MAM Week-5 PDF
Document Details
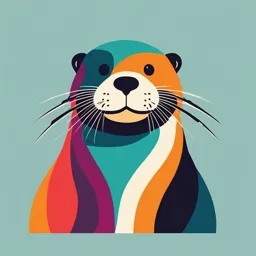
Uploaded by LuminousAllegory9463
IIT Kanpur
Dr. J. Ramkumar
Tags
Related
- DM308 Production Techniques 2 Lecture 1 – Additive Manufacturing (Part 2) PDF
- Introduction to Metal Additive Manufacturing PDF
- Metal Additive Manufacturing (MAM) Week-3 PDF
- Metal Additive Manufacturing Powder/Wire Fed Systems PDF
- MAM Week-5_merged PDF
- Additive Manufacturing of Metal Matrix Composites PDF
Summary
This document provides information about common defects in additive manufacturing (AM), specifically in metal 3D printing. It details various types of defects, such as elongated pores, gas pores, and unfused powder, along with their potential causes.
Full Transcript
EL PT N Dr. J. Ramkumar Professor Department of Mechanical Engineering and Design IIT Kanpur ▪ Defects in AM Printed Parts EL ▪ Need of Post Processing ▪ Need for Surface Finishing PT ▪ Common Post Processing for...
EL PT N Dr. J. Ramkumar Professor Department of Mechanical Engineering and Design IIT Kanpur ▪ Defects in AM Printed Parts EL ▪ Need of Post Processing ▪ Need for Surface Finishing PT ▪ Common Post Processing for MAM N 1. Elongated, non-spherical pores EL 2. Gas Pores 3. Unfused powder 4. 5. 6. Balling effect Cracking Warping PT N 7. Delamination 8. Swelling 1. ELONGATED, NON-SPHERICAL PORES EL ▪ Processed induced defect ▪ Due to an inefficient melting regime ▪ Too little energy input with respect to hatching distance or layer thickness 2. GAS PORES PT ▪ Spatter and fumes ejection (too much energy input) ▪ Due to powder surface chemistry modification N ▪ Due to trapped gas in particles that are released during melting and locked in during solidification POROSITY EL A. Entrapped gas porosity B. Incomplete C. melting induced porosity Lack of fusion with PT N unmelted particles inside large irregular pores D. Cracks Sola, Antonella, and Alireza Nouri. "Microstructural porosity in additive manufacturing: The formation and detection of pores in metal parts fabricated by powder bed fusion." Journal of Advanced Manufacturing and Processing 1.3 (2019): e10021. 3.UNFUSED POWDER EL ▪ Processed induced defect ▪ Due to insufficient melting or overlap between successive layers or adjacent melted tracks PT N W. J. Sames, F. A. List, S. Pannala, R. R. Dehoff & S. S. Babu (2016): The metallurgy and processing science of metal additive manufacturing, International Materials Reviews http://dx.doi.org/10.1080/09506608.2015.1116649 4. Balling EL ▪ Solidification of melted material into spheres ▪ Due to lack of wettability with the previous layer, driven by surface tension ▪ Directly related to melt pool characteristics PT N W. J. Sames, F. A. List, S. Pannala, R. R. Dehoff & S. S. Babu (2016): The metallurgy and processing science of metal additive manufacturing, International Materials Reviews http://dx.doi.org/10.1080/09506608.2015.1116649 5. CRACKING EL ▪ Due to solidification cracking or grain boundary cracking or other macroscopic effects like ▪ Residual stress ▪ Surface roughness PT N https://www.metal-am.com/articles/how-residual-stress-can-cause-major-build-failures-in-3d-printing/ 6. WARPING EL ▪ Occurs between two layers or at the boundary between support and part layer (curling) ▪ Occurs when the build is stopped and re-started or at the boundary between substrate and first layers PT N Di Wang, Yongqiang Yang, Ziheng Yi, Xubin Su, Research on the fabricating quality optimization of the overhanging surface in SLM process, The International Journal of Advanced Manufacturing Technology April 2013, Volume 65, Issue 9, pp 1471-1484 https://ebrary.net/132919/engineering/towards_methods_avoiding_distortion_precision_parts 7. DELAMINATION EL ▪ Separation of successive layers ▪ Due to inappropriate melting overlap with previous underlying solidified powder or incomplete particles melting. ▪ Macroscopic effects cannot be repaired by post-processing PT N W. J. Sames, F. A. List, S. Pannala, R. R. Dehoff & S. S. Babu (2016): The metallurgy and processing science of metal additive manufacturing, International Materials Reviews http://dx.doi.org/10.1080/09506608.2015.1116649 8. SWELLING EL ▪ Similar to the humping phenomenon in welding ▪ Occurs due to surface tension effects related to the melt pool geometry PT N A. Manriquez-Frayre and D. L. Bourell: ‘Selective laser sintering of binary metallic powder’, in ‘Solid freeform fabrication symposium’, Austin, TX, 1990, 99–106. N PT EL ▪ AM has the potential to transform the component design in high-value and/or low-volume manufacturing. EL ▪ AM applications range from topology optimization for fatigue performance and light-weighting in aerospace to bespoke one- medicine. PT off components for patient implants and operation guides in ▪ Surface topographies may alter functional requirements for these applications' components. N Post processing can be divided into three categories: