Principles of Flight PDF
Document Details
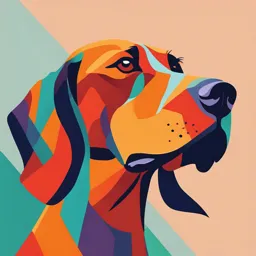
Uploaded by [email protected]
Tags
Summary
This document explains the principles of flight, focusing on the fundamental forces involved in aviation, such as thrust, lift, weight, and drag. It covers Newton's Third Law and Bernoulli's principle, using illustrative examples to clarify these concepts.
Full Transcript
Principles of Flight In the eld of aviation, the principles of physics play a crucial role in understanding and explaining the various phenomena that occur during ight. Two fundamental principles that have signi cant implications in aviation are Newton's Third Law of Motion and Bernoulli's Principle...
Principles of Flight In the eld of aviation, the principles of physics play a crucial role in understanding and explaining the various phenomena that occur during ight. Two fundamental principles that have signi cant implications in aviation are Newton's Third Law of Motion and Bernoulli's Principle. These principles provide a solid foundation for comprehending the forces acting upon aircraft and the factors that contribute to their ability to y. Newton’s Third Law of Motion Explanation of Newton’s Third Law Newton’s Third Law of Motion, often referred to as the law of action and reaction, states that for every action, there is an equal and opposite reaction. This means that when an object exerts a force on another object, the second object exerts an equal and opposite force back on the rst object. The forces, although equal in magnitude, act in opposite directions. Application in Aviation In aviation, Newton’s Third Law of Motion plays a fundamental role in the principles governing ight. To understand how this law applies, we must look at the forces acting on an aircraft during ight. During takeo , the engines of an aircraft generate thrust by expelling a large volume of air backward. According to Newton’s Third Law, as the engines push the air backward, an equal and opposite force is exerted on the aircraft, propelling it forward. This force of thrust allows the aircraft to overcome drag and accelerate down the runway. Similarly, during the ight, the wings of an aircraft generate lift, which is the upward force that counteracts gravity. As the aircraft moves forward, air ows over the wings, creating a di erence in air pressure. According to Newton’s Third Law, for every action of air pushing down on the wings (higher pressure), there is an equal and opposite reaction of the wings pushing up on the air (lower pressure). This reaction force generates lift, allowing the aircraft to stay airborne. Examples of Newton’s Third Law in Aviation One example that demonstrates Newton’s Third Law in aviation is the operation of a jet engine. As the engine's exhaust gases are expelled backward with tremendous force, an equal and opposite force is exerted on the engine itself, propelling the aircraft forward. Another example is the steering of an aircraft. To change direction, the pilot adjusts the ailerons, elevators, and rudder. When the ailerons are used to bank the plane, the downward force exerted by the wing generates an equal and opposite force that pushes the aircraft in the opposite direction, causing it to turn. In summary, Newton’s Third Law of Motion is a fundamental principle in aviation that governs the forces involved in ight. From the generation of thrust to the production of lift, this law explains how aircraft can overcome the forces of gravity and move through the air with precision and control. Bernoulli’s Principle Explanation of Bernoulli’s Principle Bernoulli’s Principle is a fundamental concept in uid dynamics that explains the relationship between the speed of a uid and its pressure. It states that as the speed of a uid increases, its pressure decreases, and vice versa. This principle is based on the law of conservation of energy, which states that energy cannot be created or destroyed but only transformed from one form to another. According to Bernoulli’s Principle, when a uid ows through a constriction, such as a pipe or a wing, the uid accelerates, which leads to a decrease in pressure perpendicular to the ow direction. This decrease in pressure creates a force, known as the Bernoulli force, which acts perpendicular to the direction of the uid ow. Application in Aviation In aviation, Bernoulli’s Principle plays a crucial role in the lift generation of aircraft. The wings of an aircraft are designed with a speci c shape called an airfoil, which is curved on the top and relatively at on the bottom. As the aircraft moves forward, air ows over and under the wings. The shape of the airfoil causes the air to travel faster over the curved top surface than the bottom surface. According to Bernoulli’s Principle, the faster-moving air over the top surface creates a region of lower pressure compared to the slowermoving air under the bottom surface. This pressure di erence creates lift, which is the force that opposes gravity and allows an aircraft to stay airborne. Examples of Bernoulli’s Principle in Aviation 1. Takeo and Landing: During takeo , pilots increase the speed of the aircraft, which results in a decrease in air pressure above the wings. This decrease in pressure generates lift, allowing the aircraft to become airborne. Similarly, during landing, pilots decrease the speed of the aircraft, which increases the air pressure above the wings, helping to slow down the aircraft. 