Ross and Wilson ANATOMY and PHYSIOLOGY in Health and Illness PDF
Document Details
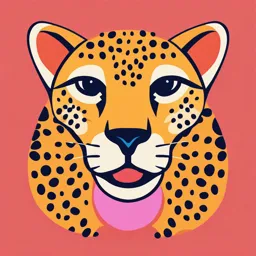
Uploaded by SpiritualDialogue
Edinburgh Napier University, Glasgow Caledonian University
2010
Anne Waugh, Allison Grant
Tags
Related
- Anatomy, Physiology, & Disease: Foundations for the Health Professions PDF
- Physiology LC1 Introduction To Human Physiology PDF
- Ross and Wilson Anatomy and Physiology in Health PDF
- HBS&DS: Introduction to Human Body PDF
- Chapter 1: Introduction to the Human Body PDF
- Essentials of Anatomy and Physiology PDF
Summary
This is a textbook on human anatomy and physiology, suitable for students of healthcare and allied health professions. It covers the normal anatomy and physiology of body systems, followed by pathology. The eleventh edition includes many revised diagrams and new micrographs.
Full Transcript
Ross and Wilson ANATOMY and PHYSIOLOGY in Health and Illness Eleventh Edition Anne Waugh, BSc(Hons) MSc CertEd SRN RNT FHEA Senior Lecturer and Senior Teaching Fellow, Schoo...
Ross and Wilson ANATOMY and PHYSIOLOGY in Health and Illness Eleventh Edition Anne Waugh, BSc(Hons) MSc CertEd SRN RNT FHEA Senior Lecturer and Senior Teaching Fellow, School of Nursing, Midwifery and Social Care, Edinburgh Napier University, Edinburgh, UK Allison Grant, BSc PhD RGN Lecturer, Department of Biological and Biomedical Sciences, Glasgow Caledonian University, Glasgow, UK Churchill Livingstone Front matter Senior Commissioning Editor: Ninette Premdas Development Editor: Clive Hewat Project Manager: Elouise Ball Designer: Kirsteen Wright Illustration Manager: Merlyn Harvey Illustrator: Graeme Chambers Ross and Wilson ANATOMY and PHYSIOLOGY in Health and Illness 11th Edition Anne Waugh BSc(Hons) MSc CertEd SRN RNT FHEA, Senior Lecturer and Senior Teaching Fellow, School of Nursing, Midwifery and Social Care, Edinburgh Napier University, Edinburgh, UK Allison Grant BSc PhD RGN, Lecturer, Department of Biological and Biomedical Sciences, Glasgow Caledonian University, Glasgow, UK Illustrations by Graeme Chambers Edinburgh London New York Oxford Philadelphia St Louis Sydney Toronto 2010 Copyright © E. & S. Livingstone Ltd 1963, 1966, 1968 © Longman Group Limited 1973, 1981, 1987, 1990 © Pearson Professional Limited 1997 © Harcourt Brace and Company Limited 1998 © Harcourt Publishers Limited 2001 © Elsevier Science Limited 2002. All rights reserved. © Elsevier Limited 2006. All rights reserved. © Elsevier Limited 2010. All rights reserved. No part of this publication may be reproduced or transmitted in any form or by any means, electronic or mechanical, including photocopying, recording, or any information storage and retrieval system, without permission in writing from the publisher. Details on how to seek permission, further information about the Publisher’s permissions policies and our arrangements with organizations such as the Copyright Clearance Center and the Copyright Licensing Agency, can be found at our website: www.elsevier.com/permissions. This book and the individual contributions contained in it are protected under copyright by the Publisher (other than as may be noted herein). ISBN 9780702032271 International edition ISBN 9780702032288 British Library Cataloguing in Publication Data A catalogue record for this book is available from the British Library Library of Congress Cataloging in Publication Data A catalog record for this book is available from the Library of Congress Notices Knowledge and best practice in this field are constantly changing. As new research and experience broaden our understanding, changes in research methods, professional practices, or medical treatment may become necessary. Practitioners and researchers must always rely on their own experience and knowledge in evaluating and using any information, methods, compounds, or experiments described herein. In using such information or methods they should be mindful of their own safety and the safety of others, including parties for whom they have a professional responsibility. To the fullest extent of the law, neither the Publisher nor the authors, contributors, or editors, assume any liability for any injury and/or damage to persons or property as a matter of products liability, negligence or otherwise, or from any use or operation of any methods, products, instructions, or ideas contained in the material herein. The Publisher Printed in China Preface Anne Waugh, Allison Grant, Edinburgh, 2009 Ross and Wilson has been a core text for students of anatomy and physiology for over 40 years. This latest edition is aimed at healthcare professionals including nurses, students of nursing, the allied health professions and complementary therapies, paramedics and ambulance technicians, many of whom have found previous editions invaluable. It retains the straightforward approach to the description of body systems and how they work, and the normal anatomy and physiology is followed by a section that covers common disorders and diseases: pathology. The human body is described system by system. The reader must, however, remember that physiology is an integrated subject and that, although the systems are considered in separate chapters, they must all function cooperatively to maintain health. The first three chapters provide an overview of the body and describe its main structures. The later chapters are organised into three further sections, reflecting those areas essential for normal body function: communication; intake of raw materials and elimination of waste; and protection and survival. Much of the material for this edition has been revised and rewritten. Many of the diagrams have been revised and over 50 new coloured electron micrographs and photographs included to provide detailed and enlightening views of many anatomical features. This edition is accompanied by a companion website (www.rossandwilson.com) with over 100 animations and an extensive range of new online self-test activities that reflect the content of each chapter. The material in this textbook is also supported by a new edition of the accompanying study guide, which provides students who prefer paper-based activities the opportunity to test their learning and improve their revision skills. Most features from the previous edition have been retained and revised, including learning outcomes, a list of common prefixes, suffixes and roots, and extensive in-text cross-references. A new and comprehensive glossary has been prepared for this edition. Some biological values, extracted from the text, are presented as an appendix for easy reference. In some cases, slight variations in ‘normals’ may be found in other texts and used by different medical practitioners. Acknowledgements Author’s acknowledgements The eleventh edition of this textbook would not have been possible without the efforts of many people. In preparing this edition, we have continued to build on the foundations established by Kathleen Wilson and we would like to acknowledge her immense contribution to the success of this title. Thanks are due once again to Graeme Chambers for his patience in the preparation of the new and revised artwork. We are indebted to readers of the tenth edition for their feedback and constructive comments, many of which have influenced the current revision. We are also grateful to the staff of Elsevier, particularly Ninette Premdas, Clive Hewat, Elouise Ball, Colin McEwan, Kirsty Guest and Fraser Johnston for their continuing support. Thanks are also due to our families, Andy, Michael, Seona and Struan, for their continued patience, support and acceptance of lost evenings and weekends. Publisher’s acknowledgements The following figures are reproduced with kind permission from: Elsevier Health Sciences Ltd: Figures 3.14, 5.3 and 12.21B from Telser AG, Young JK, Baldwin KM (2007) Elsevier’s integrated histology. Mosby, Edinburgh Figures 3.25, 7.11 and 13.19 from Young B, Lowe JS, Stevens A et al (2006) Wheater’s functional histology: a text and colour atlas. Churchill Livingstone, Edinburgh Figures 3.7, 3.8, 7.12, 7.13 and 17.1 from Standring S et al (2005) Gray’s anatomy: the anatomical basis of clinical practice, 39th edn. Churchill Livingstone, Edinburgh. The Science Photo Library: Figures 1.1, 1.6, 1.15, 1.17; 3.2B, 3.3 3.6 3.3 3.4 3.53.6 3.9, 3.12, 3.15C, 3.16B, 3.17B, 3.21B, 3.22B; 4.3C, 4.13; 6.6; 7.4, 7.18A; 8.2, 8.11C, 8.12, 8.25C; 9.13 9.16 9.13 9.149.159.16 10.8A, 10.12B, 10.19, 10.28; 12.5B, 12.20, 12.26, 12.47; 13.6, 13.7, 13.9; 14.1, 14.3, 14.4; 15.1; 16.4, 16.5B, 16.7, 16.55, 16.57; 17.2; 18.8. Common prefixes, suffixes and roots Prefix/suffix/root To do with Examples in the text a-/an- lack of anuria, agranulocyte, asystole, anaemia ab- away from abduct ad- towards adduct -aemia of the blood anaemia, hypoxaemia, uraemia, hypovolaemia angio- vessel angiotensin, haemangioma ante- before, in front of anterior anti- against antidiuretic, anticoagulant, antigen, antimicrobial -blast germ, bud reticuloblast, osteoblast brady- slow bradycardia broncho- bronchus bronchiole, bronchitis, bronchus card- heart cardiac, myocardium, tachycardia chole- bile cholecystokinin, cholecystitis, cholangitis circum- around circumduction cyto-/-cyte cell erythrocyte, cytosol, cytoplasm, cytotoxic derm- skin dermatitis, dermatome, dermis di- two disaccharide, diencephalon dys- difficult dysuria, dyspnoea, dysmenorrhoea, dysplasia -ema swelling oedema, emphysema, lymphoedema endo- inner endocrine, endocytosis, endothelium enter- intestine enterokinase, gastroenteritis epi- upon epimysium, epicardium erythro- red erythrocyte, erythropoietin, erythropoiesis exo- outside exocytosis, exophthalmos extra- outside extracellular, extrapyramidal -fferent carry afferent, efferent gast- stomach gastric, gastrin, gastritis, gastrointestinal -gen- origin/production gene, genome, genetic, antigen, pathogen, allergen -globin protein myoglobin, haemoglobin haem- blood haemostasis, haemorrhage, haemolytic hetero- different heterozygous homo- the same, steady homozygous, homologous -hydr- water dehydration, hydrostatic, hydrocephalus hepat- liver hepatic, hepatitis, hepatomegaly, hepatocyte hyper- excess/above hypertension, hypertrophy, hypercapnia hypo- below/under hypoglycaemia, hypotension, hypovolaemia intra- within intracellular, intracranial, intraocular -ism condition hyperthyroidism, dwarfism, rheumatism -itis inflammation appendicitis, hepatitis, cystitis, gastritis lact- milk lactation, lactic, lacteal lymph- lymph tissue lymphocyte, lymphatic, lymphoedema lyso-/-lysis breaking down lysosome, glycolysis, lysozyme -mega- large megaloblast, acromegaly, splenomegaly, hepatomegaly micro- small microbe, microtubules, microvilli myo- muscle myocardium, myoglobin, myopathy, myosin neo- new neoplasm, gluconeogenesis, neonate nephro- kidney nephron, nephrotic, nephroblastoma, nephrosis neuro- nerve neurone, neuralgia, neuropathy -oid resembling myeloid, sesamoid, sigmoid olig- small oliguria -ology study of cardiology, neurology, physiology -oma tumour carcinoma, melanoma, fibroma -ophth- eye xerophthalmia, ophthalmic, exophthalmos -ory referring to secretory, sensory, auditory, gustatory os-, osteo- bone osteocyte, osteoarthritis, osteoporosis -path- disease pathogenesis, neuropathy, nephropathy -penia deficiency of leukopenia, thrombocytopenia phag(o)- eating phagocyte, phagocytic -plasm substance cytoplasm, neoplasm pneumo- lung/air pneumothorax, pneumonia, pneumotoxic poly- many polypeptide, polyuria, polycythaemia -rrhagia excessive flow menorrhagia -rrhoea discharge dysmenorrhoea, diarrhoea, rhinorrhoea -scler hard arteriosclerosis sub- under subphrenic, subarachnoid, sublingual tachy- excessively fast tachycardia thrombo- clot thrombocyte, thrombosis, thrombin, thrombus -tox- poison toxin, cytotoxic, hepatotoxic tri- three tripeptide, trisaccharide -uria urine anuria, polyuria, haematuria, nocturia vas, vaso- vessel vasoconstriction, vas deferens, vascular Table of Contents Instructions for online access Front matter Copyright Preface Acknowledgements Common prefixes, suffixes and roots Section 1: The body and its constituents Introduction to the human body Introduction to the chemistry of life The cells, tissues and organisation of the body Section 2: Communication The blood The cardiovascular system The lymphatic system The nervous system The special senses The endocrine system Section 3: Intake of raw materials and elimination of waste The respiratory system Introduction to nutrition The digestive system The urinary system Section 4: Protection and survival The skin Resistance and immunity The musculoskeletal system Introduction to genetics The reproductive systems Glossary Normal values Bibliography Index Section 1 The body and its constituents CHAPTER 1 Introduction to the human body Levels of structural complexity 4 The internal environment and homeostasis 4 Homeostasis 5 Homeostatic imbalance 7 urvival needs of the body 7 Communication 7 nternal communication 9 ntake of raw materials and elimination of waste 11 rotection and survival 12 ntroduction to the study of illness 14 Aetiology 15 athogenesis 15 ANIMATIONS.1 Anatomy turntable 4.2 Cardiovascular (circulatory) system 8.3 Airflow through the lungs 11.4 The alimentary canal 11.5 Urine flow 12 The human body is rather like a highly technical and sophisticated machine. It operates as a single entity, but is made up of a number of systems that work interdependently. Each system is associated with a specific, and sometimes related, function that is normally essential for the well- being of the individual. Should one system fail, the consequences can extend to others, and may reduce the ability of the body to function normally. Integrated working of the body systems ensures survival. The human body is therefore complex in both structure and function, and this book aims to explain the fundamental structures and processes involved. Anatomy is the study of the structure of the body and the physical relationships between body systems. Physiology is the study of how the body systems work, and the ways in which their integrated activities maintain life and health of the individual. Pathology is the study of abnormalities and how they affect body functions, often causing illness. The final section of this chapter provides a framework for studying diseases, an outline of mechanisms that cause disease and some common disease processes. Building on the normal anatomy and physiology, relevant illnesses are considered at the end of the later chapters. Levels of structural complexity Learning outcome After studying this section you should be able to: describe the levels of structural complexity within the body. Within the body are different levels of structural organisation and complexity. The most fundamental level is chemical. Atoms combine to form molecules, of which there are a vast range in the body. The structures, properties and functions of important biological molecules are considered in Chapter 2. Cells are the smallest independent units of living matter and there are trillions of them within the body. They are too small to be seen with the naked eye, but when magnified using a microscope different types can be distinguished by their size, shape and the dyes they absorb when stained in the laboratory. Each cell type has become specialised, and carries out a particular function that contributes to body needs. Figure 1.1 shows some highly magnified nerve cells. In complex organisms such as the human body, cells with similar structures and functions are found together, forming tissues. The structure and functions of cells and tissues are explored in Chapter 3. Figure 1.1 Coloured scanning electron micrograph of some nerve cells (neurones). Organs are made up of a number of different types of tissue and have evolved to carry out a specific function. Figure 1.2 shows that the stomach is lined by a layer of epithelial tissue and that its wall contains layers of smooth muscle tissue. Both tissues contribute to the functions of the stomach, but in different ways. Figure 1.2 The levels of structural complexity. Systems consist of a number of organs and tissues that together contribute to one or more survival needs of the body. For example the stomach is one of several organs of the digestive system, which has its own specific function. The human body has several systems, which work interdependently carrying out specific functions. All are required for health. The structure and functions of body systems are considered in later chapters. The internal environment and homeostasis Learning outcomes After studying this section you should be able to: define the terms internal environment and homeostasis compare and contrast negative and positive feedback control mechanisms outline the potential consequences of homeostatic imbalance. The external environment surrounds the body and is the source of oxygen and nutrients required by all body cells. Waste products of cellular activity are eventually excreted into the external environment. The skin provides a barrier between the dry external environment (the atmosphere) and the aqueous (water-based) environment of most body cells. The internal environment is the water-based medium in which body cells exist. Cells are bathed in fluid called interstitial or tissue fluid. Oxygen and other substances they require must travel from the internal transport systems through the interstitial fluid to reach them. Similarly, cellular waste products must move through the interstitial fluid and transport systems to the excretory organs to be excreted. Each cell is enclosed by its plasma membrane, which provides a potential barrier to substances entering or leaving. The structure of membranes (p. 28) confers certain properties, in particular selective permeability or semipermeability. This controls the movement of molecules into and out of the cell, and allows the cell to regulate its internal composition (Fig. 1.3). Smaller particles can usually pass through the membrane, some much more readily than others, and therefore the chemical composition of the fluid inside is different from that outside the cell. Figure 1.3 Diagram of a cell with a semipermeable membrane. Homeostasis The composition of the internal environment is tightly controlled, and this fairly constant state is called homeostasis. Literally, this term means ‘unchanging’, but in practice it describes a dynamic, ever-changing situation kept within narrow limits. When this balance is threatened or lost, there is a serious risk to the well-being of the individual. Box 1.1 lists some important physiological variables maintained within narrow limits by homeostatic control mechanisms. Box 1.1 Examples of physiological variables Core temperature Water and electrolyte concentrations pH (acidity or alkalinity) of body fluids Blood glucose levels Blood and tissue oxygen and carbon dioxide levels Blood pressure Homeostasis is maintained by control systems that detect and respond to changes in the internal environment. A control system (Fig. 1.4) has three basic components: detector, control centre and effector. The control centre determines the limits within which the variable factor should be maintained. It receives an input from the detector, or sensor, and integrates the incoming information. When the incoming signal indicates that an adjustment is needed, the control centre responds and its output to the effector is changed. This is a dynamic process that allows constant readjustment of many physiological variables. Figure 1.4 Example of a negative feedback mechanism: control of room temperature by a domestic boiler. Negative feedback mechanisms In systems controlled by negative feedback, the effector response decreases or negates the effect of the original stimulus, maintaining or restoring homeostasis (thus the term negative feedback). Control of body temperature is similar to the non-physiological example of a domestic central heating system. The thermostat (temperature detector) is sensitive to changes in room temperature (variable factor). The thermostat is connected to the boiler control unit (control centre), which controls the boiler (effector). The thermostat constantly compares the information from the detector with the preset temperature and, when necessary, adjustments are made to alter the room temperature. When the thermostat detects the room temperature is low, it switches the boiler on. The result is output of heat by the boiler, warming the room. When the preset temperature is reached, the system is reversed. The thermostat detects the higher room temperature and turns the boiler off. Heat production from the boiler stops and the room slowly cools as heat is lost. This series of events is a negative feedback mechanism and it enables continuous self-regulation, or control, of a variable factor within a narrow range. Body temperature is a physiological variable controlled by negative feedback (Fig. 1.5), which prevents problems due to it becoming too high or too low. When body temperature falls below the preset level, this is detected by specialised temperature sensitive nerve endings in the hypothalamus of the brain, which form the control centre. This centre then activates mechanisms that raise body temperature (effectors). These include: stimulation of skeletal muscles causing shivering narrowing of the blood vessels in the skin reducing the blood flow to, and heat loss from, the peripheries behavioural changes, e.g. we put on more clothes or curl up. Figure 1.5 Example of a physiological negative feedback mechanism: control of body temperature. When body temperature rises within the normal range again, the temperature sensitive nerve endings are no longer stimulated, and their signals to the hypothalamus stop. Therefore, shivering stops and blood flow to the peripheries returns to normal. Most of the homeostatic controls in the body use negative feedback mechanisms to prevent sudden and serious changes in the internal environment. Many more of these are explained in the following chapters. Positive feedback mechanisms There are only a few of these cascade or amplifier systems in the body. In positive feedback mechanisms, the stimulus progressively increases the response, so that as long as the stimulus is continued the response is progressively amplified. Examples include blood clotting and uterine contractions during labour. During labour, contractions of the uterus are stimulated by the hormone oxytocin. These force the baby’s head into the cervix of the uterus, stimulating stretch receptors there. In response to this, more oxytocin is released, further strengthening the contractions and maintaining labour. After the baby is born the stimulus (stretching of the cervix) is no longer present so the release of oxytocin stops (see Fig. 9.5, p. 212). Homeostatic imbalance This arises when the fine control of a factor in the internal environment is inadequate and the level of the factor falls outside the normal range. If the control system cannot maintain homeostasis, an abnormal state develops that may threaten health, or even life. Many such situations, including effects of abnormalities of the variable factors in Box 1.1, are explained in later chapters. Survival needs of the body Learning outcomes After studying this section you should be able to: describe the roles of the body transport systems outline the roles of the nervous and endocrine systems in internal communication outline how raw materials are absorbed by the