EL 1. Process-inherent process i. De-powdering iii. Stress relief PT ii. Support removal 2. Mechanical properties N i. Heat treatment (affecting hardness, ductility, and fatigue life) ii. Surface treatment and finishes ( maximize visual appeal) 3. Visual inspection ▪ POWDER REMOVAL EL ▪ Achieved with standard cleaning procedures ▪ Often unused powder is recycled and reused PT N https://www.autodesk.com/products/fusion-360/blog/democratization-metal-additive-manufacturing-part-1/ 1 ▪ SUPPORT REMOVAL EL 1. Cutting 2 2. Band Saw 3. Drilling 5 4. CNC Machining 5. Wire EDM 4 PT 3 N https://www.mmsonline.com/articles/buying-a-wire-edm-dielectric-fluid-and-machine-maintenance https://www.additivemanufacturing.media/articles/postprocessing-steps-and-costs-for-metal-3d-printing https://caddetailsblog.com/post/how-cnc-technology-is-changing-architecture-today https://www.assemblymag.com/articles/94886-innovations-in-metal-3d-printing HEAT TREATMENT EL ▪ Hot Isostatic Pressing (HIP) ▪ Densifies material and removes the defect ▪ Improves mechanical properties PT N ▪ Increase reliability and performance ▪ High Efficiency and lower production cost https://www.azom.com/article.aspx?ArticleID=5769 ANNEALING EL ▪ Increased stress relief ▪ Improved mechanical properties PT N https://www.fivesgroup.com/ru/induction/thermal-treatment/annealing SURFACE FINISHING EL ▪ Last Touch PT ▪ Smoothening and Polishing of surface ▪ Optimize aesthetics N ▪ Reduce roughness to a minimum (if required) COMMON SURFACE FINISHING OPERATION EL ▪ Bead blasting ▪ Anodizing PT ▪ Electroplating or metal plating N ▪ Laser polishing BEAD BLASTING EL PT N https://www.vapormatt.com/our-technology/what-wet-blasting BEAD BLASTING EL PT N https://www.comcoinc.com/finishing_3d_printed_parts/ ANODIZING EL ▪ The part is dipped in an electrolytic solution and endowed with a protective anodic layer that increases hardness and resistance to corrosion, PT wear, and electrical conductivity. ▪ ELECTROPLATING N ▪ Similar to anodizing ▪ Metal coating ▪ Prevents corrosion ANODIZING EL PT N https://pubs.acs.org/doi/10.1021/acs.nanolett.1c02815 LASER POLISHING EL PT N Basha, S.M., Bhuyan, M., Basha, M.M., Venkaiah, N. and Sankar, M.R., 2020. Laser polishing of 3D printed metallic components: a review on surface integrity. Materials Today: Proceedings, 26, pp.2047-2054. LASER POLISHING EL PT N Basha, S.M., Bhuyan, M., Basha, M.M., Venkaiah, N. and Sankar, M.R., 2020. Laser polishing of 3D printed metallic components: a review on surface integrity. Materials Today: Proceedings, 26, pp.2047-2054. LASER POLISHING EL PT N Bordatchev, E.V., 2020, October. Advanced Capabilities in Laser Surface Polishing and Functionalization at the National Research Council of Canada. In Laser Applications Conference (pp. LW4B-1). Optical Society of America. ▪ Name the common defects in metal printed parts EL ▪ What is the importance of post processing? ▪ What are major post processing techniques to remove defects? PT N ▪ Make a chart of defects and their cause in MAM EL ▪ Provide solutions for MAM defects PT N N PT EL EL PT N Dr. J. Ramkumar Professor Department of Mechanical Engineering and Design IIT Kanpur ▪ AM process parameters EL ▪ Beam Scanning Strategies and Parameters for PBF and DED ▪ Powder Properties for PBF, DED, and BJ PT ▪ Methods of Powder Particles Production ▪ Wire Properties for DED ▪ Ambient Parameters for PBF and DED N ▪ Geometry-Specific Parameters (PBF) ▪ Support Structures for PBF ▪ AM process parameters can be divided into three EL categories. 1. Input parameters 2. Process physics PT 3. Output parameters N ▪ Material: powder rheology, chemical composition EL ▪ Process: beam power, layer thickness ▪ Ambient: build chamber temperature, shield gas type etc. PT ▪ Machine attributes: motion system accuracy, maximum velocity, ▪ Some factors, like laser strength, are changeable, while others, like shield gas, are usually constant. N ▪ This section explains how these parameters affect output parameters. ▪ Some parameters are exclusive to one AM method, while others are common. N PT EL ▪ Printing-related:thermodynamic phenomena, solidification, binder EL imbibition, etc. ▪ These phenomena influence the transition of raw materials (powder, selection. ▪ Measurement and PT wire, paste) to solid geometry according to input parameter control N technologies are being developed and implemented into commercial AM machines to monitor process physics in situ and change input settings. ▪ After manufacture, destructive and non-destructive tests are used to EL measure the bulk qualities of the printed item. ▪ These include geometrical ▪ ASTM PT (tolerances, distortion), mechanical, metallurgical, and other qualities. develops new AM testing standards. N ▪ Obtaining reproducible density, hardness, surface roughness, and geometrical integrity is a difficulty for the AM industry MELT POOL DIMENSIONAL CHARACTERISTICS EL PT