2. Wing Design: Aircraft wings are carefully designed to maximize the e ects of Bernoulli’s Principle. The shape, size, and curvature of the wings are optimized to ensure optimal lift generation and minimize drag. This design allows for e cient and stable ight. 3. Aerobatics: The principles of Bernoulli’s Principle are also evident in aerobatic maneuvers performed by skilled pilots. By manipulating the air ow and pressure distribution over the wings, pilots can perform maneuvers such as loops, rolls, and spins. Conclusion In conclusion, Newton’s third law of motion and Bernoulli’s principle play crucial roles in aviation, shaping the way aircraft are designed and controlled. Newton’s third law, often referred to as the law of action and reaction, states that for every action, there is an equal and opposite reaction. This law is fundamental to understanding the forces acting on an aircraft during ight. By applying Newton’s third law, aviators and engineers are able to design aircraft that can generate lift, counteract gravity, and maneuver through the air. The principle of equal and opposite reactions allows aircraft to generate lift by creating a pressure di erence between the upper and lower surfaces of the wings. This lift force enables airplanes to take o , stay aloft, and safely land. Furthermore, Bernoulli’s principle, named after the Swiss mathematician Daniel Bernoulli, explains the relationship between the speed of a uid and its pressure. This principle is an essential concept in aviation, as it helps explain the phenomenon of lift. According to Bernoulli’s principle, as the speed of a uid (in this case, air) increases, its pressure decreases. In aviation, Bernoulli’s principle is applied to the design of wings and airfoils. By shaping the wings in a speci c manner, engineers can create a pressure di erence between the upper and lower surfaces of the wings, resulting in lift. This lift force allows aircraft to overcome the force of gravity and achieve ight. Both Newton’s third law of motion and Bernoulli’s principle work in harmony to enable safe and e cient aviation. From the moment an aircraft takes o to the second it lands, these principles are at play, ensuring the aircraft's stability, maneuverability, and the safety of all those on board. In summary, Newton’s third law of motion and Bernoulli’s principle are integral to the science of aviation. They allow us to understand the forces acting upon an aircraft and enable engineers to design aircraft that can y. By harnessing these principles, aviators continue to push the boundaries of ight, making air travel faster, more e cient, and safer than ever before. Forces A ecting Flight Flight, the ability to soar through the skies, has captivated human imagination for centuries. From the mythical tales of Icarus to the groundbreaking achievements of the Wright brothers, the concept of defying gravity has always intrigued us. But what exactly makes ight possible? What are the fundamental forces that come into play when an object takes to the air? In this section, we will explore the forces that a ect ight, namely thrust, lift, weight, and drag. Each of these forces plays a crucial role in determining how an aircraft behaves in the air. By understanding these forces and their underlying principles, we can gain a deeper appreciation for the marvel of ight. Thrust Thrust is a key component of ight that propels an aircraft forward. It is the force generated by the propulsion system, such as a jet engine or a propeller, that counteracts the opposing forces of drag and allows an aircraft to move through the air. Without thrust, an aircraft would simply remain stationary in the air. Thrust is commonly measured in units of force, such as pounds or newtons. It is responsible for overcoming the drag force, which is caused by the resistance of the air against the forward motion of the aircraft, as well as other factors such as weight and lift. Types of Thrust There are several types of thrust used in aviation, each with its own unique characteristics and applications. 1. Jet Thrust: Jet engines, commonly used in commercial and military aircraft, produce jet thrust. This type of thrust is generated by the expulsion of high-velocity gases from the engine, creating a reaction force in the opposite direction. Jet thrust is highly e cient and provides signi cant power for propulsion. 2. Propeller Thrust: Propeller-driven aircraft rely on propeller thrust, which is generated by the rotation of the propeller blades. As the blades spin, they create a pressure di erence between the front and back surfaces. This pressure di erence generates a forward force that propels the aircraft. 3. Rocket Thrust: Rocket engines generate thrust by expelling high-velocity gases produced by the combustion of propellants. Unlike jet engines, rocket engines do not require atmospheric oxygen for combustion, making them suitable for space travel and high-altitude ights. Factors A ecting Thrust Several factors in uence the amount of thrust generated by an aircraft's propulsion system. These factors can impact an aircraft's performance, speed, and ability to climb or maintain altitude. 1. Engine Power: The power output of an engine directly a ects the amount of thrust it can generate. Engines with higher power outputs can produce greater thrust, allowing for faster speeds and improved performance. 