body state the waste materials eliminated from the body outline activities undertaken for protection and survival. By convention, body systems are described separately in the study of anatomy and physiology, but in reality they work interdependently. This section provides an introduction to body activities, linking them to survival needs (Table 1.1). The later chapters build on this framework, exploring human structure and functions in health and illness using a systems approach. Table 1.1 Survival needs and related body activities Survival need Body activities Transport systems: blood, circulatory system, lymphatic system Communication Internal communication: nervous system, endocrine system External communication: special senses, verbal and non-verbal communication Intake of oxygen Intake of raw materials and elimination of waste Ingestion of nutrients (eating) Elimination of wastes: carbon dioxide, urine, faeces Protection against the external environment: skin Protection against microbial infection: resistance and immunity Protection and survival Body movement Survival of the species: reproduction and transmission of inherited characteristics Communication In this section, transport and communication are considered. Transport systems ensure that all cells have access to the internal and external environments; the blood, the circulatory system and lymphatic system are involved. All communication systems involve receiving, collating and responding to appropriate information. There are different systems for communicating with the internal and external environments. Internal communication involves mainly the nervous and endocrine systems; these are important in the maintenance of homeostasis and regulation of vital body functions. Communication with the external environment involves the special senses, and verbal and non-verbal activities, and all of these also depend on the nervous system. Transport systems Blood (Ch. 4) The blood transports substances around the body through a large network of blood vessels. In adults the body contains 5 to 6 litres of blood. It consists of two parts – a fluid called plasma and blood cells suspended in the plasma. Plasma This is mainly water with a wide range of substances dissolved or suspended in it. These include: nutrients absorbed from the alimentary canal oxygen absorbed from the lungs chemical substances synthesised by body cells, e.g. hormones waste materials produced by all cells to be eliminated from the body by excretion. Blood cells There are three distinct groups, classified according to their functions (Fig. 1.6). Figure 1.6 Coloured scanning electron micrograph of blood showing red blood cells, white blood cells (yellow) and platelets (pink). Erythrocytes (red blood cells) transport oxygen and, to a lesser extent, carbon dioxide between the lungs and all body cells. Leukocytes (white blood cells) are mainly concerned with protection of the body against infection and foreign substances. There are several types of leukocytes, which carry out their protective functions in different ways. These cells are larger and less numerous than erythrocytes. Platelets (thrombocytes) are tiny cell fragments that play an essential part in blood clotting. Circulatory system (Ch. 5) This consists of a network of blood vessels and the heart (Fig. 1.7). Figure 1.7 The circulatory system. Blood vessels There are three types: arteries, which carry blood away from the heart veins, which return blood to the heart capillaries, which link the arteries and veins. Capillaries are tiny blood vessels with very thin walls consisting of only one layer of cells. They are the site of exchange of substances between the blood and body tissues, e.g. nutrients, oxygen and cellular waste products. Blood vessels form a network that transports blood to: the lungs (pulmonary circulation) where oxygen is absorbed from the air in the lungs and, at the same time, carbon dioxide is excreted from the blood into the air cells in all other parts of the body (general or systemic circulation). Heart The heart is a muscular sac, which pumps blood round the body and maintains the blood pressure. The heart muscle is not under conscious (voluntary) control. At rest, the heart contracts, or beats, between 65 and 75 times per minute. The rate is greatly increased during exercise, when body oxygen requirements are increased. The rate at which the heart beats can be counted by taking the pulse. The pulse can be felt most easily where a superficial artery can be pressed gently against a bone. The wrist is the site most commonly used for this purpose. Lymphatic system (Ch. 6) The lymphatic system (Fig. 1.8) consists of a series of lymph vessels, which begin as blind-ended tubes in the spaces between the blood capillaries and tissue cells. Structurally they are similar to veins and blood capillaries but the pores in the walls of the lymph capillaries are larger than those of the blood capillaries. Lymph is tissue fluid that also contains material drained from tissue spaces, including plasma proteins and, sometimes, bacteria or cell debris. It is transported along lymph vessels and returned to the bloodstream. Figure 1.8 The lymphatic system: lymph nodes and vessels. There are collections of lymph nodes situated at various points along the length of the lymph vessels. Lymph is filtered as it passes through the lymph nodes, and microbes and other materials are removed. The lymphatic system (Ch. 6) also provides the sites for formation and maturation of lymphocytes, the white blood cells involved in immunity (Ch. 15). Internal communication This is carried out through the activities of the nervous and endocrine systems. Nervous system (Ch. 7) The nervous system is a rapid communication system. The main components are shown in Figure 1.9. Figure 1.9 The nervous system. The central nervous system consists of: the brain, situated inside the skull the spinal cord, which extends from the base of the skull to the lumbar region and is protected from injury by the bones of the spinal column. The peripheral nervous system is a network of nerve fibres, which are: sensory or afferent nerves that transmit signals from the body to the brain, or motor or efferent nerves, which transmit signals from the brain to the effector organs, such as muscles and glands. The somatic (common) senses are pain, touch, heat and cold, and they arise following stimulation of specialised sensory receptors at nerve endings found throughout the skin. There are different receptors in muscles and joints that respond to changes in the position and orientation of the body, maintaining posture and balance. Yet other receptors are activated by stimuli in internal organs and control vital body functions, e.g. heart rate, respiratory rate and blood pressure. Stimulation of any of these receptors sets up impulses that are conducted to the brain in sensory (afferent) nerves. Communication along nerve fibres (cells) is by electrical impulses that are generated when nerve endings are stimulated. Nerve signals travel at great speed, so response to a nerve signal is almost immediate, making rapid and fine adjustments to body functions possible. Communication between nerve cells is also required, since more than one nerve is involved in the chain of events occurring between the initial stimulus and the reaction to it. Nerves communicate with each other by releasing a chemical (the neurotransmitter) into tiny gaps between them. The neurotransmitter quickly travels across the gap and either stimulates or inhibits the next nerve cell, thus ensuring the message is transmitted. Sensory nerves send signals from the body to the appropriate parts of the brain, where the incoming information is analysed and collated. The brain responds by sending signals along motor (efferent) nerves to the appropriate effector organ(s). In this way, many aspects of body function are continuously monitored and adjusted, usually by negative feedback control, and usually without conscious awareness, e.g. regulation of blood pressure. Reflex actions are fast, involuntary, and usually protective motor responses to specific stimuli. They include: withdrawal of a finger from a very hot surface constriction of the pupil in response to bright light control of blood pressure. Endocrine system (Ch. 9) The endocrine system consists of a number of discrete glands situated in different parts of the body. They synthesise and secrete chemical messengers called hormones that circulate round the body in the blood. Hormones stimulate target glands or tissues, influencing metabolic and other cellular activities and regulating body growth and maturation. Endocrine glands detect and respond to levels of particular substances in the blood, including specific hormones. Changes in blood hormone levels are usually controlled by negative feedback mechanisms (see Fig. 1.4). The endocrine system provides slower and more precise control of body functions than the nervous system. Communication with the external environment Special senses (Ch. 8) These senses arise following stimulation of specialised sensory receptor cells located in sensory organs or tissues in the head. The senses and the special organs involved are shown in Box 1.2. Box 1.2 The special senses and related sense organs Sight – eyes Hearing – ears Balance – ears Smell – nose Taste – tongue Although these senses are usually considered to be separate and different from each other, one sense is rarely used alone (Fig. 1.10). For example, when the smell of smoke is perceived then other senses such as sight and sound are used to try and locate the source of a fire. Similarly, taste and smell are closely associated in the enjoyment, or otherwise, of food. The brain collates incoming information with information from the memory and initiates a response by setting up electrical impulses in motor (efferent) nerves to effector organs, muscles and glands. Such responses enable the individual to escape from the fire, or to prepare the digestive system for eating. Figure 1.10 Combined use of the special senses: vision, hearing, smell and taste. Verbal communication Sound is a means of communication that is produced in the larynx as a result of blowing air through the space between the vocal cords (see Fig. 10.8) during expiration. Speech produces recognisable sounds by co-ordinated contraction of the muscles of the throat and cheeks, and movements of the tongue and lower jaw. Non-verbal communication Posture and movements are often associated with non-verbal communication, e.g. nodding the head and shrugging the shoulders. The skeleton provides the bony framework of the body (Ch. 16), and movement takes place at joints between bones. Skeletal muscles move the skeleton and attach bones to one another, spanning one or more joints in between. They are stimulated by the part of the nervous system under voluntary (conscious) control. Some non-verbal communication, e.g. changes in facial expression, may not involve the movement of bones. Intake of raw materials and elimination of waste This section considers substances taken into and excreted from the body. Oxygen, water and food are taken in, and carbon dioxide, urine and faeces are excreted. Intake of oxygen Oxygen gas makes up about 21% of atmospheric air. A continuous supply is essential for human life because it is needed for most chemical activities that take place in the body cells. Oxygen is necessary for the series of chemical reactions that result in the release of energy from nutrients. The upper respiratory system carries air between the nose and the lungs during breathing (Ch. 10). Air passes through a system of passages consisting of the pharynx (also part of the digestive tract), the larynx (voice box), the