motion-Detection Task Form-Perception Task Results Fig. 3. Results from Experiment 1: mean percentage of signal dots Experiment 1 present at the motion-coherence threshold in the motion-detection task and at the form-coherence threshold in the form-perception task. I calculated the mean percentage of signal dots at thresh- The results are shown separately for the two hand-posture conditions old for each participant before and after the novel power- before and after training. Error bars represent ±1 SEM, calculated within grasp training for each combination of visual processing subjects. 128 Thomas Backs-of-Hands-Near Hands-Far.128; the interaction between time and hand posture was marginally significant, F(1, 55) = 3.39, p =.071, ηp2 =.058; 15 and the three-way interaction of time, task, and hand posture was marginally significant, F(1, 55) = 3.45, p = Change in Signal Dots.068, ηp2 =.059 (all other ps >.5). Bonferroni-adjusted After Training (%) 10 post hoc pairwise comparisons between performance in the little-fingers-near and hands-far conditions for both 5 tasks before and after training indicated that the hands’ positioning led to a significant difference in performance 0 only when the form-perception task was performed after training, t(55) = 3.33, p <.01 (all other adjusted ps >.1). Motion-Detection Task Form-Perception Task As in Experiment 1, I calculated each participant’s –5 posttraining change in the percentage of signal dots at Fig. 4. Results from Experiment 1: mean change in the percentage of threshold for each task in each hand-posture condition. signal dots at threshold (pretraining minus posttraining) in the motion- As Figure 6 shows, participants were more sensitive on detection and form-perception tasks. The results are shown separately the form-perception task after training than they were for the two hand-posture conditions. Error bars represent ±1 SEM, cal- culated within subjects. before training when they held their little fingers near the display. Bonferroni-adjusted post hoc one-sample t tests confirmed that, although the change in performance fol- threshold (pretraining threshold minus posttraining lowing training was significantly different from zero in threshold) in each task in each hand-posture condition. the little-fingers-near condition for the form-perception Thus, positive scores indicated greater sensitivity follow- task, t(55) = 5.99, p <.01, participants’ performance did ing training. As Figure 4 shows, although participants’ not improve substantially on this task in the hands-far sensitivity on the motion-detection task improved sub- stantially in the backs-of-hands-near condition following grasp training, training made little difference to perfor- Before Training mance on the motion-detection task in the hands-far Little-Fingers-Near Hands-Far condition or on the form-perception task in either hand- posture condition. Bonferroni-adjusted post hoc one-sample 55 at Threshold (%) t tests confirmed these impressions, indicating that the Signal Dots change in performance was significantly different from 45 zero only in the backs-of-hands-near condition of the motion-detection task, t(59) = 3.94, p <.01 (all other 35 adjusted ps >.1). 25 Experiment 2 Motion-Detection Task Form-Perception Task An experimenter error resulted in the failure to record a After Training complete data set for 2 participants in Experiment 2; Little-Fingers-Near Hands-Far these participants were dropped from analyses. An addi- tional 2 participants were dropped from analyses for fail- 55 at Threshold (%) ing to use their little fingers appropriately during the Signal Dots grasp-training session of the experiment. 45 Figure 5 displays the mean percentage of signal dots at threshold before and after training for each task in 35 each hand-posture condition. As in Experiment 1, these scores were submitted to a 2 (time: before vs. after train- 25 ing) × 2 (task: motion detection vs. form perception) × 2 Motion-Detection Task Form-Perception Task (hand posture: little fingers near vs. hands far) ANOVA. This analysis revealed significant main effects of time, Fig. 5. Results from Experiment 2: mean percentage of signal dots F(1, 55) = 13.19, p <.01, ηp2 =.193; task, F(1, 55) = 11.34, present at the motion-coherence threshold in the motion-detection task and at the form-coherence threshold in the form-perception task. p <.01, ηp2 =.171; and hand posture, F(1, 55) = 4.41, p = The results are shown separately for