Production of Metal Powder Lecture (4) PDF
Document Details
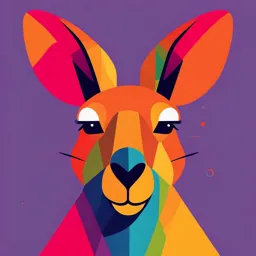
Uploaded by SaneNashville7918
Mohammed J. Kadhim
Tags
Summary
This lecture discusses the production of metal powders by various methods. Mechanical methods, such as machining and crushing, are explored and the subsequent pulverization processes are detailed.
Full Transcript
Production of Metal Powder Lecture (4) Asst. Prof. Dr. Mohammed J. Kadhim Powder Production Methods Methods for powder production are broadly classified as mechanical, chemical or physical methods based on the nature of energy used for production. Mechanical Methods: Mech...
Production of Metal Powder Lecture (4) Asst. Prof. Dr. Mohammed J. Kadhim Powder Production Methods Methods for powder production are broadly classified as mechanical, chemical or physical methods based on the nature of energy used for production. Mechanical Methods: Mechanical methods are among the cheapest employed for powder production. Mechanical methods involve the use of mechanical forces such as compressive force, shear or impact to effect particle size reduction of bulk materials. A number of methods are available to achieve size reduction. The different methods of milling are discussed in this section. Machining This method is employed to produce filings, turnings, scratching, chips, etc, which are subsequently pulverized by crushing and milling. Since relatively coarse and bulky powders entirely free from fine particles are obtained by this method, it is particularly suitable in a very few special cases; such as the production of magnesium powders for pyrotechnic application where the explosiveness and malleability of the powder would prohibit the use of other methods: beryllium powders, silver solders, and dental alloys (containing up to 70 % silver among its constituents). The powder particles produced are of irregular shape. This method is highly expensive and, therefore, has a limited application. This is especially employed where cost is not excessive in relation to the cost of the metals themselves or where the choice of the method is considered a necessity as in the case of Mg. Magnesium, beryllium, silver, solder and dental alloy powders are specifically made by machining. Turnings and chips obtained during machining are subsequently crushed or ground (to the desired degree of fineness) into powders. Crushing This method is mostly used for the disintegration of oxides (subsequently reduced to metal powders) and brittle materials. Any type of crushing equipment such as stamps, hammers, jaw crushers or gyratory crushes may be employed for crushing brittle materials. Various ferrous and nonferrous alloys can be heat treated in order to obtain a sufficiently brittle material which can be easily crushed into powder form. Some metals particularly titanium, zirconium, vanadium, niobium, and tantalum when heated to moderate temperature in hydrogen atmosphere is converted to brittle hydrides. The powders produced by this method are of angular shapes which are subsequently comminuted by milling to attain the required fineness of the powder for processing by P/M technique. Comminution equipment: (i) jaw crusher, (ii) gyratory crusher, (iii) roll crusher Particles and powders are classified according to particle size, particle size distribution, particle shape, and surface texture. These powder characteristics determine flowability, apparent density, color, sinterability, compactibility, and the properties of sintered products. Figure below illustrates the crushing of single particles between impacting balls. This condition is encountered in the crushing of relatively large particles or a fraction of a powder, as with large granules of a ceramic material, hard metals, or large atomized particles of a ductile metal powder. Under these conditions, many single-particle impact events occur. With ceramics and hard metals, large brittle particles are rapidly reduced to fine and ultrafine powders. The crushing of a brittle single particle is shown in Fig. (a). During crushing of ductile metals (Fig. b), individual particles do not fracture, but deform and thereby undergo shape change with little or no change in mass. Effect of impact. (a) Brittle single particle. (b) Ductile single spherical particle Milling Milling of materials is of economic importance for P/M industry since mechanical comminution of hard and brittle as well as soft and ductile materials is the most widely used method of powder production. Hammer and rod mills are used to mill spongy cakes of oxide-reduced, atomized or electrolytic powders. Mechanical comminution has been successfully employed for milling electrolytic iron, bismuth, beryllium, metal hydrides as well as chemically-embrittled materials like sensitized stainless steel. Mechanism of Milling Milling of coarse or bulk raw material occurs