trachea, two bronchi (one bronchus to each lung) and a large number of bronchial passages (Fig. 1.11). These end in alveoli, millions of tiny air sacs in each lung. They are surrounded by a network of tiny capillaries and are the sites where vital gas exchange between the lungs and the blood takes place (Fig. 1.12). Figure 1.11 The respiratory system. Figure 1.12 Alveoli: the site of gas exchange. Nitrogen, which makes up about 80% of atmospheric air, is breathed in and out, but it cannot be used by the body in gaseous form. The nitrogen needed by the body is obtained by eating protein- containing foods, mainly meat and fish. Ingestion of nutrients (eating) Nutrition is considered in Chapter 11. A balanced diet is important for health and provides nutrients, substances that are absorbed, usually following digestion, and promote body function. Nutrients include water, carbohydrates, proteins, fats, vitamins and mineral salts. They serve vital functions including: maintenance of water balance within the body energy production, mainly carbohydrates and fats synthesis of large and complex molecules, using mineral salts, proteins, fats, carbohydrates and vitamins cell building, growth and repair, especially proteins. Digestion The digestive system evolved because food is chemically complex and seldom in a form body cells can use. Its function is to break down, or digest, food so that it can be absorbed into the circulation and then used by body cells. The digestive system consists of the alimentary canal and accessory glands (Fig. 1.13). Figure 1.13 The digestive system. Alimentary canal This is essentially a tube that begins at the mouth and continues through the pharynx, oesophagus, stomach, small and large intestines, rectum and anus. Glands The accessory organs situated outside the alimentary canal with ducts leading into it are the salivary glands, the pancreas and the liver. There are also many tiny glands situated in the walls of the alimentary canal. Most of these glands synthesise digestive enzymes that are involved in the chemical breakdown of food. Some others secrete mucus that provides lubrication. Metabolism This is the sum total of the chemical activity in the body. It consists of two groups of processes: anabolism, building or synthesising large and complex substances catabolism, breaking down substances to provide energy and raw materials for anabolism, and substances for excretion as waste. The sources of energy are mainly the carbohydrates and fats provided by the diet. If these are in short supply, proteins are used. Elimination of wastes Carbon dioxide This is a waste product of cellular metabolism. Because it dissolves in body fluids to make an acid solution, it must be excreted in appropriate amounts to maintain pH (acidity or alkalinity) within the normal range. The main route of carbon dioxide excretion is through the lungs during expiration. Urine This is formed by the kidneys, which are part of the urinary system (Ch. 13). The organs of the urinary system are shown in Figure 1.14. Urine consists of water and waste products mainly of protein breakdown, e.g. urea. Under the influence of hormones from the endocrine system, the kidneys regulate water balance. They also play a role in maintaining blood pH within the normal range. The bladder stores urine until it is excreted during micturition. Figure 1.14 The urinary system. Faeces The waste materials from the digestive system are excreted as faeces during defaecation. They contain: indigestible food residue that remains in the alimentary canal because it cannot be absorbed bile from the liver, which contains the waste products from the breakdown of red blood cells large numbers of microbes. Protection and survival In this section relevant activities are outlined under the following headings: protection against the external environment, protection against infection, movement and survival of the species. Protection against the external environment The skin (Fig. 1.15) forms a barrier against invasion by microbes, chemicals and dehydration (Ch. 14). It consists of two layers: the epidermis and the dermis. Figure 1.15 Coloured scanning electron micrograph of the skin. The epidermis lies superficially and is composed of several layers of cells that grow towards the surface from its deepest layer. The surface layer consists of dead cells that are constantly being rubbed off and replaced from below. The epidermis constitutes the barrier between the moist internal environment and the dry atmosphere of the external environment. The dermis contains tiny sweat glands that have little canals or ducts, leading to the surface. Hairs grow from follicles in the dermis. The dermis is rich in sensory nerve endings sensitive to pain, temperature and touch. It is a vast organ that constantly provides the central nervous system with sensory input from the body surfaces. The skin also plays an important role in regulation of body temperature. Protection against infection The body has many means of self-protection from invaders, which confer resistance and/or immunity (Ch. 15). They are divided into two categories: specific and non-specific defence mechanisms. Non-specific defence mechanisms These are effective against any invaders. The protection provided by the skin is outlined above. In addition there are other protective features at body surfaces, e.g. mucus secreted by mucous membranes traps microbes and other foreign materials on its sticky surface. Some body fluids contain antimicrobial substances, e.g. gastric juice contains hydrochloric acid, which kills most ingested microbes. Following successful invasion other non-specific processes that counteract potentially harmful consequences may occur, including the inflammatory response. Specific defence mechanisms The body generates a specific (immune) response against any substance it identifies as foreign. Such substances are called antigens and include: bacteria and other microbes cancer cells or transplanted tissue cells pollen from flowers and plants. Following exposure to an antigen, lifelong immunity against further invasion by the same antigen often develops. Over a lifetime, an individual gradually builds up immunity to millions of antigens. Allergic reactions are abnormally powerful immune responses to an antigen that usually poses no threat to the body. Movement Movement of the whole body, or parts of it, is essential for many body activities, e.g. obtaining food, avoiding injury and reproduction. Most body movement is under conscious (voluntary) control. Exceptions include protective movements that are carried out before the individual is aware of them, e.g. the reflex action of removing the finger from a very hot surface. The musculoskeletal system includes the bones of the skeleton, skeletal muscles and joints. The skeleton provides the rigid body framework and movement takes place at joints between two or more bones. Skeletal muscles (Fig. 1.16), under the control of the voluntary nervous system, maintain posture and balance, and move the skeleton. A brief description of the skeleton is given in Chapter 3, and a more detailed account of bones, muscles and joints is presented in Chapter 16. Figure 1.16 The skeletal muscles. Survival of the species Survival of a species is essential to prevent its extinction. This requires the transmission of inherited characteristics to a new generation by reproduction. Transmission of inherited characteristics Individuals with the most advantageous genetic make-up are most likely to survive, reproduce and pass their genes on to the next generation. This is the basis of natural selection, i.e. ‘survival of the fittest’. Chapter 17 explores the transmission of inherited characteristics. Reproduction (Ch. 18) Successful reproduction is essential in order to ensure the continuation of a species and its genetic characteristics from one generation to the next. Fertilisation (Fig. 1.17) occurs when a female egg cell or ovum fuses with a male sperm cell or spermatozoon. Ova are produced by two ovaries situated in the female pelvis (Fig. 1.18). Usually only one ovum is released at a time and it travels towards the uterus in the uterine tube. Spermatozoa are produced in large numbers by the two testes, situated in the scrotum. From each testis, spermatozoa pass through the deferent duct (vas deferens) to the urethra. During sexual intercourse (coitus) the spermatozoa are deposited in the vagina. Figure 1.17 Coloured scanning electron micrograph showing fertilisation (spermatozoon: orange, ovum: blue). Figure 1.18 The reproductive systems: male and female. They then swim upwards through the uterus and fertilise the ovum in the uterine tube. The fertilised ovum (zygote) then passes into the uterus, embeds itself in the uterine wall and grows to maturity during pregnancy or gestation, in about 40 weeks. When the ovum is not fertilised it is expelled from the uterus accompanied by menstrual bleeding, known as menstruation. In females, the reproductive cycle consists of phases associated with changes in hormone levels involving the endocrine system. A cycle takes around 28 days and they take place continuously between puberty and the menopause, except during pregnancy. At ovulation (Fig. 18.8) an ovum is released from one of the ovaries mid-cycle. There is no such cycle in the male but hormones, similar to those of the female, are involved in the production and maturation of spermatozoa. Introduction to the study of illness Learning outcomes After studying this section you should be able to: list mechanisms that commonly cause disease define the terms aetiology, pathogenesis and prognosis name some common disease processes. In order to understand the specific diseases described in later chapters, knowledge of the relevant anatomy and physiology is necessary, as well as familiarity with the pathological processes outlined below. There are many different illnesses, disorders and diseases, which vary from minor, but often very troublesome conditions, to the very serious. The study of abnormalities can be made much easier when a systematic approach is adopted. In order to achieve this in later chapters where specific diseases are explained, the headings shown in Box 1.3 will be used as a guide. Causes (aetiology) are outlined first when there are clear links between them and the effects of the abnormality (pathogenesis). Box 1.3 Suggested framework for understanding diseases Aetiology: cause of the disease Pathogenesis: the nature of the disease process and its effect on normal body functioning Complications: other consequences which might arise if the disease progresses Prognosis: the likely outcome Aetiology Diseases are usually caused by one or more of a limited number of mechanisms that may include: genetic abnormalities, either inherited or acquired infection by micro-organisms, e.g. bacteria, viruses, microbes or parasites, e.g. worms chemicals ionising radiation physical trauma degeneration, e.g. excessive use or ageing. In some diseases more than one of the aetiological factors listed above is involved, while in others, no specific cause has been identified and these may be described as essential, idiopathic or spontaneous. Although the precise cause of a disease may not be known, predisposing (risk) factors are usually identifiable. Iatrogenic conditions are those that result from healthcare interventions. Pathogenesis The main processes causing illness or disease are out-lined below. Box 1.4 contains a glossary of disease-associated terminology. Box 1.4 Glossary of terminology associated with disease Acute: a disease with sudden onset often requiring urgent treatment (compare with chronic). Acquired: a disorder which develops any time after birth (compare with