2. Air Density: The density of the air surrounding an aircraft a ects thrust production. As air density decreases with altitude, the engine's e ciency in generating thrust is also reduced. This is why aircraft often struggle to climb and maintain performance at higher altitudes. 3. Temperature: The temperature of the air entering the engine can impact thrust. Warmer air is less dense than cooler air, resulting in reduced engine e ciency and lower thrust production. 4. Altitude: The altitude at which an aircraft operates a ects thrust production. As altitude increases, the air pressure decreases, reducing the amount of air available for the engine to generate thrust. This can result in diminished engine performance and reduced thrust. Understanding the concept of thrust and the factors that in uence it is essential for comprehending the principles of ight. By harnessing and controlling this force, pilots and engineers can optimize the performance and e ciency of aircraft, allowing for safe and successful journeys through the skies. Lift Lift is the force that allows an aircraft to overcome gravity and stay in the air. It is the upward force generated by the interaction between the wings and the air owing over them. Without lift, an aircraft would not be able to sustain ight. To understand lift, it is important to grasp a concept known as Bernoulli's principle. According to this principle, as the speed of a uid (in this case, air) increases, its pressure decreases. In the context of aviation, this principle explains how air moves over and under an aircraft wing to generate lift. Principles of Lift The shape of an aircraft wing, known as an airfoil, plays a crucial role in generating lift. The airfoil's shape, with a curved upper surface and a atter lower surface, creates di erent air pressures above and below the wing. This pressure di erence results in an upward force called lift. When air ows over the curved upper surface of the wing, it has to travel a longer distance compared to the air owing beneath the wing. As a result, the air above the wing has to move faster, in accordance with Bernoulli's principle, to catch up with the air below. This increase in air speed over the wing's surface leads to a decrease in pressure, creating a low-pressure zone above the wing. Conversely, the air owing beneath the wing moves at a slower speed, creating a higher pressure zone. This pressure di erence between the upper and lower surfaces of the wing generates lift, pulling the aircraft upward. Factors A ecting Lift Several factors in uence the amount of lift generated by an aircraft wing. These include: 1. Angle of Attack : The angle at which the wing meets the oncoming air ow determines the amount of lift generated. Increasing the angle of attack increases lift up to a certain point, beyond which the air ow becomes turbulent and lift decreases. 2. Airfoil Shape: The shape and design of the wing's airfoil greatly impact lift generation. Di erent airfoils are optimized for speci c ight characteristics, such as high lift for takeo and landing, or low drag for cruising. 3. Wing Area: The size of the wing's surface area a ects the amount of lift produced. A larger wing area creates more lift, allowing for heavier aircraft or slower ight speeds. 4. Airspeed: The speed at which an aircraft is ying signi cantly in uences lift. As airspeed increases, the amount of lift generated also increases. However, there is a critical speed beyond which lift decreases due to the onset of drag. Understanding the principles and factors that a ect lift is vital for pilots and aircraft designers. By manipulating these variables, pilots can control altitude, climb, and descent, while designers can optimize aircraft performance for speci c missions. In the next section, we will explore the concept of weight and its impact on ight. Understanding the relationship between lift and weight is crucial for maintaining stability and control in the air. Weight Weight, in the context of ight, refers to the force exerted on an aircraft due to the gravitational pull of the Earth. It is the result of the mass of the aircraft and all its contents, including passengers, cargo, and fuel. Weight is a crucial factor in ight as it directly a ects the performance and stability of an aircraft. E ects of Weight on Flight The weight of an aircraft has a signi cant impact on its ability to take o , climb, cruise, and land. The primary e ects of weight on ight include: 1. Lift Requirement: To achieve and maintain level ight, an aircraft must generate enough lift to counteract its weight. The greater the weight of an aircraft, the more lift it needs to generate to stay airborne. This relationship between weight and lift is crucial for maintaining the desired altitude during ight. 2. Takeo and Landing Distance: The weight of an aircraft a ects the distance required for takeo and landing. Heavier aircraft need a longer runway for takeo because they require a higher speed to generate enough lift. Similarly, during landing, a heavier aircraft needs a longer runway to safely decelerate and come to a stop. 3. Rate of Climb: The weight of an aircraft also a ects its rate of climb. A heavier aircraft requires a higher amount of thrust to overcome the gravitational pull and ascend. Consequently, the rate at which it can climb will be slower compared to a lighter aircraft. Weight Distribution and Balance In addition to the overall weight of an aircraft, how that weight is distributed plays a crucial role in ight safety and stability. Improper weight distribution can lead to handling di culties and a ect the aircraft's performance. To ensure proper weight distribution and balance, aircraft manufacturers provide guidelines on how to load cargo, passengers, and fuel. 1. Center of Gravity: The center of gravity (CG) is the point at which an aircraft balances horizontally. It represents the average location of the weight of the aircraft. Maintaining the correct CG position is vital for maintaining stability during ight. If the CG is too far forward or too far aft, it can result in poor control, reduced maneuverability, and potentially even loss of control. 