in stages. In the initial stage of milling of ductile materials, individual particles are deformed by microforging resulting in welding and fracture. Eventually the particles become so severely cold worked that they fracture into fragments of smaller and smaller dimensions resulting in agglomerates due to coupling forces. After a period of cold welding and fracturing, steady-state equilibrium is achieved. Constant kneading, fracturing and rewelding produce a composite structure containing fragmented composite particles. Changes in the morphology of powder particles during milling may lead to the following events: (i) Microforging. (ii) Fracture. (iii) Agglomeration. (iv) Deagglomeration Microforging refers to the process during milling in which individual particles or a group of particles are impacted repeatedly so that they flatten with very little change in mass. After a period of milling, individual particles deform to such an extent that cracks initiate and propagate resulting in fracture. Mechanical interlocking due to atomic bonding as well as autohesion resulting from vander Waal’s forces or welding may lead to agglomeration. The process that breaks up agglomerates is known as deagglomeration. Milling of metal powders results in external shape and internal structural changes. The extent of such changes is determined by milling parameters, milling environment and the physical and chemical properties of the metal or alloy powder being milled. The different powder characteristics influenced by milling are shape, texture, reflectivity, particle size, particle size distribution, crystallite size, chemical composition, hardness, apparent density, flowability, sinterability, compressibility and sintered density. Mechanical mills have been successfully employed to achieve innovative P/M products not possible by conventional processing. Some of these include oxide dispersion strengthened alloys, superconducting materials made from niobium and tin, silicon carbide whisker reinforced composites and intermetallics such as aluminides of titanium, nickel and iron. Principles of Milling--Phenomenological Description The impact process is shown in Fig. This model represents the moment of collision, at which particles are trapped between two colliding balls within a space occupied by a dense cloud, dispersion, or mass of powder particles. This phenomenon is typical in dry and wet milling operations that use colliding Fig. Model of impact event at milling mediums such as tumbler, a time of maximum impacting force showing the formation vibratory, and attrition ball mills. of a micro compact Griffith theory Brittle Fracture. The Griffith theory assumes that a brittle material has minute cracks dispersed within it and that fracture emanates from these cracks. The stress σc at which a crack propagates depends on the size of the crack. The general form of the fracture equation for modes of loading that lead to catastrophic failure is: where A is a numerical constant that depends on crack geometry and its location, mode of loading, and dimensions of the particle (for example, A is for the slit crack in an infinite sheet); c is the size of the crack, E is the modulus of elasticity, ì is Poisson's ratio, and is the surface energy of the milled substance. Thus, measured strength depends on the size of the crack, elastic properties, and surface energy. For brittle materials, ã is between 103 and 104 erg/cm2. The stress for fracture of a particle may be represented by: where L is the length of the crack, and r is the radius of the crack tip. Ball Milling Milling is the most important and widely used method of producing powders of the required grade of fineness. Milling or grinding can be classified as comminution of brittle, friable, tough and hard materials and pulverization of malleable and ductile metals. It involves the application of impact force on the material being comminuted. The milling action is carried out by the use of a wide variety of equipment such as ball mill, rod mill, impact mill, disk mill, eddy mill, vortex mill, etc. In the ball milling method, the material to be disintegrated is tumbled in the a container together with a large number of hard wear resistant solid balls which by hitting the materials, cause them to break down. The ball mills are of two types, rotary mill and vibratory mill. The latter produces equivalent grinding in a very short time as compared with the former type and more efficient grinding action takes place due to vibration with rotary motion. The powder so produced is also less contaminated because of smaller wear on balls and mills. The drums of rotary mills are usually made of stainless steel or steel lined with hard alloy plates. The balls are made of steel or hard alloy. Hard alloy balls contained in a drum lined with hard alloy plate are most frequently used since milling