congenital). Chronic: a long-standing disorder which cannot usually be cured (compare with acute). Congenital: a disorder which one is born with (compare with acquired). Sign: an abnormality seen or measured by people other than the patient. Symptom: an abnormality described by the patient. Syndrome: a collection of signs and symptoms which tend to occur together. Inflammation (p. 367) – this is a tissue response to any kind of tissue damage such as trauma or infection. Inflammatory conditions are recognised by the suffix -itis, e.g. appendicitis. Tumours (p. 49) – these arise when abnormal cells escape body surveillance and proliferate. The rate of their production exceeds that of normal cell death causing a mass to develop. Tumours are recognised by the suffix -oma, e.g. carcinoma. Abnormal immune mechanisms (p. 374) – these are responses of the normally protective immune system that cause undesirable effects. Thrombosis, embolism and infarction (p. 111) – these are the effects and consequences of abnormal changes in the blood and/or blood vessel walls. Degeneration – this is often associated with normal ageing but may also arise prematurely when structures deteriorate causing impaired function. Metabolic abnormalities – these cause undesirable metabolic effects, e.g. diabetes mellitus, page 227. Genetic abnormalities – these may be either inherited (e.g. phenylketonuria, p. 435) or caused by environmental factors such as exposure to ionising radiation (p. 49). For a range of self-assessment exercises on the topcs in this chapter, visit www.rossandwilson.com. CHAPTER 2 Introduction to the chemistry of life Atoms, molecules and compounds 18 Acids, alkalis and pH 21 mportant biological molecules 22 Carbohydrates 22 Amino acids and proteins 23 ipids 23 Nucleotides 24 nzymes 24 Movement of substances within body fluids 25 Body fluids 26 ANIMATIONS.1 Molecule formation 19.2 Chemical bonding 20.3 Diffusion and osmosis 25 Because living tissues are composed of chemical building blocks, the study of anatomy and physiology depends upon some understanding of biochemistry, the chemistry of life. This chapter introduces core concepts in chemistry that will underpin the remaining chapters in this book. Atoms, molecules and compounds Learning outcomes After studying this section, you should be able to: define the following terms: atomic number, atomic weight, isotope, molecular weight, ion, electrolyte, pH, acid and alkali describe the structure of an atom discuss the types of bond that hold molecules together outline the concept of molar concentration explain the importance of buffers in the regulation of pH. The atom is the smallest unit of an element that exists as a stable entity. An element is a substance containing only one type of atom, e.g. iron contains only iron atoms. When a substance contains two or more different types of atom, it is called a compound. For instance, water is a compound containing both hydrogen and oxygen atoms. There are 92 naturally occurring elements, but the wide variety of compounds that make up living tissues are composed almost entirely of only four: carbon, hydrogen, oxygen and nitrogen. Small amounts (about 4% of body weight) of others are present, including sodium, potassium, calcium and phosphorus. Atomic structure Atoms are mainly empty space, with a tiny central nucleus containing protons and neutrons surrounded by clouds of tiny orbiting electrons (Fig. 2.1). Neutrons carry no electrical charge, but protons are positively charged, and electrons are negatively charged. Because atoms contain equal numbers of protons and electrons, they carry no net charge. Figure 2.1 The atom, showing the nucleus and four electron shells. These subatomic particles differ also in terms of their mass. Electrons are so small that their mass is negligible, but the bigger neutrons and protons carry one atomic mass unit each. The physical characteristics of electrons, protons and neutrons are summarised in Table 2.1. Table 2.1 Characteristics of subatomic particles Particle Mass Electric charge Proton 1 unit 1 positive Neutron 1 unit neutral Electron negligible 1 negative Atomic number and atomic weight What makes one element different from another is the number of protons in the nuclei of its atoms (Fig. 2.2). This is called the atomic number and each element has its own atomic number, unique to its atoms. For instance, hydrogen has only one proton per nucleus, oxygen has eight and sodium has eleven. The atomic numbers of hydrogen, oxygen and sodium are therefore 1, 8 and 11 respectively. The atomic weight of an element is the sum of the protons and neutrons in the atomic nucleus. Figure 2.2 The atomic structures of the elements hydrogen, oxygen and sodium. The electrons are shown in Figure 2.1 as though they orbit in concentric rings round the nucleus. These shells diagrammatically represent the different energy levels of the electrons in relation to the nucleus, not their physical positions. The first energy level can hold only two electrons and is filled first. The second energy level can hold only eight electrons and is filled next. The third and subsequent energy levels hold increased numbers of electrons, each containing more than the preceding level. The electron configuration describes the distribution of the electrons in each element, e.g. sodium is 2 8 1 (Fig. 2.2). The chemistry of life depends upon the ability of atoms to react and combine with one another, to produce the wide range of molecules required for biological diversity. The atomic particles important for this are the electrons of the outermost shell. An atom is reactive when it does not have a stable number of electrons in its outer shell, and may donate, receive or share electrons with one or more other atoms to achieve stability. This will be described more fully in the section discussing molecules and compounds. Isotopes These are atoms of an element in which there is a different number of neutrons in the nucleus. This does not affect the electrical activity of these atoms because neutrons carry no electrical charge, but it does affect their atomic weight. For example, there are three forms of the hydrogen atom. The most common form has one proton in the nucleus and one orbiting electron. Another form (deuterium) has one proton and one neutron in the nucleus. A third form (tritium) has one proton and two neutrons in the nucleus and one orbiting electron. These three forms of hydrogen are called isotopes (Fig. 2.3). Figure 2.3 The isotopes of hydrogen. Because the atomic weight of an element is actually an average atomic weight calculated using all its atoms, the true atomic weight of hydrogen is 1.008, although for most practical purposes it can be taken as 1. Chlorine has an atomic weight of 35.5, because it contains two isotopes, one with an atomic weight of 35 (with 18 neutrons in the nucleus) and the other 37 (with 20 neutrons in the nucleus). Because the proportion of these two forms is not equal, the average atomic weight is 35.5. Molecules and compounds It was mentioned earlier that the atoms of each element have a specific number of electrons around the nucleus. When the number of electrons in the outer shell of an element is either the maximum number (Fig. 2.1), or a stable proportion of this fraction, the element is described as inert or chemically unreactive, i.e. it will not easily combine with other elements to form compounds. These elements are the inert gases – helium, neon, argon, krypton, xenon and radon. Molecules consist of two or more atoms that are chemically combined. The atoms may be of the same element, e.g. a molecule of atmospheric oxygen (O2) contains two oxygen atoms. Most molecules, however, contain two or more different elements, e.g. a water molecule (H2O) consists of two hydrogen atoms and an oxygen atom. As mentioned earlier, when two or more elements combine, the resulting molecule is referred to as a compound. Compounds that contain the elements carbon and hydrogen are classified as organic, and all others as inorganic. Living tissues are based on organic compounds, but the body requires inorganic compounds too. Covalent and ionic bonds The vast array of chemical processes on which life is based is completely dependent upon the way atoms come together, bind and break apart. For example, the simple water molecule is a crucial foundation of all life on Earth. If water was a less stable compound, and the atoms came apart easily, human biology could never have evolved. On the other hand, the body is dependent upon the breaking down of various molecules (e.g. sugars, fats) to release energy for cellular activities. When atoms are joined together, they form a chemical bond that is generally one of two types: covalent or ionic. Covalent bonds are formed when atoms share their electrons with each other. Most molecules are held together with this type of bond; it forms a strong and stable link between its constituent atoms. A water molecule is built using covalent bonds. Hydrogen has one electron in its outer shell, but the optimum number for this shell is two. Oxygen has six electrons in its outer shell, but the optimum number for this shell is eight. Therefore, if one oxygen atom and two hydrogen atoms combine, each hydrogen atom will share its electron with the oxygen atom, giving the oxygen atom a total of eight outer electrons, making it stable. The oxygen atom shares one of its electrons with each of the two hydrogen atoms, so that each hydrogen atom has two electrons in its outer shell, and they too are stable (Fig. 2.4). Figure 2.4 A water molecule, showing the covalent bonds between hydrogen (yellow) and oxygen (green). Ionic bonds are weaker than covalent bonds and are formed when electrons are transferred from one atom to another. For example, when sodium (Na) combines with chlorine (Cl) to form sodium chloride (NaCl) there is a transfer of the only electron in the outer shell of the sodium atom to the outer shell of the chlorine atom (Fig. 2.5). Figure 2.5 Formation of the ionic compound, sodium chloride. This leaves the sodium atom of the compound with eight electrons in its outer (second) shell, and therefore stable. The chlorine atom also has eight electrons in its outer shell, which, although not filling the shell, is a stable number. The sodium atom is now positively charged because it has given away a negatively charged electron, and the chloride ion is now negatively charged because it has accepted sodium’s extra electron. The two atoms, therefore, stick together because they are carrying opposite, mutually attractive, charges. When sodium chloride is dissolved in water the ionic bond breaks and the two atoms separate. The atoms are charged, because they have traded electrons, so are no longer called atoms, but ions. Sodium, with the positive charge, is a cation, written Na+, and chloride, being negatively charged, is an anion, written Cl−. By convention the number of electrical charges carried by an ion is indicated by the superscript plus or minus signs. Electrolytes An ionic compound, e.g. sodium chloride, dissolved in water is called an electrolyte because it conducts electricity. Electrolytes are important body constituents because they: conduct electricity, essential for muscle and nerve function exert osmotic pressure, keeping body fluids in their own compartments act as buffers to resist pH changes in body fluids. Many biological compounds, e.g. carbohydrates, are not ionic, and therefore have no electrical properties when dissolved in water. Important electrolytes other than sodium and chloride include potassium (K+), calcium (Ca2+), bicarbonate (HCO3−) and phosphate (PO42−). Molecular weight The molecular weight of a molecule is the sum of the atomic weights of the elements