Sites Of Drug Action PDF
Document Details
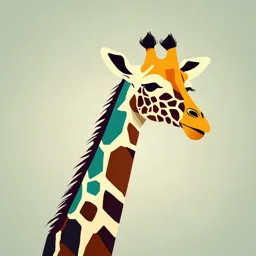
Uploaded by CredibleOcean
Tags
Summary
This document is about psychopharmacology, the study of drug effects on the nervous system. It explores the different meanings of "drug", discussing exogenous chemicals that significantly alter cellular function. It also looks at how drugs affect neurotransmitter production and neurotransmitter activity.
Full Transcript
Several years ago I spent the academic year in a neurological research center affiliated with the teaching hospital at a medical center. One morning as I was having breakfast, I read a brief item in the newspaper about a man who had been hospitalized for botulism. Later that morning, I attended a we...
Several years ago I spent the academic year in a neurological research center affiliated with the teaching hospital at a medical center. One morning as I was having breakfast, I read a brief item in the newspaper about a man who had been hospitalized for botulism. Later that morning, I attended a weekly meeting during which the chief of neurology discussed interesting cases presented by the neurological residents. I was surprised to see that we would visit the man with botulism. We entered the intensive care unit and saw that the man was clearly on his way to recovery. His face was pale and his voice was weak, but he was no longer on a respirator. There wasn’t much to see, so we went back to the lounge and discussed his case. Just before dinner a few days earlier, Mr. F. had opened a jar of asparagus that his family had canned. He noted right away that it smelled funny. Because his family had grown the asparagus in their own garden, he was reluctant to throw it away. However, he decided that he wouldn’t take any chances. He dipped a spoon into the liquid in the jar and touched it to his tongue. It didn’t taste right, so he didn’t swallow it. Instead, he stuck his tongue out and rinsed it under a stream of water from the faucet at the kitchen sink. He dumped the asparagus into the garbage disposal. About an hour later, as the family was finishing dinner, Mr. F. discovered that he was seeing double. Alarmed, he asked his wife to drive him to the hospital. When he C hapter 2 introduced you to the cells of the nervous system, and Chapter 3 described its basic structure. Now it is time to build on this information by introducing the field of psychopharmacology. Psychopharmacology is the study of the effects of drugs on the nervous system and (of course) on behavior. (Pharmakon is the Greek word for “drug.”) But what is a drug? Like many words, this one has several different meanings. In one context it refers to a medication that we would obtain from a pharmacist—a chemical that has a therapeutic effect on a disease or its symptoms. In another context the word refers to a chemical that people are likely to abuse, such as heroin or cocaine. The meaning that will be used in this book (and the one generally accepted by pharmacologists) is “an exogenous chemical not necessary for normal cellular functioning that significantly alters the functions of certain cells of the body when taken in relatively low doses.” Because the topic of this chapter is psychopharmacology, arrived at the emergency room, he was seen by one of the neurological residents, who asked him, “Mr. F., you haven’t eaten some home-canned foods recently, have you?” Learning that he had indeed let some liquid from a suspect jar of asparagus touch his tongue, the resident ordered a vial of botulinum antitoxin from the pharmacy. Meanwhile, he took a blood sample from Mr. F.’s vein and sent it to the lab for some in vivo testing in mice. He then administered the antitoxin to Mr. F., but already he could see that it was too late: The patient was showing obvious signs of muscular weakness and was having some difficulty breathing. He was immediately sent to the intensive care unit, where he was put on a respirator. Although he became completely paralyzed, the life support system did what its name indicates, and he regained control of his muscles. What fascinated me the most was the in vivo testing procedure for the presence of botulinum toxin in Mr. F.’s blood. Plasma extracted from the blood was injected into several mice, half of which had been pretreated with botulinum antitoxin. The pretreated mice survived; the others died. Just think: Mr. F. had touched only a few drops of the contaminated liquid on his tongue and then rinsed it off immediately, but enough of the toxin entered his bloodstream that a small amount of his blood plasma could kill a mouse. By the way, we will examine the pharmacological effect of botulinum toxin later in this chapter. we will concern ourselves here only with chemicals that alter the functions of cells within the nervous system. The word exogenous rules out chemical messengers produced