by other means will cause the contamination of the finished powder by iron (greater than 0.5 %) due to wear of the liners and balls. The fact that the critical speed of rotation of the ball mill should be maintained is very important for the proper milling action of the balls. It should be neither slow nor very high. The milling action will be insignificant due to the movement of the balls only in the lower part of the drum in the former case. The milling action will not be carried out correctly in the latter case because the centrifugal force will result in the clinging of balls to the walls of the drum whereby the required trumbling action will not be achieved. The most intense grinding action is produced at the critical speed of rotation of the drum whereby the balls are lifted up to the top part of the drum and fall down on the material to be ground. The milling action by eddy mill is based on a relatively new grinding principle which grinds through impact of the metal particles against each other. The eddy mill, consisting of two fans mounted at the opposite end of the entirely enclosed casing and rotating in opposite direction produces two high velocities but opposing gas-stream which carry the powder particles thus pulverizing them by collision. During the grinding operation, the metallic particles get heated, hardened and oxidized. In order to prevent oxidation and spontaneous combustion of the powder, the mill casing is furnished with a water jacket and inert or protective gas is continuously forced into the chamber. The powders produced by this mill are characterized by the saucer-like shape of the particles which is particularly suitable for the manufacture of sintered products. It is useful for: i. The production of extremely fine and very pure metal powder due to the complete elimination of contamination from either grinding balls or walls of the mill. ii. Comminuting of malleable metals such as aluminum, iron, copper and final comminution of brittle metals previously disintegrated to coarse particle size by other methods iii. Modification of powder particle shape such as flake powder so that it may become suitable for sintering. Another mill based on a similar principle is known as Micronizer in which high velocity (at the pressure of 100-500 Psi) jets of air or superheated steam in a particular direction is injected into the grinding chamber. Pure powders free from contamination may be produced in vortex mills in which the particles of the materials to be ground are fractured by mutual collision. Such a mill consists of two or more very rapidly rotating propellers within a relatively small mill casing and gas flow systems which remove desired size fractions of powder particles. Its advantages include: i. Simple mechanism of the mill. ii. Use of cheaper by product such as machine waste, etc, as the starting raw material. iii. Suitability for mass as well as small scale production of powders. Milling may be carried out in either dry or wet medium. Wet grinding is widely employed for attaining the specified quality of the product because of acceleration of comminution or reduction of milling time and absence of oxidation in the liquid medium. Distilled water, alcohol, acetone, paraffin, and stearic acid are examples of the liquid media used in wet milling. The main disadvantages of milling are i. work hardening. ii. excessive oxidation of the final powder iii. particle welding and agglomeration. The milling process is widely employed for carbide metal mixture and cermet materials to perform blending and particle size reduction. Vibratory Ball Mill Finer powder particles need longer periods for grinding In this case, vibratory ball mill is better => here high amount of energy is imparted to the particles and milling is accelerated by vibrating the container This mill contains an electric motor connected to the shaft of the drum by an elastic coupling. The drum is usually lined with wear resistant material. During operation, 80% of the container is filled with grinding bodies and the starting material. Here vibratory motion is obtained by an eccentric shaft that is mounted on a frame inside the mill. The rotation of eccentric shaft causes the drum of the vibrating mill to oscillate In general, vibration frequency is equal to 1500 to 3000 oscillations/min. The amplitude of oscillations is 2 to 3 mm. The grinding bodies is made of steel or carbide balls, that are 10-20 mm in diameter. The mass of the balls is 8-10 times the charged particles. Final particle size is of the order of 5-100 microns Attrition Mill Attrition Milling in an attrition mill (Fig.) is effected by the stirring action of an agitator that has a vertical rotating shaft with horizontal arms. This motion causes a differential movement between the balls and the material being milled, thus providing a substantially higher degree of