forming its molecules, e.g.: Water (H2O) 2 hydrogen atoms (atomic weight 1) 2 1 oxygen atom (atomic weight 16) 16 Molecular weight = 18 Sodium bicarbonate (NaHCO3) 1 sodium atom (atomic weight 23) 23 1 hydrogen atom (atomic weight 1) 1 1 carbon atom (atomic weight 12) 12 3 oxygen atoms (atomic weight 16) 48 Molecular weight = 84 Molecular weight, like atomic weight, is expressed simply as a figure until a scale of measurement of weight is applied. Molarity This is the commonest way to express the concentration of many substances in body fluids. The mole (mol) is the molecular weight in grams of a substance. One mole of any substance contains 6.023 × 1023 molecules or atoms. For example, 1 mole of sodium bicarbonate (the example above) is 84 g. In a molar solution, 1 mole of a substance is dissolved in 1 litre of solvent (dissolving fluid). In the human body the solvent is usually water. A molar solution of sodium bicarbonate is therefore prepared by dissolving 84 g of sodium bicarbonate in 1 litre of solvent. Molar concentration may be used to measure quantities of electrolytes, non-electrolytes, ions and atoms provided the molecular weight of the substance is known. It means that a molar solution of a substance contains exactly the same number of particles as any other molar solution. If the molecular weight of the substance is not known, or if there is more than one material in solution, another system of measuring concentration has to be used, such as grams per litre. The tiny quantities of many substances dissolved in body fluids mean that physiological concentrations are often expressed as fractions of a mole: millimoles/litre (thousandths of a mole) or micromoles/litre (millionths of a mole) (Table 2.2). Table 2.3 gives examples of the normal plasma levels of some important substances, given in molar concentrations and alternative units. Table 2.2 Molar concentrations Solute units Quantity per litre of solvent 1 mole of sodium chloride molecules (NaCl) 58.5 g 1 millimole of sodium chloride molecules 0.0585 g (58.5 mg) 1 mole of sodium ions 23 g 1 micromole of sodium ions 0.000023 g (23 μg) 1 mole of carbon atoms 12 g 1 mole of oxygen gas (O2) 32 g Table 2.3 Examples of normal plasma levels Substance Amount in moles Amount in other units Chloride 97–106 mmol/l 97–106 mEq/l* Sodium 135–143 mmol/l 135–143 mEq/l* Glucose 3.5–5.5 mmol/l 60–100 mg/100 ml Iron 14–35 mmol/l 90–196 mg/100 ml Acids, alkalis and pH The concentration of hydrogen ions ([H+]) in a solution is a measure of the acidity of the solution. Control of hydrogen ion levels in body fluids is an important factor in maintaining a stable internal environment. An acid substance releases hydrogen ions when in solution. On the other hand, a basic (alkaline) substance accepts hydrogen ions, often with the release of hydroxyl (OH−) ions. A salt releases other anions and cations when dissolved; sodium chloride is therefore a salt because in solution it releases sodium and chloride ions. * Milliequivalents per litre (mEq/l) Concentration is expressed as: The pH scale The standard scale for measurement of hydrogen ion concentration in solution is the pH scale. The scale measures from 0 to 14, with 7, the midpoint, as neutral; this is the pH of water. Water is a neutral molecule, neither acid nor alkaline, because when the molecule breaks up into its constituent ions, it releases one H+ and one OH−, which balance one another. Most body fluids are close to neutral, because strong acids and bases are damaging to living tissues, and body fluids contain buffers, themselves weak acids and bases, to keep their pH within narrow ranges. A pH reading below 7 indicates an acid solution, while readings above 7 indicate alkalinity (Fig. 2.6). A change of one whole number on the pH scale indicates a 10-fold change in [H+]. Therefore, a solution of pH 5 contains ten times as many hydrogen ions as a solution of pH 6. Figure 2.6 The pH scale. Not all acids ionise completely when dissolved in water. The hydrogen ion concentration is, therefore, a measure of the amount of dissociated acid (ionised acid) rather than of the total amount of acid present. Strong acids dissociate more freely than weak acids, e.g. hydrochloric acid dissociates freely into H+ and Cl−, while carbonic acid dissociates much less freely into H+ and HCO3−. Likewise, not all bases dissociate completely. Strong bases dissociate more fully, i.e. release more OH− than weaker ones. pH values of the body fluids The pH of body fluids must be maintained within relatively narrow limits depending on the fluid concerned. The normal range of pH values of some body fluids are shown in Table 2.4. Table 2.4 pH values of certain body fluids Body fluid pH Blood 7.35 to 7.45 Saliva 5.4 to 7.5 Gastric juice 1.5 to 3.5 Bile 6 to 8.5 Urine 4.5 to 8.0 The highly acid pH of the gastric juice is maintained by hydrochloric acid secreted by the parietal cells in the walls of the gastric glands. The low pH of the stomach fluids destroys microbes and toxins that may be swallowed in food or drink. Saliva has a pH of between 5.4 and 7.5, which is the optimum value for the action of salivary amylase, the enzyme present in saliva which initiates the digestion of carbohydrates. Amylase is destroyed by gastric acid when it reaches the stomach. Blood pH is kept between 7.35 and 7.45, and outwith this narrow range there is severe disruption of normal physiological and biochemical processes. Normal metabolic activity of body cells produces certain acids and alkalis, which would tend to alter the pH of the tissue fluid and blood. Chemical buffers are responsible for keeping body pH stable. Buffers Despite the constant cellular production of acid and alkaline substances, body pH is kept stable by systems of buffering chemicals in body fluids and tissues. These buffering mechanisms temporarily neutralise fluctuations in pH, but can function effectively only if there is some means by which excess acid or alkali can be excreted from the body. The organs most active in this way are the lungs and the kidneys. The lungs are important regulators of blood pH because they excrete carbon dioxide (CO2). CO2 increases [H+] in body fluids because it combines with water to form carbonic acid, which then dissociates into a bicarbonate ion and a hydrogen ion. The lungs, therefore, help to control blood pH by regulating levels of excreted CO2. The brain detects rising [H+] in the blood and stimulates breathing, causing increased CO2 loss and a fall in [H+]. Conversely, if blood pH becomes too alkaline, the brain can reduce the respiration rate to increase CO2 levels and increase [H+], restoring pH towards normal (see Ch. 10). The kidneys regulate blood pH by increasing or decreasing the excretion of hydrogen and bicarbonate ions as required. If pH falls, hydrogen ion excretion is increased and bicarbonate conserved; the reverse happens if pH rises. In addition, the kidneys generate bicarbonate ions as a by- product of amino acid breakdown in the renal tubules; this process also generates ammonium ions, which are rapidly excreted. Other buffer systems include body proteins, which absorb excess H+, and phosphate, which is particularly important in controlling pH inside cells. The buffer and excretory systems of the body together maintain the acid–base balance so that the pH range of the blood remains within normal, but narrow, limits. Acidosis and alkalosis The buffer systems described above compensate for most pH fluctuations, but these reserves are limited and, in extreme cases, can become exhausted. When the pH falls below 7.35, and all the reserves of alkaline buffers are used up, the condition of acidosis exists. In the reverse situation, when the pH rises above 7.45, the increased alkali uses up all the acid reserve and the state of alkalosis exists. Acidosis and alkalosis are both dangerous, particularly to the central nervous system and the cardiovascular system. In practice, acidotic conditions are commoner than alkalotic ones, because the body tends to produce more acid than alkali. Acidosis may follow respiratory problems, if the lungs are not excreting CO2 as efficiently as normal, or if the body is producing excess acids (e.g. diabetic ketoacidosis, p. 228) or in kidney disease, if renal H+ excretion is reduced. Alkalosis may be caused by loss of acidic substances through vomiting, diarrhoea, endocrine disorders or diuretic therapy, which stimulates increased renal excretion. Rarely, it may follow increased respiratory effort, such as in an acute anxiety attack where excessive amounts of CO2 are lost through overbreathing (hyperventilation). Important biological molecules Learning outcomes After studying this section, you should be able to: describe in simple terms the chemical nature of sugars, proteins, lipids, nucleotides and enzymes discuss the biological importance of each of these important groups of molecules. Carbohydrates Carbohydrates (sugars and starches) are composed of carbon, oxygen and hydrogen. The carbon atoms are normally arranged in a ring, with the oxygen and hydrogen atoms linked to them. The structures of glucose, fructose and sucrose are shown in Figure 2.7. When two sugars combine to form a bigger sugar, a water molecule is expelled and the bond formed is called a glycosidic linkage. Figure 2.7 The combination of glucose and fructose to make sucrose. Glucose, the main form in which sugar is used by cells, is a monosaccharide. Monosaccharides can be linked together to form bigger sugars, ranging in size from two sugar units (disaccharides), e.g. sucrose (Fig. 2.7) or table sugar, to long chains containing many thousands of monosaccharides e.g. starch. Such complex carbohydrates are called polysaccharides. Glucose can be broken down (metabolised) in either the presence (aerobically) or the absence (anaerobically) of oxygen, but the process is much more efficient when O2 is used. During this process, energy, water and carbon dioxide are released (p. 307). To ensure a constant supply of glucose for cellular metabolism, blood glucose levels are tightly controlled. The sugars: provide a ready source of energy to fuel cell metabolism (p. 307) provide a form of energy storage, e.g. glycogen (p. 307) form an integral part of the structure of DNA and RNA (pp. 429, 431) can act as receptors on the cell surface, allowing the cell to recognise other molecules and cells. Amino acids and proteins Amino acids always contain carbon, hydrogen, oxygen and nitrogen, and many in addition carry sulphur. In human biochemistry, 20 amino acids are used as the principal building blocks of protein, although there are others; for instance, there are some amino acids used only in certain proteins, and some are seen only in microbial products. Of the amino acids used in human protein synthesis, there is a basic common structure, including an amino group (NH2), a carboxyl group (COOH) and a hydrogen atom. What makes one amino acid different from the next is a variable side chain. The basic structure and three common amino acids are shown in Figure 2.8. As in formation of glycosidic linkages, when two amino acids join up the reaction expels a molecule of water and the resulting bond is called a peptide bond. Figure 2.8 Amino acid structures: A. Common structure, R = variable side chain. B. Glycine, the simplest amino acid. C. Alanine. D. Phenylalanine. Proteins are made from amino acids joined together, and are the main family of molecules from which the human body is built. Protein molecules vary enormously in size, shape, chemical constituents and function. Many important groups of biologically active substances are proteins, e.g.: carrier molecules, e.g. haemoglobin (p. 59) enzymes (p. 24) many hormones, e.g. insulin (p. 218) antibodies (pp. 371–372). Proteins can also be used as an alternative energy source, usually in starvation. In starvation, the main source of body protein is muscle tissue, so it is accompanied by wasting of muscles. Lipids The lipids are a diverse group of substances whose common property is an inability to mix with water (i.e. they are hydrophobic). They are made up of carbon, hydrogen and oxygen atoms. The most important groups of lipids include: phospholipids, integral to cell membrane structure. They form a double layer, providing a water- repellant barrier separating the cell contents from its environment (p. 28) certain vitamins (p. 270). The fat-soluble vitamins are A, D, E and K fats (triglycerides), stored in adipose tissue (p. 37) as an energy source. Fat also insulates the body and protects internal organs. A molecule of fat contains three fatty acids attached to a molecule of glycerol (Fig. 2.9). When fat is broken down under optimal conditions, more energy is released than when glucose is fully broken down. Figure 2.9 Structure of a fat (triglyceride) molecule. Fats are classified as saturated or unsaturated, depending on the chemical nature of the fatty acids present. Saturated fat tends to be solid, whereas unsaturated fats are fluid. prostaglandins are important chemicals derived from fatty acids and are involved in inflammation (p. 367) and other processes. cholesterol is a lipid made in the liver and available in the diet (p. 269). It is an integral part of cell membranes and is used to make steroid hormones (p. 216). Nucleotides Nucleic acids These are the largest molecules in the body and are built from nucleotides. They include deoxyribonucleic acid (DNA, p. 429) and ribonucleic acid (RNA, p. 431). Adenosine triphosphate (ATP) ATP is a nucleotide that contains ribose (the sugar unit), adenine (the base) and three phosphate groups attached to the ribose (Fig. 2.10A). It is sometimes known as the energy currency of the body, which implies that the body has to ‘earn’ (synthesise) it before it can ‘spend’ it. Many of the body’s huge number of reactions release energy, e.g. the breakdown of sugars in the presence of O2. The body captures the energy released by these reactions, using it to make ATP from adenosine diphosphate (ADP). When the body needs chemical energy to fuel cellular activities, ATP releases its stored energy, water and a phosphate group through the splitting of a high-energy phosphate bond, and reverts to ADP (Fig. 2.10B). Figure 2.10 ATP and ADP: A. Structures. B. Conversion cycle. The body needs chemical energy to: drive synthetic reactions (i.e. building biological molecules) fuel movement (locomotion) transport substances across membranes. Enzymes Many of the body’s chemical reactions can be reproduced in a test-tube. Surprisingly, the rate at which the reactions then occur usually plummets to the extent that, for all practical purposes, chemical activity ceases. The cells of the body have developed a solution to this apparent problem – they are equipped with a huge array of enzymes. Enzymes are proteins that act as catalysts for biochemical reactions – that is, they speed the reaction up but are not themselves changed by it, and therefore can be used over and over again. Enzymes are very selective and will usually catalyse only one specific reaction. The molecule(s) entering the reaction is called the substrate and it binds to a very specific site on the enzyme, called the active site. Whilst the substrate(s) is bound to the active site the reaction proceeds, and once it is complete the product(s) of the reaction breaks away from the enzyme and the active site is ready for use again (Fig. 2.11). Figure 2.11 Action of an enzyme: A. Enzyme and substrates. B. Enzyme–substrate complex. C. Enzyme and product. Enzyme action is reduced or stopped altogether if conditions are unsuitable. Increased or decreased temperature is likely to reduce activity, as is any change in pH. Some enzymes require the presence of a cofactor, an ion or small molecule that allows the enzyme to bind its substrate(s). Some vitamins are cofactors in enzyme reactions. Enzymes can catalyse both synthetic and breakdown reactions, and their names (almost always!) end in ˜ase. When an enzyme catalyses the combination of two or more substrates into a larger product, this is called an anabolic reaction. Catabolic reactions involve the breakdown of the substrate into smaller products, as occurs during the digestion of foods. Movement of substances within body fluids Learning outcomes After studying this section, you should be able to: compare and contrast the processes of osmosis and diffusion using these concepts, describe how molecules move within and between body compartments. Movement of substances within and between body fluids, sometimes across a barrier such as the cell membrane, is essential in normal physiology. From a physical point of view, substances will always travel from an area of high concentration to one of low concentration, assuming that there is no barrier in the way. Between two such areas, there exists a concentration gradient and movement of substances occurs down the concentration gradient, or downhill, until concentrations on each side are equal (equilibrium is reached). No energy is required for such movement, so this process is described as passive. There are many examples in the body of substances moving uphill, i.e. against the concentration gradient; in this case, chemical energy is required, usually in the form of ATP. These processes are described as active. Movement of substances across cell membranes by active transport is described on page 32. Passive movement of substances in the body proceeds usually in one of two main ways – diffusion or osmosis. Diffusion Diffusion refers to the movement of a chemical substance from an area of high concentration to an area of low concentration, and occurs mainly in gases, liquids and solutions. Sugar molecules heaped at the bottom of a cup of coffee that has not been stirred will, in time, become evenly distributed throughout the liquid by diffusion (Fig. 2.12). The process of diffusion is speeded up if the temperature rises and/or the concentration of the diffusing substance is increased. Figure 2.12 The process of diffusion: a spoonful of sugar in a cup of coffee. Diffusion can also occur across a semipermeable membrane, such as the plasma membrane or the capillary wall. Only molecules able to cross the membrane will be able to diffuse through. For example, oxygen diffuses freely through the walls of the alveoli (airsacs in the lungs), where oxygen concentrations are high, into the bloodstream, where oxygen concentrations are low. However, blood cells and large protein molecules in the plasma are too large to cross and so remain in the blood. Osmosis While diffusion of solute molecules across a semipermeable membrane results in equal concentrations of the solute on both sides of the membrane, osmosis refers specifically to diffusion of water down its concentration gradient. This is usually because the solute molecules are too large to pass through the pores in the membrane. The force with which this occurs is called the osmotic pressure. Imagine two solutions of sugar separated by a semipermeable membrane whose pores are too small to let the sugar molecules through. On one side, the sugar solution is twice as concentrated as on the other. After a period of time, the concentration of sugar molecules will have equalised on both sides of the membrane, not because sugar molecules have diffused across the membrane, but because osmotic pressure across the membrane pulls water from the dilute solution into the concentrated solution – i.e. water has moved down its concentration gradient. Osmosis proceeds until equilibrium is reached, at which point the solutions on each side of the membrane are of the same concentration and are said to be isotonic. The importance of careful control of solute concentrations in the body fluids can be illustrated by looking at what happens to a cell (e.g. a red blood cell) when it is exposed to solutions that differ from normal physiological conditions. Plasma osmolarity is maintained within a very narrow range because if the plasma water concentration rises, i.e. the plasma becomes more dilute than the intracellular fluid within the red blood cells, then water will move down its concentration gradient across their membranes and into the red blood cells. This may cause the red blood cells to swell and burst. In this situation, the plasma is said to be hypotonic. Conversely, if the plasma water concentration falls so that the plasma becomes more concentrated than the intracellular fluid within the red blood cells (the plasma becomes hypertonic), water passively moves by osmosis from the blood cells into the plasma and the blood cells shrink (Fig. 2.13). Figure 2.13 The process of osmosis. Net water movement when a red blood cell is suspended in solutions of varying concentrations (tonicity): A. Isotonic solution. B. Hypotonic solution. C. Hypertonic solution. Body fluids Learning outcomes After studying this section, you should be able to: define the terms intra- and extracellular fluid using examples, explain why homeostatic control of the composition of these fluids is vital to body function. The total body water in adults of average build is about 60% of body weight. This proportion is higher in babies and young people and in adults below average weight. It is lower in the elderly and in obesity in all age groups. About 22% of body weight is extracellular water and about 38% is intracellular water (Fig. 2.14). Figure 2.14 Distribution of body water in a 70 kg person. Extracellular fluid The extracellular fluid (ECF) consists mainly of blood, plasma, lymph, cerebrospinal fluid and fluid in the interstitial spaces of the body. Other extracellular fluids are present in very small amounts; their role is mainly in lubrication, and they include joint (synovial) fluid, pericardial fluid (around the heart) and pleural fluid (around the lungs). Interstitial or intercellular fluid (tissue fluid) bathes all the cells of the body except the outer layers of skin. It is the medium through which substances pass from blood to the body cells, and from the cells to blood. Every body cell in contact with the ECF is directly dependent upon the composition of that fluid for its well-being. Even slight changes can cause permanent damage, and ECF composition is, therefore, closely regulated. For example, a fall in plasma potassium levels may cause muscle weakness and cardiac arrhythmia, because of increased excitability of muscle and nervous tissue. Rising blood potassium also interferes with cardiac function, and can even cause the heart to stop beating. Potassium levels in the blood are only one of the many parameters under constant, careful adjustment by the homeostatic mechanisms of the body. Intracellular fluid The composition of intracellular fluid (ICF) is largely controlled by the cell itself, because there are selective uptake and discharge mechanisms present in the cell membrane. In some respects, the composition of ICF is very different from ECF. Thus, sodium levels are nearly ten times higher in the ECF than in the ICF. This concentration difference occurs because, although sodium diffuses into the cell down its concentration gradient, there is a pump in the membrane that selectively pumps it back out again. This concentration gradient is essential for the function of excitable cells (mainly nerve and muscle). Conversely, many substances are found inside the cell in significantly higher amounts than outside, e.g. ATP, protein and potassium. For a range of self-assessment exercises on the topcs in this chapter, visit