by the body, such as neurotransmitters, neuromodulators, or hormones. (Exogenous means “produced from without”—that is, from outside the body.) Chemical messengers produced by the body are not drugs, although synthetic chemicals that mimic their effects are classified as drugs. The definition of a drug also rules out essential nutrients, such as proteins, fats, carbohydrates, minerals, and vitamins that are a necessary constituent of a healthy diet. Finally, it states that drugs are effective in low doses. This qualification is important, because large quantities of almost any substance—even common ones such as table salt—will alter the functions of cells. x psychopharmacology The study of the effects of drugs on the nervous system and on behavior. 106 Chapter 4 Psychopharmacology SECTION SUMMARY Principles of Psychopharmacology Psychopharmacology is the study of the effects of drugs on the nervous system and behavior. Drugs are exogenous chemicals that are not necessary for normal cellular functioning that significantly alter the functions of certain cells of the body when taken in relatively low doses. Drugs have effects, physiological and behavioral, and they have sites of action—molecules located somewhere in that body with which they interact to produce these effects. Pharmacokinetics is the fate of a drug as it is absorbed into the body, circulates throughout the body, and reaches its sites of action. Drugs may be administered by intravenous, intraperitoneal, intramuscular, and subcutaneous injection; they may be administered orally, sublingually, intrarectally, by inhalation, and topically (on skin or mucous membrane); and they may be injected intracerebrally or intracerebroventricularly. Lipid-soluble drugs easily pass through the blood–brain barrier, whereas others pass this barrier slowly or not at all. The time courses of various routes of drug administration are different. Eventually, drugs disappear from the body. Some are deactivated by enzymes, especially in the liver, and others are simply excreted. The dose-response curve represents a drug’s effectiveness; it relates the amount administered Sites of Drug Action Throughout the history of our species, people have discovered that plants—and some animals—produce chemicals that act on the nervous system. (Of course, the people who discovered these chemicals knew nothing about neurons and synapses.) Some of these chemicals have been used for their pleasurable effects; others have been used to treat illness, reduce pain, or poison other animals (or enemies). More recently, scientists have learned to produce completely artificial drugs, some with potencies far greater than those of the naturally occurring drugs. The traditional uses of drugs remain, but in addition they can be used in research (usually in milligrams per kilogram of the subject’s body weight) to the resulting effect. Most drugs have more than one site of action and thus more than one effect. The safety of a drug is measured by the difference between doses that produce desirable effects and those that produce toxic side effects. Drugs vary in their effectiveness because of the nature of their sites of actions and the affinity between molecules of the drug and these sites of action. Repeated administration of a drug can cause either tolerance, often resulting in withdrawal symptoms, or sensitization. Tolerance can be caused by decreased affinity of a drug with its receptors, by decreased numbers of receptors, or by decreased coupling of receptors with the biochemical steps it controls. Some of the effects of a drug may show tolerance, while others may not—or may even show sensitization. THOUGHT QUESTIONS 1. Choose a drug whose effects you are familiar with and suggest where in the body the sites of action of that drug might be. 2. Some drugs can cause liver damage if large doses are taken for an extended period of time. What aspect of the pharmacokinetics of these drugs might cause the liver damage? j laboratories to investigate the operations of the nervous system. Most drugs that affect behavior do so by affecting synaptic transmission. Drugs that affect synaptic transmission are classified into two general categories. Those that block or inhibit the postsynaptic effects are called antagonists. Those that facilitate them are called agonists. (The Greek word agon means “contest.” Thus, an agonist is one who takes part in the contest.) x antagonist A drug that opposes or inhibits the effects of a particular neurotransmitter on the postsynaptic cell. x agonist A drug that facilitates the effects of a particular neurotransmitter on the postsynaptic cell. Sites of Drug Action This section will describe the basic effects of drugs on synaptic activity. Recall from Chapter 2 that the sequence of synaptic activity goes like this: Neurotransmitters are synthesized and stored in synaptic