surface contact than is achieved in tumbler or vibratory mills. Attrition mill: IN this case, the charge is ground to fine size by the action of a vertical shaft with side arms attached to it. The ball to charge ratio may be 5:1, 10:1, 15:1. This method is more efficient in achieving fine particle size. Attritors are the mills in which large quantities of powder (from a few pounds to 100 lb) can be milled at a time (Fig.). Commercial attritors are available from Union Process, Akron, OH. The velocity of the grinding medium is much lower ( 0.5 m/s) than in Fritsch or SPEX mills, and consequently the energy of the attritors is low. Attritors of different sizes and capacities are available. The grinding tanks or containers are available either in stainless steel or stainless steel coated inside with alumina, silicon carbide, silicon nitride, zirconia, rubber, and polyurethane. A variety of grinding media also is available--glass, flint stones, steatite ceramic, mullite, silicon carbide, silicon nitride, sialon, alumina, zirconium silicate, zirconia, stainless steel, carbon steel, chrome steel, and tungsten carbide. Fig. Model 1-S attritor. Rod Mills Rod mills: Horizontal rods are used instead of balls to grind. Granularity of the discharge material is 40-10 mm. The mill speed varies from 12 to 30 rpm. Rod mills are used in the P/M industry to mill large quantities of sinter cakes, which are intermediate products in the production of metal powders by reduction of their oxides. If such powders are to be used in conventional P/M parts processing, it is essential that they possess good green strength and compressibility characteristics. For these reasons, the sinter cakes must be ground into powder, typically - 80 mesh, while introducing minimal cold work and densification. Planetary Mill Planetary mill: High energy mill widely used for producing metal, alloy, and composite powders. The is the angular velocity of the ball mill plate, and is the angular velocity of the vials. Fluid energy grinding or Jet milling The basic principle of fluid energy mill is to induce particles to collide against each other at high velocity, causing them to fracture into fine particles Multiple collisions enhance the reduction process and therefore, multiple jet arrangements are normally incorporated in the mill design. The fluid used is either air about 0.7 MPa or stream at 2 MPa. In the case of volatile materials, protective atmosphere of nitrogen and carbon-di-oxide is used. The pressurized fluid is introduced into the grinding zone through specially designed nozzles which convert the applied pressure to kinetic energy. Also materials to be powdered are introduced simultaneously into the turbulent zone. The velocity of fluid coming out from the nozzles is directly proportional to the square root of the absolute temperature of the fluid entering the nozzle. Hence it is preferable to raise the temperature of fluid to the maximum possible level without affecting the feed material. If further powdering is required, large size particles are separated from the rest centrifugal forces and re-circulated into the turbulent zone for size reduction. Fine particles are taken to the exit by viscous drag of the exhaust gases to be carried away for collection. This Jet milling process can create powders of average particle size less than 5 μm Shotting The method consists essentially in pouring a fine stream of molten metal through a vibrating screen into air or neutral atmosphere. In this way, molten metal stream is disintegrated into a large number of droplets which solidify as spherical particles during its free fall. All metal and alloys can be shotted; the size and character of the resultant shot depending on the temperature of molten metal and gas, diameter of the holes and frequency of vibration in case of vibrating screen. One drawback of this process is the formation of high oxide content which can be minimized with the use of inert gas. The metal powders occasionally produced by this method for preliminary breakdown are those of copper, brass, aluminium, tin, zinc, gold, silver, lead, nickel, etc. Graining Graining involves the same procedure as the shotting the only difference being that the solidification is allowed to take place in water. In similar manner, other pulverization methods are used for the production of very fine powders. Frequently cadmium, zinc, tin, bismuth, antimony, and lead alloys are pulverized by this method. Same as shotting except that the falling material through sieve is collected in water; Powders of cadmium, Bismuth, antimony are produced. Atomization Atomization Basically atomization consists of mechanically disintegrating a stream of molten metal into the fine particles by means of a jet of compressed air, inert gases or water. The method has gained greater use because of the following advantages and