Psychology Chapter 10-12 PDF
Document Details
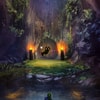
Uploaded by iiScholar
Arizona State University
Tags
Summary
This psychology chapter covers eye structure and function, visual processing, and parallel processing. It discusses how light interacts with the eye, how photoreceptors work, and how visual information is relayed to the brain. It also touches on concepts of feature detection and parallel processing within the visual system.
Full Transcript
**Eye Structure and Function** 10.1.01 Eye Structure and Function **Light** is an electromagnetic wave (ie, a form of electromagnetic radiation). Waves are characterized by several properties, including wavelength and amplitude. Wavelength, which describes the distance between the wave\'s peaks, d...
**Eye Structure and Function** 10.1.01 Eye Structure and Function **Light** is an electromagnetic wave (ie, a form of electromagnetic radiation). Waves are characterized by several properties, including wavelength and amplitude. Wavelength, which describes the distance between the wave\'s peaks, determines light\'s hue (color), whereas amplitude, which describes the wave\'s height, determines light\'s intensity (contributing to brightness). The [electromagnetic spectrum](javascript:void(0)) arranges the different types of waves by wavelength, ranging from very long wavelength radio waves to very small wavelength gamma rays. The visible light spectrum is the part of the electromagnetic spectrum that the human eye can detect. The electromagnetic spectrum shows that different colors of visible light have different wavelengths (eg, the wavelength of green light is around 500 nm, and the wavelength of red light is around 750 nm). Visible lights often comprise different wavelengths, determining light\'s purity (the degree of mixture). During the process of **vision**, light passes through several eye structures (Figure 10.1) before reaching the **retina**, the layer in the back of the eye containing photoreceptors. The outer layer of the eye is made up of two continuous segments. At the front of the eye, the cornea covers the pupil (ie, the opening in the eye\'s center that enables light to travel through the eye) and iris (ie, the colored part of the eye containing muscle that reacts to light\'s brightness by dilating or constricting the pupil). A second structure, the sclera (sometimes referred to as the white of the eye), makes up the outer layer of the rest of the eye. **Figure 10.1** Selected structures involved in vision. The **lens** is a transparent structure that changes shape to sharpen the image projected onto the retina. The term [accommodation](javascript:void(0)) describes when the shape or thickness of the eye\'s lens changes to view objects that are close or far away. Cells called **photoreceptors** (ie, rods and cones) in the retina convert light to neural signals. **Rods** are the receptor cells of the eye that detect movement, as well as black, white, and gray, whereas **cones** detect color. The **fovea**, a small pit in the retina with the [greatest concentration of cones](javascript:void(0)), has the highest level of visual acuity (ie, sharpness). Rods are more densely concentrated in the periphery of the retina and are not found in the fovea. Signals from photoreceptors are passed to other retinal cells such as [bipolar cells](javascript:void(0)) and then transmitted to **ganglion cells**. The axons of retinal ganglion cells, which make up the **optic nerve**, send the information to the thalamus, where it is then relayed to the visual cortex (in the brain\'s occipital lobe). The optic disc is the region of the retina where the optic nerve exits the back of the eye and the vessels that supply blood to the retina enter. There are no photoreceptors in this area, resulting in a blind spot: a small gap in the visual field. The brain automatically fills in this blind spot, so it usually goes undetected A diagram of a human eye Description automatically generated **Visual Processing** 10.2.01 Visual Processing As Lesson 10.1 states, the optic nerve (comprising the axons of retinal ganglion cells) relays visual information from the eyes. At the optic chiasm, some axons cross over, carrying messages to the opposite brain hemisphere, and other axons continue in the same hemisphere. From the optic chiasm, the axons (now called the optic tract) travel to the **thalamus**, where they synapse in the **lateral geniculate nucleus** (LGN), the region of the thalamus that receives visual input. From the LGN, visual information is transmitted to the **primary visual cortex** (V1) of the [occipital lobe](javascript:void(0)), which is the primary site of visual processing. Another area that receives visual input directly from the optic tract is the midbrain\'s superior colliculus, which also contributes to visual processing. **Feature Detection** David Hubel and Torsten Wiesel studied the responses of some of V1\'s neurons. Their work showed that cells