the two hand-posture conditions.040, ηp2 =.074. In addition, the interaction between time before and after training. Error bars represent ±1 SEM, calculated within and task was significant, F(1, 55) = 8.04, p <.01, ηp2 = subjects. Action Experience Biases Processing 129 Little-Fingers-Near Hands-Far of their hands in a novel power grasp. However, in Experiment 2, participants’ spatial sensitivity near the tips 15 of their little fingers was enhanced after training to use their little fingers in a novel precision grasp. Taken Change in Signal Dots together, these findings provide evidence that recent After Training (%) 10 action experience drives altered vision near the hands, weighting processing in favor of information relevant to 5 newly practiced grasp affordances: Learned power-grasp postures facilitate processing of temporal visual informa- tion that will aid in fast and forceful actions, whereas 0 learned precision-grasp postures lead to increased fine Motion-Detection Task Form-Perception Task spatial sensitivity for more effective detail-oriented –5 actions. This work demonstrates an unprecedented level of cross talk between vision and action, illustrating a Fig. 6. Results from Experiment 2: mean change in the percentage of signal dots at threshold (pretraining minus posttraining) in the motion- remarkable plasticity of the visual-processing system to detection and form-perception tasks. The results are shown separately accommodate action. for the two hand-posture conditions. Error bars represent ±1 SEM, cal- It is important to note that in both experiments and on culated within subjects. both tasks, participants’ performance prior to training was similar regardless of whether their hands were positioned condition or on the motion-detection task in either of the near or far from the display. These results are consistent hand-posture conditions (all other adjusted ps >.1). with previous investigations suggesting that visual biases A comparison of Figures 4 and 6 highlights a striking do not automatically occur near the hands if objects are difference between Experiments 1 and 2 in the influence presented outside of the hands’ typical grasping space of grasp training on performance of the motion-detection (e.g., Reed et al., 2010). Also of importance is the fact that and form-perception tasks. To directly examine this dif- participants showed virtually no improvement in perfor- ference, I submitted participants’ scores for the change in mance following training in the hands-far condition: Sim- signal dots at threshold to a 2 × 2 × 2 mixed ANOVA with ply training with an atypical power or precision grasp did within-subjects factors of task (motion detection vs. form not extend benefits in processing information relevant to perception) and hand posture (hands or fingers near vs. the trained action to regions outside of peripersonal hands far) and a between-subjects factor of experiment space. Instead, participants specifically showed greater (1 vs. 2). This analysis yielded significant main effects of sensitivity after training only when they viewed action- task, F(1, 114) = 4.05, p =.046, ηp2 =.034, and hand pos- relevant information presented near their hands. Together ture, F(1, 114) = 7.52, p <.01, ηp2 =.062; a significant with the absence of a near-hand bias prior to training, interaction between task and experiment, F(1, 114) = these results show that a brief training session essentially 7.48, p <.01, ηp2 =.062; and, most important, a significant created a new zone of grasping space surrounding the three-way interaction of task, hand posture, and experi- hands—space the visual system evaluated in an affor- ment, F(1, 114) = 9.35, p <.01, ηp2 =.076 (all other ps > dance-specific manner. Although previous work has dem-.1). Training with the novel power grasp led to increased onstrated that a short session of practice with a simple temporal sensitivity in Experiment 1, whereas training movement can lead to rapid transient remapping of motor with the novel precision grasp led to increased spatial cortex (e.g., Classen, Liepert, Wise, Hallett, & Cohen, sensitivity in Experiment 2. Grasp training led to changes 1998), and that several days of training with an awkward in how participants processed stimuli presented near grasp using the dominant hand can alter the extent to their hands, but the nature of these changes depended which the grasp is susceptible to visual illusions (Gonza- on the type of grasp on