vesicles. The synaptic vesicles travel to the presynaptic membrane, where they become docked. When an axon fires, voltage-dependent calcium channels in the presynaptic membrane open, permitting the entry of calcium ions. The calcium ions interact with the docking proteins and initiate the release of the neurotransmitters into the synaptic cleft. Molecules of the neurotransmitter bind with postsynaptic receptors, causing particular ion channels to open, which produces excitatory or inhibitory postsynaptic potentials. The effects of the neurotransmitter are kept relatively brief by their reuptake by transporter molecules in the presynaptic membrane or by their destruction by enzymes. In addition, the stimulation of presynaptic autoreceptors on the terminal buttons regulates the synthesis and release of the neurotransmitter. The discussion of the effects of drugs in this section follows the same basic sequence. All of the 107 effects I will describe are summarized in Figure 4.4, with some details shown in additional figures. I should warn you that some of the effects are complex, so the discussion that follows bears careful reading. I recommend that you Simulate actions of drugs on MyPsychLab, which reviews this material. Effects on Production of Neurotransmitters The first step is the synthesis of the neurotransmitter from its precursors. In some cases the rate of synthesis and release of a neurotransmitter is increased when a View precursor is administered; in these cases the precursor itself serves as an agonist. (See step 1 in Figure 4.4.) Watch The steps in the synthesis of neurotransmitters are controlled by enzymes. Therefore, if a drug inactivates Listen one of these enzymes, it will prevent the neurotransmitter from being produced. Such a drug serves as an Explore antagonist. (See step 2 in Figure 4.4.) Simulate Study and Review Drug serves as precursor AGO (e.g., L-DOPA—dopamine) 1 2 Read Precursor Map 3 4 Drug prevents storage of NT in vesicles ANT (e.g., reserpine—monoamines) Drug inactivates synthetic enzyme; inhibits synthesis of NT ANT (e.g., PCPA—serotonin) Enzyme 8 Drug stimulates autoreceptors; inhibits synthesis/release of NT ANT (e.g., apomorphine—dopamine) Neurotransmitter Drug stimulates release of NT AGO (e.g., black widow spider venom—ACh) 9 5 6 Inhibition Drug inhibits release of NT ANT (e.g., botulinum toxin—ACh) 10 Drug stimulates postsynaptic receptors AGO (e.g., nicotine, muscarine—ACh) Choline + acetate ACh 7 Drug blocks postsynaptic receptors ANT (e.g., curare, atropine—ACh) FIGURE Drug blocks autoreceptors; increases synthesis/release of NT AGO (e.g., idazoxan—norepinephrine) Molecules of drugs AChE 11 Drug blocks reuptake AGO (e.g., cocaine—dopamine) Drug inactivates acetylcholinesterase AGO (e.g., physostigmine—ACh) 4.4 Drug Effects on Synaptic Transmission The figure summarizes the ways in which drugs can affect the synaptic transmission (AGO = agonist; ANT = antagonist; NT = neurotransmitter). Drugs that act as agonists POB,11e/C11B04F04.eps Carlson/ are marked in blue; drugs that act as antagonists are marked in red. 42.0 x 24.8 108 Chapter 4 Psychopharmacology Effects on Storage and Release of Neurotransmitters Neurotransmitters are stored in synaptic vesicles, which are transported to the presynaptic membrane, where the chemicals are released. The storage of neurotransmitters in vesicles is accomplished by the same kind of transporter molecules that are responsible for reuptake of a neurotransmitter into a terminal button. The transporter molecules are located in the membrane of synaptic vesicles, and their action is to pump molecules of the neurotransmitter across the membrane, filling the vesicles. Some of the transporter molecules that fill synaptic vesicles are capable of being blocked by a drug. Molecules of the drug bind with a particular site on the transporter and inactivate it. Because the synaptic vesicles remain empty, nothing is released when the vesicles eventually rupture against the presynaptic membrane. The drug serves as an antagonist. (See step 3 in Figure 4.4.) Some drugs act as antagonists by preventing the release of neurotransmitters from the terminal button. They do so by deactivating the proteins that cause docked synaptic vesicles to fuse with the presynaptic membrane and expel their contents into the synaptic cleft. Other drugs have just the opposite effect: They act as agonists by binding with these proteins and directly triggering release of the neurotransmitter. (See steps 4 and 5 in Figure 4.4.) Effects on Receptors The most important—and most complex—site of action of drugs in the nervous system is on receptors, both Drug Neurotransmitter binding site Drug presynaptic and postsynaptic. Let’s consider postsynaptic receptors first. (Here is where the careful reading should begin.) Once a neurotransmitter is released, it must stimulate the