called **feature detectors** respond only to specific visual stimuli. For example, some of these neurons fire only in response to lines at one angle, and others fire only in response to lines at other angles (Figure 10.2). Other cells in the visual system respond to more complex stimuli (eg, faces). **Figure 10.2** Feature detection. **Parallel Processing** Cells in V1 that are selectively responsive to orientation (ie, some feature detectors) explain the processing of just one aspect of visual stimuli. **Parallel processing** is the brain\'s ability to process different aspects of a stimulus simultaneously. For visual stimuli, the brain processes information about form/color and motion separately but at the same time (ie, in parallel). LGN and V1 are organized into fairly segregated layers or regions. Information from the retina is transmitted to these regions in LGN and V1 via relatively separate pathways. The **parvo pathway**, which travels to V1 through the parvocellular layers of the LGN, includes the neurons responsible for the  Chapter 10: Vision 60 perception of fine object shape. Conversely, the **magno pathway**, which travels to V1 through the magnocellular layers of the LGN, includes neurons responsible for the perception of object motion. Visual information then travels from V1 to other brain areas in two major streams. The **ventral stream**, also known as the \"what\" pathway, projects toward the temporal lobe and is involved in the perception of form and color. The **dorsal stream**, also known as the \"where\" pathway, projects toward the parietal lobe and is involved in the perception of motion. **Color Perception** The processing and perception of color begin with the cells that detect color in the retina. Two theories explain the perception of color: trichromatic theory and opponent process theory. The **trichromatic theory** states that color vision results from three types of color receptors (ie, cones) that are most sensitive to red, blue, or green. The **opponent process theory** states that color vision results from cells that respond to color in opposing pairs: red-green and blue-yellow. An opponent process cell responds to one color of the pair (eg, red) and is inhibited by the other color (eg, green). Therefore, both colors cannot be processed at the same time. The phenomenon of [afterimages](javascript:void(0)) demonstrates the opponent process theory: Looking at one of the colors (eg, red) fatigues opponent process cells, resulting in seeing the other color (eg, green) on looking away. **Ear Structure and Function** 11.1.01 Ear Structure and Function Specialized components in the outer, middle, and inner ear (Figure 11.1) contribute to **audition** (ie, hearing). The pinna, the visible part of the outer ear, funnels sound to the middle ear. Sound waves vibrate the tympanic membrane (also called the eardrum), separating the outer ear from the middle ear, which then vibrates the middle ear\'s ossicles. The **ossicles** are three small bones (malleus or \"hammer,\" incus or \"anvil,\" and stapes or \"stirrup\") that transmit sound vibrations to the inner ear. **Figure 11.1** Selected structures in the ear. A diagram of the ear Description automatically generated Chapter 11: Hearing 62 The vibrations move the fluid in a spiraled inner ear structure called the **cochlea** (Figure 11.2). Inside the cochlea are three fluid-filled chambers, the middle of which houses the organ of Corti. The organ of Corti comprises the supporting cells and auditory mechanoreceptors that sit along the **basilar membrane**, a membrane at the base of the middle cochlear chamber. The movement of endolymph (fluid in the middle cochlear chamber) results in activation of the auditory receptors. The auditory receptors are sometimes referred to as **hair cells** because on top of them sit cilia (also called stereocilia) that bend in response to movement, causing depolarization of the cell. Signals from these auditory receptors are transmitted to spiral ganglion cells, whose axons make up the auditory nerve. The auditory nerve (a branch of the vestibulocochlear cranial nerve) then relays the sound information to the brain. **Figure 11.2** Selected structures in the cochlea. In addition to the cochlea, the inner ear also contains the semicircular canals and the otolith organs, structures that are important in the vestibular, rather than in the auditory, sense. These vestibular structures are discussed in more detail in Lesson 12.5 **Auditory Processing** 11.2.01 Auditory Processing After the auditory receptors [transduce](javascript:void(0)) sound, the auditory nerve, a branch of the vestibulocochlear cranial nerve, then carries the sound information from the inner ear to the medial geniculate nucleus (MGN) of the thalamus. The MGN relays the information to the [temporal lobe\'s](javascript:void(0)) primary auditory cortex, the brain\'s auditory processing area. Another key area that receives and processes auditory input is the