benefits: 1. Virtually any material that can be melted can be made into powder by this technique 2. Relative ease of preparing higher purity metals, and pre-alloyed powder directly from the melt 3. Similar and uniform composition of atomized powder 4. Control of particle size, size distribution, shape and structure are made possible by the control of atomization processing parameters; i.e. liquid metal superheat or the atomization stream 5. Lower capital or investment cost 6. High productivity. For example, it is now possible to atomize 10 tons of molten steel within 30 minutes. This is the main process for preparing aluminium, zinc, lead, pure iron, and low alloy steel powders, noble metals and more recently, high temperature alloys and special alloy powders (e.g., superalloys) Cold Stream Process Cold Stream Process Cold stream process relies upon the brittleness of certain metals and alloys at low temperatures. The starting materials is coarse particles (often obtained by grinding or atomization) of the required composition. This is conveyed in a high velocity, high-pressure air stream through a vertical nozzle and strikes on a target in an evacuated blast chamber. At the nozzle, the pressure drop occurs at once from about (7 atmospheres to 1 atmospheric) and this results in a very quick temperature drop to subzero. The brittle raw material shatters against the target into an irregular shaped powder having very little surface contamination and excellent pressing characteristics. The resulting powder is separated into suitable size fraction using a classifier. This process has been used to produce tungsten, tantalum, tungsten carbide, and tool steel powders. Atomization In this process molten metal is broken up into small droplets by spraying it on the stream of inset gas or air jets and rapidly frozen before the drops come into contact with each other or with the solid surface this technique is applicable to all metals that can be melted and is used commercially for the production of iron, copper, brass, bronze, lead, zinc etc. Types of Atomization Atomization of molten metal can be done in different ways depending upon the factors like economy and required powder characteristics. At present, water or gas atomizing medium can be used to disintegrate a molten metal stream. The various types of atomization techniques used are: 1. Water atomization: High pressure water jets are used to bring about the disintegration of molten metal stream. Water jets are used mainly because of their higher viscosity and quenching ability. This is an inexpensive process and can be used for small or large scale production. But water should not chemically react with metals or alloys used. 2. Gas atomization: Here instead of water, high velocity argon, nitrogen and helium gas jets are used. The molten metal is disintegrated and collected as atomized powder in a water bath. Fluidized bed cooling is used when certain powder characteristics are required. 3. Vacuum atomization: In this method, when a molten metal supersaturated with a gas under pressure is suddenly exposed into vacuum, the gas coming from metal solution expands, causing atomization of the metal stream. This process gives very high purity powder. Usually hydrogen is used as gas. Hydrogen and argon mixture can also be used. 4. Centrifugal atomization: In this method, one end of the metal bar is heated and melted by bringing it into contact with a non-consumable tungsten electrode, while rotating it longitudinally at very high speeds. The centrifugal force created causes the metal drops to be thrown off outwards. This will then be solidified as spherical shaped particles inside an evacuated chamber. Titanium powder can be made using this technique. 5. Rotating disk atomization: Impinging of a stream of molten metal on to the surface of rapidly spinning disk. This causes mechanical atomization of metal stream and causes the droplets to be thrown off the edges of the disk. The particles are spherical in shape and their size decreases with increasing disk speed. 6. Ultrarapid solidification processes: A solidification rate of 1000C/s is achieved in this process. This results in enhanced chemical homogeneity, formation of metastable crystalline phases, amorphous materials. Atomization Unit Melting and superheating facility: Standard melting furnaces can be used for producing the liquid metal. This is usually done by air melting, inert gas or vacuum induction melting. Complex alloys that are susceptible to contamination are melted in vacuum induction furnaces. The metal is transferred to a tundish, which serves as reservoir for molten metal. Atomization chamber: An atomization nozzle system is necessary. The nozzle that controls the size and shape of the metal stream if fixed at the bottom of the atomizing chamber. In order to avoid oxidation of powders, the tank is purged