which participants trained. lez, Ganel, Whitwell, Morrissey, & Goodale, 2008), the current research provides the first evidence for short-term motor-driven plasticity in visual processing. Discussion A popular account of altered vision near the hands pos- Across two experiments, I found evidence that training in its that viewing information near hands positioned to using different types of novel grasps led participants to afford a typical power grasp biases processing toward show improvements in sensitivity to different types of increased contribution from the high-temporal-resolution visual information viewed near their hands. In Experi- M pathway at the expense of the high-spatial-resolution P ment 1, participants’ temporal sensitivity near the backs pathway (e.g., Abrams & Weidler, 2014). Although the cur- of their hands was enhanced after training to use this part rent results are not inconsistent with this claim, they also 130 Thomas suggest that visual biases are more malleable than this Notes standard pathways hypothesis suggests. It seems that func- 1. A power analysis based on a moderate effect size (d = 0.4) tion may trump form when it comes to altered vision near derived from previous research (Thomas, 2015) revealed that the hands. When an observer experiences an affordance 55 participants were needed to achieve 95% power. To account for a power grasp—regardless of the hands’ exact pos- for the possibility of missing data or dropped participants, I ran ture—processing may be biased toward the M pathway, an additional 5 participants. For both Experiments 1 and 2, data whereas any affordance for a precision grasp may instead collection stopped after 60 participants were tested. weight processing toward increased contributions from 2. This manipulation potentially introduced a subtle confound: the P pathway. The current results are also potentially con- In the hands-near condition, participants’ hands were posi- tioned somewhat awkwardly, whereas in the hands-far condi- sistent with an attentional account of altered vision near tion, the hands were positioned relatively naturally. However, the hands (e.g., Bush & Vecera, 2014), which suggests that previous research has found that atypical positioning of the power-grasp affordances encourage a broader focus of arms and hands does not drive visual biases near the hands attention consistent with temporal sensitivity whereas pre- (Davoli, Feng, Montana, Garverick, & Abrams, 2010; Weidler & cision-grasp affordances instead create a tighter focus of Abrams, 2013). In addition, data from the pretraining blocks of attention that favors spatial sensitivity. Although future this experiment suggest that this potential difference in comfort work is necessary to delineate the neural underpinnings of between the hands-near and hands-far postures did not lead to visual biases in perihand space, the current results suggest performance differences. that any theory of altered vision near the hands must con- sider action experience and affordances. References Action Editor Abrams, R. A., Davoli, C. C., Du, F., Knapp, W. K., & Paull, D. (2008). Altered vision near the hands. Cognition, 107, Alice J. O’Toole served as action editor for this article. 1035–1047. Abrams, R. A., & Weidler, B. J. (2014). Trade-offs in visual pro- Author Contributions cessing for stimuli near the hands. Attention, Perception, & L. E. Thomas is the sole author of this article and is responsible Psychophysics, 73, 383–390. for its content. Brainard, D. H. (1997). The Psychophysics Toolbox. Spatial Vision, 10, 433–436. Acknowledgments Brockmole, J. R., Davoli, C. C., Abrams, R. A., & Witt, J. K. (2013). The world within reach: Effects of hand posture I thank Hallie Anderson, Katelynn Cox, Alissa Gordon, Morgan and tool use on visual cognition. Current Directions in Jacoby, Maren Lack, Jessica Stelter, and Zainah Haider for their Psychological Science, 22, 38–44. assistance with data collection. Bush, W. S., & Vecera, S. P. (2014). Differential effect of one versus two hands on visual processing. Cognition, 133, Declaration of Conflicting Interests 232–237. The author declared that she had no conflicts of interest with Classen, J., Liepert, J., Wise, S. P., Hallett, M., & Cohen, L. G. respect to her authorship or the publication of this article. (1998). Rapid plasticity of human cortical movement repre- sentation induced by practice. Journal of Neurophysiology, 79, 1117–1123. Funding