midbrain\'s inferior colliculus. **Pitch Perception** In the process of hearing, the brain interprets sound stimuli. Pitch refers to how a sound is experienced as a high (eg, a whistle) or a low (eg, a bass drum) tone. Frequency, the length of sound waves, determines a sound\'s pitch. Two theories describe pitch perception: frequency theory and place theory (Figure 11.3). **Frequency theory** states that pitch perception occurs because the frequency of a sound wave (eg, 75 waves per second) corresponds with stimulation of the auditory nerve (eg, 75 pulses per second). In contrast, **place theory** states that pitch perception is based on the location where sounds activate receptors along the basilar membrane. Different frequencies activate receptors at specific locations **Auditory Localization** Auditory processing also includes auditory localization. **Auditory localization** describes how the brain perceives the source of sounds by comparing the difference between the arrival time and intensity of the sound hitting the two ears. **Somatosensation** 12.1.01 The Biological Underpinnings of Somatosensation **Somatosensation** is enabled by the activation of somatosensory receptors in the skin that detect touch, pain, vibration, temperature, and movement sensations. The somatosensory receptors [transduce](javascript:void(0)) this sensory information into neural impulses. Different [types of sensory receptors](javascript:void(0)) (see Concept 8.2.01) respond to different kinds of somatosensory stimuli (eg, touch, pain, temperature). Mechanoreceptors depolarize in response to mechanical stimulation caused by pressure, vibration, or movement. Thermoreceptors are sensitive to temperature (eg, depolarize in response to heat). Nociceptors are typically free nerve endings that respond to potentially harmful stimuli (ie, stimuli that can cause tissue damage), such as a sharp needle. Activation of nociceptors contributes to the perception of pain. 12.1.02 The Process of Somatosensation In the process of somatosensation, receptors [transduce](javascript:void(0)) somatosensory stimuli (eg, touch, temperature) into neural signals. In the context of somatosensation, afferent neurons are neurons that relay sensory information toward the spinal cord and brain. Following transduction, these sensory neurons then relay the somatosensory information from receptors through tracts in the spinal cord to the thalamus and then the primary somatosensory cortex (Figure 12.1). **Figure 12.1** The process of somatosensation. Chapter 12: Other Senses 66 As Concept 4.3.02 introduces, somatosensory information is processed in the [parietal lobe\'s](javascript:void(0)) somatosensory cortex. The parietal lobe is responsible for both somatosensation and [proprioception](javascript:void(0)) (awareness of one\'s body position in space). The most sensitive parts of the body (eg, fingers, tongue) have the most somatosensory receptors; subsequently, these sensitive regions of the body occupy a greater area of the somatosensory cortex (see Figure 12.2). **Figure 12.2** The primary somatosensory cortex. The brain\'s right and left hemispheres are each specialized for certain processes; this specialization is known as hemispheric lateralization. Each hemisphere controls touch and movement on the opposite side of the body. Thus, the somatosensory cortex in the right hemisphere processes sensory information from the left side of the body and vice versa. **Pain Processing** In addition to utilizing different receptor types, the touch pathway further differs from the pain pathway. The afferent sensory neurons relaying information from nociceptors differ (eg, in size, in myelination) from other types of somatosensory afferents. Information from nociceptors also ascends in different spinal tracts (eg, in a different region of the spinal cord) though it is also relayed through the thalamus. Pain serves as a warning of physiological damage. By drawing attention to the affected area (eg, a twisted ankle) and encouraging a change in behavior (eg, taking weight off that foot), pain can help prevent additional injury. As Concept 4.4.01 discusses, the [spinal reflex](javascript:void(0)) enables the body to withdraw from a painful stimulus before the sensory information reaches the brain. In this reflex arc, spinal interneurons process the sensory information before it travels to the brain and directly stimulate efferent motor neurons. One model for somatic (bodily) pain, the gate-control theory, states that pain signals to the brain are regulated by a neurological \"gate\" in the spinal cord. When open, the \"gate\" allows pain signals to reach the brain. However, massage or mental distractions can close the \"gate\" to block pain signals. For example, while concentrating during a game, an injured athlete feels less pain **Smell** 12.3.01 The Biological Underpinnings of Smell **Olfaction** (ie, smell) occurs when odorants (airborne odor molecules dissolved in mucus) stimulate **olfactory