with inert gas like nitrogen. Powder collection tank: The powders are collected in tank. It could be dry collection or wet collection. In dry collection, the powder particles solidify before reaching the bottom of the tank. In wet collection, powder particles collected in the bottom of the water tank. The tank has to be cooled extremely if used for large scale production. During operation, the atomization unit is kept evacuated to 10-3 mm of Hg, tested for leak and filled with argon gas. Atomization Process Parameters 1. Effect of pressure of metal head: r = a + b√h; r – rate of atomization 2. Effect of atomizing medium pressure: r = a√p + b; Increase in air pressure increases the fineness of powder up to a limit, after which no increase is seen 3. Molten metal temperature: As temperature increases, both surface tension and viscosity decrease; so available energy can efficiently disintegrate the metal stream producing fine powders than at lower temperature; Temperature effect on particle shape is dependent on particle temperature at the instant of formation and time interval between formation of the particle and its solidification; Temperature increase will reduce surface tension and hence formation of spherical particle is minimal; however spherical particles can still be formed if the disintegrated particles remain as liquid for longer times. 4. Orifice area: negligible effect 5. Molten metal properties: Iron and Cu powder => fine spherical size; Pb, Sn => irregular shape powder; Al powders => irregular shape even at high surface tension (oxidation effect) Physical &Chemical Process The most common chemical powder treatments involve oxide reduction precipitate form solution and thermal decomposition. The powder produced can have a great variation in properties and yet have closely controlled particle size and shape. 1. Condensation: This method is used especially to produce a fine powder of Zinc of performed by evaporating and condensing of the respective material in a vacuum cylinder filled with a low pressure of an inert gass, the particle fog condenses preferably on cooled substrates that are implemented into a container. The particles build up a spherical powder layer on the cooling wall and can be removed continuously by a scraper. 2. Precipitation :The principal of precipitating a metal from its aqueous solution by the addition of a less noble metal which is higher in the electromotive series has been applied in numerous metallurgical processes. Ag powder is produced in quantity from its nitrate solution by adding copper or iron according to the reaction: AgNO3 + Fe FeNO3 + Ag This method is used for producing metal powders of Ag, Sn, Pt and iron particles coated with copper. Precipitation is also synonymous with the term electrolytic precipitation to coat metals with a corrosion resistance film. The produced powder take the form of spongy mass which crashed into a hard and brittle powder. 3. Carbonyl Method (Gaseous or Pyrolysis method) The carbonyl method is one of the most widely used methods of chemical deposition of the group of thermally decomposed powders, those produced by thermal decomposition of carbonyls are the most important. Both iron and nickel are produced by decomposition of the respective carbonyls. Carbonyls are obtained by passing carbon monoxide over spongy metal at specific temperatures and pressures according to the reaction: Fe + 5 CO → Fe(CO)5 This reaction can be controlled by changing temperature and pressure so the following reaction will take place: Fe + (CO)5 → Fe powder +5CO The carbonyl process is used for manufacturing powders of non- ferrous metals of VI, VII and VIII groups of the Periodic Table (nickel, partly tungsten, molybdenum, cobalt and some platinum group metals). Characteristic features of this process are low raw material and energy consumption, versatility of raw materials, high yield and purity of powders produced, possibility of completely automated operation. The technique affords control of particle size, shape and structure. It makes possible the production of metallic films, coatings, filamentary crystals, as well as of composite materials. Processing Conditions. Carbonyls are obtained by passing carbon monoxide over spongy metal at specific temperatures and pressures. Iron pentacarbonyl, Fe(CO)5, is a liquid at room temperature, boiling at 103 °C (217 °F). Nickel tetracarbonyl, Ni(CO)4, boils at 43 °C (109 °F). When the pressure is reduced to 1 atm and the temperature is raised correspondingly, both of these carbonyls decompose to re-form the metal and carbon monoxide. The latter is recycled to form more carbonyl and to continue the process. These reactions are expressed as follows: Fe + 5CO Fe (CO)5 Ni + 4CO Ni (CO)4 Powder is produced by boiling the carbonyls in heated vessels at atmospheric pressure under conditions that allow the vapors