postsynaptic receptors. Some drugs bind with these receptors, just as the neurotransmitter does. Once a drug has bound with the receptor, it can serve as either an agonist or an antagonist. A drug that mimics the effects of a neurotransmitter acts as a direct agonist. Molecules of the drug attach to the binding site to which the neurotransmitter normally attaches. This binding causes ion channels controlled by the receptor to open, just as they do when the neurotransmitter is present. Ions then pass through these channels and produce postsynaptic potentials. (See step 6 in Figure 4.4.) Drugs that bind with postsynaptic receptors can also serve as antagonists. Molecules of such drugs bind with the receptors but do not open the ion channel. Because they occupy the receptor’s binding site, they prevent the neurotransmitter from opening the ion channel. These drugs are called receptor blockers or direct antagonists. (See step 7 in Figure 4.4.) Some receptors have multiple binding sites, to which different ligands can attach. Molecules of the neurotransmitter bind with one site, and other substances (such as neuromodulators and various drugs) bind with the others. Binding of a molecule with one of these alternative sites is referred to as noncompetitive binding, because the molecule does not compete with molecules of the neurotransmitter for the same binding site. If a drug attaches to one of these alternative sites and prevents the ion channel from opening, the drug is said to be an indirect antagonist. The ultimate effect of an indirect antagonist is similar to that of a direct antagonist, but its Neurotransmitter Drug Neurotransmitter Drug Competitive Binding Direct agonist Direct antagonist (a) FIGURE Neuromodulator binding site Noncompetitive Binding Indirect agonist Indirect antagonist (b) 4.5 Drug Actions at Binding Sites (a) Competitive binding: Direct agonists and antagonists act directly on the Carlson/ POB,11e/C11B04F05.eps neurotransmitter binding site. (b) Noncompetitive binding: Indirect agonists and x 12.3 39.8 antagonists act on an alternative binding site and modify the effects of the neurotransmitter on opening of the ion channel. Sites of Drug Action Molecule of drug that activates presynaptic heteroreceptors Presynaptic terminal button Presynaptic heteroreceptor Presynaptic inhibition: Opening of calcium channels is inhibited Synaptic vesicle Postsynaptic terminal button Calcium channels must open to permit the release of the neurotransmitter FIGURE Presynaptic facilitation: Opening of calcium channels is facilitated Postsynaptic receptor 4.6 Presynaptic Heteroreceptors Presynaptic facilitation is caused by activation of Carlson/ POB,11e/C11B04F06.eps receptors that facilitate opening of calcium 20.0 the x 28.0 channels near the active zone of the postsynaptic terminal button, which promotes release of the neurotransmitter. Presynaptic inhibition is caused by activation of receptors that inhibit the opening of these calcium channels. site of action is different. If a drug attaches to one of the alternative sites and facilitates the opening of the ion channel, it is said to be an indirect agonist. (See Figure 4.5.) As we saw in Chapter 2, the presynaptic membranes of some neurons contain autoreceptors that regulate the amount of neurotransmitter that is released. Because stimulation of these receptors causes less neurotransmitter to be released, drugs that selectively activate presynaptic receptors act as antagonists. Drugs that block presynaptic autoreceptors have the opposite effect: They increase the release of the neurotransmitter, acting as agonists. (Refer to steps 8 and 9 in Figure 4.4.) We also saw in Chapter 2 that some terminal buttons form axoaxonic synapses—synapses of one terminal 109 button with another. Activation of the first terminal button causes presynaptic inhibition or facilitation of the second one. The second terminal button contains presynaptic heteroreceptors, which are sensitive to the neurotransmitter released by the first one. (Auto means “self”; hetero means “other.”) Presynaptic heteroreceptors that produce presynaptic inhibition do so by inhibiting the release of the neurotransmitter. Conversely, presynaptic heteroreceptors responsible for presynaptic facilitation facilitate the release of the neurotransmitter. So drugs can block or facilitate presynaptic inhibition or facilitation, depending on whether they block or activate presynaptic heteroreceptors. (See Figure 4.6.) Finally (yes, this is the last site of action I will describe in this subsection), you will recall from Chapter 2 that autoreceptors are located in the membrane of dendrites of some neurons. When these neurons become active, their dendrites, as well as their terminal buttons, release neurotransmitter. The neurotransmitter released by the dendrites stimulates autoreceptors located on these same