www.rossandwilson.com. CHAPTER 3 The cells, tissues and organisation of the body The cell: structure and functions 28 lasma membrane 28 Organelles 28 he cell cycle 31 ransport of substances across cell membranes 31 Tissues 33 pithelial tissue 34 Connective tissue 35 Muscle tissue 38 Nervous tissue 40 issue regeneration 40 Membranes 40 Glands 41 Organisation of the body 41 Anatomical terms 41 The skeleton 41 Axial skeleton 43 Appendicular skeleton 45 Cavities of the body 45 Cranial cavity 45 horacic cavity 46 Abdominal cavity 46 elvic cavity 47 Disorders of cells and tissues 49 Neoplasms or tumours 49 Causes of neoplasms 49 Growth of tumours 50 ffects of tumours 51 Causes of death in malignant disease 51 ANIMATIONS.1 Cellular functions compared to organ systems 28.2 Mitosis 31.3 Selective permeability 31.4 Passive transport 32.5 Active transport 32.6 Mucous membrane 40.7 Serous membrane 40.8 Synovial membrane 40.9 Vertebral column 43.10 Anatomy and physiology of the abdomen 46 Cells are the smallest functional units of the body. They are grouped together to form tissues, each of which has a specialised function, e.g. blood, muscle, bone. Different tissues are grouped together to form organs, e.g. heart, stomach, brain. Organs are grouped together to form systems, each of which performs a particular function that maintains homeostasis and contributes to the health of the individual (see Fig. 1.2, p. 5). For example, the digestive system is responsible for taking in, digesting and absorbing food and involves a number of organs, including the stomach and intestines. The structure and functions of cells and types of tissue are explored in this chapter. The terminology used to describe the anatomical relationships of body parts, the skeleton and the cavities within the body are then described. The final section considers features of benign and malignant tumours, their causes and how they grow and may spread. The cell: structure and functions Learning outcomes After studying this section you should be able to: describe the structure of the plasma membrane explain the functions of the principal organelles outline the process of mitosis compare and contrast active, passive and bulk transport of substances across cell membranes. The human body develops from a single cell called the zygote, which results from the fusion of the ovum (female egg cell) and the spermatozoon (male sex cell). Cell division follows and, as the fetus grows, cells with different structural and functional specialisations develop, all with the same genetic make-up as the zygote. Individual cells are too small to be seen with the naked eye. However, they can be seen when thin slices of tissue are stained in the laboratory and magnified by a microscope. A cell consists of a plasma membrane inside which are a number of organelles suspended in a watery fluid called cytoplasm (Fig. 3.1). Organelles, literally ‘small organs’, have individual and highly specialised functions, and are often enclosed in their own membrane within the cytoplasm. They include: the nucleus, mitochondria, ribosomes, endoplasmic reticulum, Golgi apparatus, lysosomes and the cytoskeleton. Figure 3.1 The simple cell. Plasma membrane The plasma membrane (Fig. 3.2) consists of two layers of phospholipids (fatty substances, see p. 23) with protein and sugar molecules embedded in them. In addition to phospholipids, the lipid cholesterol is also present in the plasma membrane. Those proteins that extend all the way through the membrane may provide channels that allow the passage of, for example, electrolytes and non-lipid- soluble substances. Protein molecules on the surface of the plasma membrane are shown in Figure 3.2B. Figure 3.2 The plasma membrane. A. Diagram showing structure. B. Coloured atomic force micrograph of the surface showing plasma proteins. The phospholipid molecules have a head, which is electrically charged and hydrophilic (meaning ‘water loving’), and a tail which has no charge and is hydrophobic (meaning ‘water hating’, Fig. 3.2A). The phospholipid bilayer is arranged like a sandwich with the hydrophilic heads aligned on the outer surfaces of the membrane and the hydrophobic tails forming a central water-repelling layer. These differences influence the transfer of substances across the membrane. The membrane proteins perform several functions: branched carbohydrate molecules attached to the outside of some membrane protein molecules give the cell its immunological identity they can act as specific receptors (recognition sites) for hormones and other chemical messengers some are enzymes (p. 24) some are involved in transport across the membrane. Organelles Nucleus Every cell in the body has a nucleus, with the exception of mature erythrocytes (red blood cells). Skeletal muscle and some other cells contain several nuclei. The nucleus is the largest organelle and is contained within the nuclear envelope, a membrane similar to the plasma membrane but with tiny pores through which some substances can pass between it and the cytoplasm, i.e. the cell contents excluding the nucleus. The nucleus contains the body’s genetic material, which directs all the metabolic activities of the cell. This consists of 46 chromosomes, which are made from deoxyribonucleic acid (DNA, p. 428). Except during cell division, the chromosomes resemble a fine network of threads called chromatin. Within the nucleus is a roughly spherical structure called the nucleolus, which is involved in manufacture (synthesis) and assembly of the components of ribosomes. Mitochondria Mitochondria are membranous, sausage-shaped structures in the cytoplasm, sometimes described as the ‘power house’ of the cell (Fig. 3.3). They are involved in aerobic respiration, the processes by which chemical energy is made available in the cell. This is in the form of ATP, which releases energy when the cell breaks it down (see Fig. 2.10, p. 24). Synthesis of ATP is most efficient in the final stages of aerobic respiration, a process requiring oxygen (p. 308). The most active cell types have the greatest number of mitochondria, e.g. liver, muscle and spermatozoa. Figure 3.3 Mitochondrion and rough endoplasmic reticulum. False colour transmission electron micrograph showing mitochondrion (orange) and rough endoplasmic reticulum (turquoise) studded with ribosomes (dots). Ribosomes These are tiny granules composed of RNA and protein. They synthesise proteins from amino acids, using RNA as the template (see Fig. 17.5, p. 430). When present in free units or in small clusters in the cytoplasm, the ribosomes make proteins for use within the cell. These include the enzymes required for metabolism. Metabolic pathways consist of a series of steps, each driven by a specific enzyme. Ribosomes are also found on the outer surface of the nuclear envelope and rough endoplasmic reticulum (see Fig. 3.3 and below) where they manufacture proteins for export from the cell. Endoplasmic reticulum (ER) Endoplasmic reticulum is an extensive series of interconnecting membranous canals in the cytoplasm (Fig. 3.3). There are two types: smooth and rough. Smooth ER synthesises lipids and steroid hormones, and is also associated with the detoxification of some drugs. Some of the lipids are used to replace and repair the plasma membrane and membranes of organelles. Rough ER is studded with ribosomes. These are the site of synthesis of proteins, some of which are ‘exported’ from cells, i.e. enzymes and hormones that leave the parent cell by exocytosis (p. 33) to be used by cells elsewhere. Golgi apparatus The Golgi apparatus consists of stacks of closely folded flattened membranous sacs (Fig. 3.4). It is present in all cells but is larger in those that synthesise and export proteins. The proteins move from the endoplasmic reticulum to the Golgi apparatus where they are ‘packaged’ into membrane-bound vesicles called secretory granules. The vesicles are stored and, when needed, they move to the plasma membrane and fuse with it. The contents then leave the cell by exocytosis (p. 33). Figure 3.4 Coloured transmission electron micrograph showing the Golgi apparatus (green). Lysosomes Lysosomes are one type of secretory vesicle with membranous walls, which are formed by the Golgi apparatus. They contain a variety of enzymes involved in breaking down fragments of organelles and large molecules (e.g. RNA, DNA, carbohydrates, proteins) inside the cell into smaller particles that are either recycled, or extruded from the cell as waste material. Lysosomes in white blood cells contain enzymes that digest foreign material such as microbes. Cytoskeleton This consists of an extensive network of tiny protein fibres (Fig. 3.5). Figure 3.5 Fibroblasts. Fluorescent light micrograph showing their nuclei (purple) and cytoskeletons (yellow and blue). Microfilaments These are the smallest fibres. They provide structural support, maintain the characteristic shape of the cell and permit contraction, e.g. in muscle cells. Microtubules These are larger contractile protein fibres that are involved in movement of: organelles within the cell chromosomes during cell division cell extensions (see below). Centrosome This directs organisation of microtubules within the cell. It consists of a pair of centrioles (small clusters of microtubules) and plays an important role during cell division. Cell extensions These project from the plasma membrane in some types of cell and their main components are microtubules, which allow movement. They include: microvilli – tiny projections that contain microfilaments. They cover the surface of certain types of cell, e.g. absorptive cells that line the small intestine (see Fig. 3.6). By greatly increasing the surface area, microvilli make the structure of these cells ideal for their function – maximising absorption of nutrients from the small intestine. cilia – microscopic hair-like projections containing microtubules that lie along the free borders of some cells (see Fig. 10.12, p. 241). They beat in unison, moving substances along the surface, e.g. mucus upwards in the respiratory tract. flagella – single, long whip-like projections, containing microtubules, which form the ‘tails’ of spermatozoa (see Fig. 1.17, p. 14) that enable their movement along the female reproductive tract. Figure 3.6 Coloured scanning electron micrograph of microvilli in small intestine. The cell cycle Many damaged, dead, and worn out cells can be replaced by growth and division of other similar cells. Most body cells have 46 chromosomes and divide by mitosis, a process that results in two new genetically identical daughter cells. The only exception to this is the formation of gametes (sex cells), i.e. ova and spermatozoa, which takes place by meiosis (p. 432). The period between two cell divisions is known as the cell cycle, which has two phases that can be seen on light microscopy: mitosis (M phase) and interphase (Fig. 3.7). Figure 3.7 The cell cycle. Interphase This is the longer phase and three separate stages are recognised: first gap phase (G1) – the cell grows in size and volume. This is usually the longest phase and most variable in length. Sometimes cells do not continue round the cell cycle but enter a resting phase instead (G0). synthesis of DNA (S phase) – the chromosomes replicate forming two identical copies of DNA (see p. 432). Therefore, following the S phase, the cell now has 92 chromosomes, i.e. enough DNA for two cells and is nearly ready to divide by mitosis. second gap phase – (G2) there is further growth and preparation for cell division. Mitosis (Figs 3.8 and 3.9) This is a continuous process involving four distinct stages seen by light microscopy. Figure 3.8 The stages of mitosis. Figure 3.9 Mitosis. Light micrograph showing cells at different stages of reproduc