Cosman, J. D., & Vecera, S. P. (2010). Attention affects visual This material is based on work supported by the National Sci- perceptual processing near the hand. Psychological Science, ence Foundation under Grant BCS 1556336 and by a Google 21, 1254–1258. Faculty Research Award. Davoli, C. C., Feng, D., Montana, J., Garverick, S., & Abrams, R. A. (2010). When meaning matters, look but don’t touch: The effects Open Practices of posture on reading. Memory & Cognition, 38, 555–562. Glass, L. (1969). Moire effect from random dots. Nature, 223, 578–580. Gonzalez, C. L. R., Ganel, T., Whitwell, R. L., Morrissey, B., & All data have been made publicly available via the Open Science Goodale, M. A. (2008). Practice makes perfect, but only Framework and can be accessed at https://osf.io/tqzkd/. The with the right hand: Sensitivity to perceptual illusions with complete Open Practices Disclosure for this article can be found awkward grasps decreases with practice in the right but not at http://pss.sagepub.com/content/by/supplemental-data. This the left hand. Neuropsychologia, 46, 624–631. article has received the badge for Open Data. More information Goodhew, S. C., & Clarke, R. (2016). Contributions of parvocel- about the Open Practices badges can be found at https://osf.io/ lular and magnocellular pathways to visual perception near tvyxz/wiki/1.%20View%20the%20Badges/ and http://pss.sagepub the hands are not fixed, but can be dynamically altered..com/content/25/1/3.full. Psychonomic Bulletin & Review, 23, 156–162. Action Experience Biases Processing 131 Gozli, D. G., West, G. L., & Pratt, J. (2012). Hand position alters with your partner. Journal of Experimental Psychology: vision by biasing processing through different visual path- General, 144, 873–888. ways. Cognition, 124, 244–250. Sun, H.-M., & Thomas, L. E. (2013). Biased attention near anoth- Iriki, A., Tanaka, M., & Iwamura, Y. (1996). Coding of modi- er’s hand following joint action. Frontiers in Psychology, 4, fied body schema during tool use by macaque postcentral Article 443. doi:10.3389/fpsyg.2013.00443 neurons. NeuroReport, 7, 2325–2330. Taylor, J. E. T., Gozli, D. G., Chan, D., Huffman, G., & Pratt, Kelly, S. P., & Brockmole, J. R. (2014). Hand proximity differ- J. (2015). A touchy subject: Advancing the modulated entially affects visual working memory for color and orien- visual pathways account of altered vision near the hand. tation in a binding task. Frontiers in Psychology, 5, Article Translational Neuroscience, 6, 1–7. 318. doi:10.3389/fpsyg.2014.00318 Thomas, L. E. (2013). Grasp posture modulates attentional pri- Kleiner, M., Brainard, D., & Pelli, D. (2007). What’s new in oritization of space near the hands. Frontiers in Psychology, Psychtoolbox-3? [Abstract]. Perception, 36(1 Suppl.), 14. 4, Article 312. doi:10.3389/fpsyg.2013.00312 Napier, J. R. (1956). The prehensile movements of the human Thomas, L. E. (2015). Grasp posture alters visual process- hand. The Journal of Bone and Joint Surgery, 38, 902–913. ing biases near the hands. Psychological Science, 26, Pelli, D. G. (1997). The VideoToolbox software for visual psy- 625–632. chophysics: Transforming numbers into movies. Spatial Toibana, N., Ishikawa, N., & Sakakibara, H. (2002). Measurement Vision, 10, 437–442. of manipulative dexterity in patients with hand-arm vibra- Reed, C. L., Betz, R., Garza, J. P., & Roberts, R. J. (2010). Grab tion syndrome. International Archives of Occupational and it! Biased attention in functional hand and tool space. Environmental Health, 75, 106–110. Attention, Perception, & Psychophysics, 72, 236–245. Tseng, P., & Bridgeman, B. (2011). Improved change detec- Reed, C. L., Grubb, J. D., & Steele, C. (2006). Hands up: Attentional tion with nearby hands. Experimental Brain Research, 209, prioritization of space near the hand. Journal of Experimental 257–269. Psychology: Human Perception and Performance, 32, 166–177. Tseng, P., Bridgeman, B., & Juan, C. H. (2012). Take the matter into Rosenbaum, D. A., Halloran, E. S., & Cohen, R. G. (2006). your own hands: A brief review of the effect of nearby-hands Grasping movement plans. Psychonomic Bulletin & Review, on visual processing. Vision Research, 72, 74–77. 13, 918–922. Weidler, B. J., & Abrams, R. A. (2013). Hand proximity—not Soliman, T. M., Ferguson, R., Dexheimer, M. S., & Glenberg, arm posture—alters vision near the hands. Attention, A. M. (2015). Consequences of joint action: Entanglement Perception, & Psychophysics, 75, 650–653.