dendrites, which decrease neural firing by producing hyperpolarizations. This mechanism has a regulatory effect, serving to prevent these neurons from becoming too active. Thus, drugs that bind with and activate dendritic autoreceptors will serve as antagonists. Those that bind with and block dendritic autoreceptors will serve as agonists, because they will prevent the inhibitory hyperpolarizations. (See Figure 4.7.) As you will surely realize, the effects of a particular drug that binds with a particular type of receptor can be very complex. The effects depend on where the receptor is located, what its normal effects are, and whether the drug activates the receptor or blocks its actions. x direct agonist A drug that binds with and activates a receptor. x receptor blocker A drug that binds with a receptor but does not activate it; prevents the natural ligand from binding with the receptor. x direct antagonist A synonym for receptor blocker. x noncompetitive binding Binding of a drug to a site on a receptor; does not interfere with the binding site for the principal ligand. x indirect antagonist A drug that attaches to a binding site on a receptor and interferes with the action of the receptor; does not interfere with the binding site for the principal ligand. x indirect agonist A drug that attaches to a binding site on a receptor and facilitates the action of the receptor; does not interfere with the binding site for the principal ligand. x presynaptic heteroreceptor A receptor located in the membrane of a terminal button that receives input from another terminal button by means of an axoaxonic synapse; binds with the neurotransmitter released by the presynaptic terminal button. 110 Chapter 4 Psychopharmacology Activation of dendritic autoreceptors produces a hyperpolarization Molecule of neurotransmitter released by dendrite when neuron becomes active Dendritic autoreceptor Cell body Nucleus FIGURE 4.7 Dendritic Autoreceptors Carlson/ POB,11e/C11B04F07.eps The dendrites of certain neurons release some 20.0 the x 16.2 neurotransmitter when cell is active. Activation of dendritic autoreceptors by the neurotransmitter (or by a drug that binds with these receptors) hyperpolarizes the membrane, reducing the neuron’s rate of firing. Blocking of dendritic autoreceptors by a drug prevents this effect. Effects on Reuptake or Destruction of Neurotransmitters The next step after stimulation of the postsynaptic receptor is termination of the postsynaptic potential. Two processes accomplish that task: Molecules of the neurotransmitter are taken back into the terminal button through the process of reuptake, or they are destroyed by an enzyme. Drugs can interfere with either of these processes. In the first case, molecules of the drug attach to the transporter molecules responsible for reuptake and inactivate them, thus blocking reuptake. In the second case, molecules of the drug bind with the enzyme that normally destroys the neurotransmitter and prevents the enzymes from working. The most important example of such an enzyme is acetylcholinesterase, which destroys acetylcholine. Because both types of drugs prolong the presence of molecules of the neurotransmitter in the synaptic cleft (and hence in a location where these molecules can stimulate postsynaptic receptors), they serve as agonists. (Refer to steps 10 and 11 in Figure 4.4.) SECTION SUMMARY Sites of Drug Action The process of synaptic transmission entails the synthesis of the neurotransmitter, its storage in synaptic vesicles, its release into the synaptic cleft, its interaction with postsynaptic receptors, and the consequent opening of ion channels in the postsynaptic membrane. The effects of the neurotransmitter are then terminated by reuptake into the terminal button or by enzymatic deactivation. Each of the steps necessary for synaptic transmission can be interfered with by drugs that serve as antagonists, and a few can be stimulated by drugs that serve as agonists. Thus, drugs can increase the pool of available precursor, block a biosynthetic enzyme, prevent the storage of neurotransmitter in synaptic vesicles, stimulate or block the release of the neurotransmitter, stimulate or block presynaptic or postsynaptic receptors, retard reuptake, or deactivate enzymes that destroy the neurotransmitter. A drug that activates postsynaptic receptors serves as an agonist, whereas one that activates presynaptic or dendritic autoreceptors serves as an antagonist. A drug that blocks postsynaptic receptors serves as an antagonist, whereas one that blocks autoreceptors serves as an agonist. A drug that activates or blocks presynaptic heteroreceptors serves as an agonist or antagonist, depending on whether the heteroreceptors are responsible for presynaptic facilitation or inhibition. THOUGHT QUESTION Explain how a drug that blocks receptors can serve as an agonist. j