receptor neurons**, also called olfactory receptor cells. Odorants bind cilia on these receptors (located in the nasal cavity's olfactory epithelium), whose axons travel to the olfactory bulb (Figure 12.4). These axons collectively comprise the olfactory cranial nerve (also called the olfactory nerve). **Figure 12.4** The olfactory bulb. 12.3.02 The Process of Smell As Concept 12.3.01 describes, in the process of smell, olfactory chemoreceptors [transduce](javascript:void(0)) olfactory information. The olfactory receptor neurons stimulate neurons within the **olfactory bulb** (see Figure 12.5). Axons from these olfactory bulb neurons comprise the **olfactory tract**. The olfactory tract relays smell information to other brain areas, such as the olfactory cortex, the hippocampus (involved in memory and learning), and the amygdala (involved in emotion The olfactory pathway is distinct from the other sensory pathways in that information from all the other senses travels through the thalamus before reaching other brain areas. However, olfactory information bypasses the thalamus and is instead sent directly to cortical and temporal lobe structures. **The Kinesthetic Sense** 12.4.01 The Biological Underpinnings of the Kinesthetic Sense The **kinesthetic sense** is awareness of body position and movement (eg, knowing where one\'s limbs are without looking). In the kinesthetic process (ie, proprioception), mechanoreceptors called **proprioceptors**---located in skin, joints, tendons, and muscles---respond to stretching or movement (Figure 12.6). Afferent sensory neurons then relay information from the proprioceptors to the brain. **Figure 12.6** Proprioceptors. 12.4.02 The Kinesthetic Process As Concept 12.4.01 introduces, in the kinesthetic process, proprioceptors are stimulated in response to stretching or movement. Afferent sensory neurons relay information from proprioceptors through tracts in the spinal cord to the thalamus (Figure 12.7). The proprioceptive information is then transmitted to and processed in the parietal lobe\'s [somatosensory cortex](javascript:void(0))**Vestibular Sense** 12.5.01 The Biological Underpinnings of the Vestibular Sense The **vestibular sense** helps maintain the body\'s sense of balance and depends on two structures in the inner ear: the semicircular canals and the otolith organs. The **semicircular canals** are three perpendicular fluid-filled canals (Figure 12.8). In each canal, a gelatinous structure called the cupula contains the vestibular receptor cells, hair cells. Rotation of the head moves the endolymph (fluid) in the interior of the canals, which pushes on the cupula. Displacement of the cupula bends the hair cells\' cilia, causing the cells to depolarize. When a person spins and then stops suddenly, the fluid in the semicircular canals briefly continues to move. Consequently, the sensation of spinning continues, which can make it difficult to maintain balance. **Figure 12.8** Overview of semicircular canal structure and function. The other type of vestibular organs, also located in the inner ear, are the two **otolith organs**, the utricle and saccule (Figure 12.9). Both otolith organs contain hair cells that are located within a gelatinous membrane. On top of this membrane sit crystals that move when the head tilts. Movement of these crystals causes movement of the gelatinous membrane, which in turn bends the cilia of the hair cells, stimulating the hair cells to depolarize.  12.5.02 The Vestibular Process Together, the [semicircular canals and otolith organs](javascript:void(0)) provide the brain with information about movement of the head. The semicircular canals detect angular acceleration: when the head rotates, hair cells in the semicircular canals are stimulated, whereas the otolith organs detect linear acceleration: when the head tilts, hair cells in the otolith organs are stimulated. The hair cells in both structures transmit the sensory information to the brain via the vestibulocochlear cranial nerve. The **vestibulocochlear nerve** relays vestibular information directly to the cerebellum, as well as through the brainstem to the thalamus and then to the cerebral cortex. These areas coordinate posture and balance, for example, through eye movements and postural adjustments. In addition to the contributions of the vestibular structures, maintenance of spatial orientation and balance also relies on input from the visual system and the kinesthetic sense. Visual cues provide information about the relative orientation of the body, objects, and light within one\'s surroundings. Proprioceptors (see Lesson 12.4) in skeletal muscles, tendons, skin, and joints provide information about the body\'s position and movement. This integration of vestibular, visual, and kinesthetic inputs in order to maintain balance is an example of [sensory interaction](javascript:void(0)), which describes when the senses influence each other A person doing a handstand Description automatically generated