to decompose within the heated space and not on the sides of the container. The powder is collected and sieved and may be milled, followed by an anneal in hydrogen. The chemical purity of the powders can be very high (over 99.5%), with the principal impurities being carbon, nitrogen, and oxygen. Particle size can be controlled very closely. Iron carbonyl powder is usually spherical in shape and very fine (less than 10 Micrometer) used for the production of magnet cores, while the nickel powder is usually quite irregular in shape, porous, and fine but the production process is of high cost. Another examples such as: Ni (CO)4 → Ni powder +4CO Mo (CO)6 → Mo powder +6 CO W(CO)6 → W powder +5CO Co (CO)8 → Co powder +5CO Iron pentacarbonyl | Fe(CO)5 4. Precipitation :The principal of precipitating a metal from its aqueous solution by the addition of a less noble metal which is higher in the electromotive series has been applied in numerous metallurgical processes. Ag powder is produced in quantity from its nitrate solution by adding copper or iron according to the reaction: AgNO3 + Fe FeNO3 + Ag This method is used for producing metal powders of Ag, Sn, Pt and iron particles coated with copper. Precipitation is also synonymous with the term electrolytic precipitation to coat metals with a corrosion resistance film. The produced powder take the form of spongy mass which crashed into a hard and brittle powder. 4. Oxide reduction: Reduction of compounds particularly oxides by the use of reducing agent in the form of either solid or gas according to the reaction: Fe2O3+ 2C → 3Fe +2CO2 This is a convenient, economical and extremely flexible method for controlling the properties of the product regarding size, shape and porosity over a wide range. The production of iron, copper, tungsten, and molybdenum powders from their respective oxides are well-established commercial processes. On a smaller scale, oxide reduction is also used for production of cobalt and nickel powders. This process yields extremely fine powders with irregularly shape particles, good compactibility (high green strength) and sinterability, low final porosity of such powders and relatively low cost. 5. Electrolytic deposition: In this method, the processing conditions are so chosen that metals of high purity are precipitated from aqueous solution on the cathode of an electrolytic cell. This method is mainly used for producing copper, iron powders. This method is also used for producing zinc, tin, nickel, cadmium, antimony, silver, lead, beryllium powders. By choosing suitable conditions such as electrolytic composition and concentration temp and current density many metals can be deposited in a spungy or powdered state further processing is required which yields high purity and high density powders. Cu is the primary mental produced. Copper powder => Solution containing copper sulphate and sulphuric acid; crude copper as anode. Reaction: at anode: Cu -> Cu+ + e-; at cathode: Cu+ + e- ->Cu. Iron powder => anode is low carbon steel; cathode is stainless steel. The iron powder deposits are subsequently pulverized by milling in hammer mill. The milled powders are annealed in hydrogen atmosphere to make them soft. Mg powder => electrode position from a purified magnesium sulphate electrolyte using insoluble lead anodes and stainless steel cathodes. Powders of thorium, tantalum, vanadium => fused salt electrolysis is carried out at a temperature below melting point of the metal. Here deposition will occur in the form of small crystals with dendritic shape. In this method, final deposition occurs in three ways, 1. A hard brittle layer of pure metal which is subsequently milled to obtain powder (eg. iron powder) 2. A soft, spongy substance which is loosely adherent and easily removed by scrubbing 3. A direct powder deposit from the electrolyte that collects at the bottom of the cell. NOTE: Factors promoting powder deposits are, high current density, low metal concentration, pH of the bath, low temperature, high viscosity, circulation of electrolyte to avoid of convection. Advantages: Powders of high purity with excellent sinterability Wide range of powder quality can be produced by altering bath composition. Disadvantages: Time consuming process; Pollution of work place because of toxic chemicals; Waste disposal is another issue; Cost involved in oxidation of powders and hence they should be washed thoroughly 6. Precipitation from aqueous solutions and fused salts Metal powders (Reactive powder produce) can be produced by electro deposition from aqueous solutions and fused salts. This method is reversed adaption of electroplating. A hard and brittle mass is deposited which is subsequently ground to powder having a dendritic shape. This technique is mainly employed for commercial production of iron , copper, Thorium & Beryllium) Thank You For Your Attention