2. Weight and Balance Calculations: Pilots and ground crews must perform weight and balance calculations before every ight. These calculations ensure that the aircraft remains within its speci ed weight and balance limits. By distributing the weight properly, pilots can optimize the aircraft's performance and stability, enhancing safety during ight. Understanding the role of weight in ight is crucial for pilots, engineers, and anyone involved in the aviation industry. By considering weight distribution, balance, and the e ects of weight on performance, aircraft can be operated safely and e ciently. Drag Drag is a force that opposes the forward motion of an object through a uid, such as air. In the context of ight, drag refers to the resistance encountered by an aircraft as it moves through the atmosphere. It is an essential force to understand as it plays a crucial role in determining an aircraft's performance and e ciency. Types of Drag There are primarily four types of drag that a ect the ight of an aircraft: 1. Parasitic Drag: This type of drag is caused by the non-lifting parts of an aircraft, such as the fuselage, wings, landing gear, and any protrusions like antennas or rivets. Parasitic drag can further be divided into two components: form drag and skin friction drag. Form drag is the resistance created by the shape of an aircraft, while skin friction drag is the frictional resistance between the aircraft's surface and the surrounding air. 2. Induced Drag: Induced drag is generated by the production of lift. As an aircraft generates lift, it also creates vortices at the wingtips, which result in a backward force known as induced drag. This type of drag increases as the aircraft's angle of attack increases or as the aircraft slows down. 3. Interference Drag: Interference drag occurs when two or more components of an aircraft interact, causing turbulence and additional resistance. It typically arises at junctions between di erent sections of the aircraft, such as the wing-fuselage junction or the wing-wing junction. 4. Wave Drag: Wave drag is associated with supersonic or transonic ight. As an aircraft approaches or exceeds the speed of sound, shock waves form around the aircraft, creating a signi cant increase in drag. This type of drag can be reduced through careful design considerations, such as using streamlined shapes and minimizing sharp edges. Minimizing Drag Minimizing drag is crucial for improving an aircraft's performance and fuel e ciency. Several techniques are employed to reduce drag: 1. Streamlining: By designing aircraft with smooth and streamlined shapes, engineers can reduce form drag. Streamlining involves minimizing areas of high pressure and ensuring smooth air ow over the aircraft's surfaces. 2. Wing Design: The shape and aspect ratio of an aircraft's wings play a signi cant role in reducing drag. High aspect ratio wings, which are long and narrow, create less induced drag than low aspect ratio wings. 3. Winglets: Winglets are vertical extensions at the wingtips that help reduce induced drag by minimizing the vortices formed at the wingtips. They e ectively decrease the lift-induced drag and improve an aircraft's fuel e ciency. 4. Surface Treatments: The use of special coatings or materials on an aircraft's surface can reduce skin friction drag by reducing the friction between the aircraft's surface and the air. 5. Proper Aircraft Maintenance: Regular maintenance ensures that an aircraft's surfaces remain clean and free from debris, which can increase drag. Minimizing drag is a constant priority for aircraft designers and operators, as any reduction in drag can lead to improved speed, range, and fuel e ciency. Conclusion In conclusion, understanding and managing drag is essential for optimizing the ight performance of an aircraft. By reducing drag through streamlining, wing design, and other techniques, aircraft designers can enhance an aircraft's e ciency and overall performance. Minimizing drag not only improves an aircraft's fuel consumption but also allows it to achieve higher speeds and better maneuverability. As aviation continues to evolve, the ongoing quest for reduced drag remains a critical aspect of ight innovation. The forces a ecting ight, including thrust, lift, weight, and drag, are fundamental concepts that underpin the science of aviation. They work together in a delicate balance to keep an aircraft airborne and maneuverable. By continuously studying and re ning our understanding of these forces, we can continue to push the boundaries of ight and explore the vast possibilities of the skies above.