N ▪ h is the track height, w is the track or melt pool width, ▪ θ is the wetting angle, and b is the melt pool depth (without the track height), representing the thickness of substrate melted during the DED or LPBF and added to the track region. ▪ In PBF, the melt pool depth is considered b, whereas, in DED, h+b is considered the melt pool height DILUTION EL ▪ According to the previous fig it can be defined as PT ▪ Alternatively, dilution may be defined as the percentage of the surface layer’s total volume contributed by the substrate’s melting N where ρc is the density of melted powder alloy (kg/m3 ), ρs is the density of substrate material (kg/m3 ), Xs + c is the weight percentage of element X in the total surface of the clad region (%), Xc is the weight percentage of element X in the powder alloy (%), and Xs is the weight percentage of element X in the substrate ▪ Specific energy: The specific energy is indicative of input energy from the surface irradiated by the laser beam. EL ▪ Volumetric energy density (VED): It is the amount of thermal PT energy received by each unit of volume. ▪ The spot size d provides an alternative definition more similar N to specific energy P is laser power (W), v is scanning speed (m/s), s is hatch distance (m), and l is layer thickness (m) ▪ Parameters such as: EL ▪ beam scan velocity, ▪ hatch distance, ▪ scan pattern, ▪ pre-scanning, and ▪ rescanning PT are considered beam scanning parameters and highly N affect the microstructure, surface quality, internal porosity, and mechanical properties of AM-made parts. ▪ Different scanning patterns have been adopted to control residual stresses and part distortions COMMON SCANNING PATTERNS EL ▪ Line scanning is the most basic scanning strategy, which is achieved by following simple rectilinear scanning paths for all the layers either in the x-direction (0 ) or y-direction (90 ). ▪ Changing the scanning angle (e.g., 30, 45, 67, …) PT ▪ or adopting different scanning angles for successive layers in a rotational manner (e.g., 0, 90, 0, … or 45, 135, 45, …) are other options commonly practiced by AM professionals. N ▪ Among these angles, many machine developers use 67 between the layers. ▪ Island or chessboard scanning is another popular approach that divides the print layer into multiple squares or randomly shaped smaller islands with different scanning types or angles assigned to each. ▪ In-out and out-in scanning strategies that include a spiral motion. ▪ COMMON SCANNING PATTERNS EL PT N ▪ Powder bed-based AM methods investigate material properties under two sub-categories: 1. Powder particle properties and EL 2. Bulk powder behavior. ▪ At the particle level, morphology, including ▪ Sphericity, PT ▪ Powder size distribution (PSD), ▪ Surface roughness, and N ▪ Skewness, determines ▪ Flowability, ▪ Beam/powder interaction, and ▪ Final part quality. ▪ In AM powder characterization, PSD and particle shape affect flowability. EL ▪ Chemical composition and microstructure of powder have PT been explored less. ▪ PSD determines PBF's lateral and vertical printing resolution N and surface quality. N PT EL ▪ Smaller particles improve print resolution and morphology. EL ▪ As particle size decreases, electrostatic interactions between particles rise, causing agglomeration and reducing powder flow. PT ▪ Rough surface, wide PSD, and low sphericity all increase interparticle friction and flowability. ▪ A powder system with larger spherical particles and a N smoother surface flows better. ▪ Coarser particles with uniform sizes create powder beds with limited packing efficiency, resulting in increased porosity. ▪ Ideal feedstock should be spherical with small enough particles to form high-density powder beds, EL ▪ but not so small that they won't flow correctly or become airborne and damage laser-optical systems or cause safety hazards. PT ▪ The particle size range should be large enough for smaller satellite particles to fill gaps between coarser particles and improve layer density, ▪ but not so wide as to limit flowability. N ▪ Powder bed-based systems prefer a particle size 100< µm. ▪ Powder flow qualities are usually reliant on the testing apparatus and experimental conditions. EL ▪ Different systems' powder flowability qualities aren't easily comparable. PT ▪ One method for characterizing powder flow characteristics for AM evaluates the powder's resistance to a rotating and vertically moving blade. N ▪ Higher blade energy means more cohesive powder. Cohesiveness reduces powder flowability. Relationship between particle and flow properties and flowability EL PT N S. Vock, B. Klöden, A. Kirchner, T. Weißgärber, and B. Kieback, “Powders for powder bed fusion: a review,” Prog. Addit. Manuf., vol. 4, pp. 383–397, Feb. 2019. Available: https://link.springer.com/content/pdf/10.1007/s40964-019-00 ▪ The powder bed's packing efficiency is affected by the bulk powder's compressibility. EL ▪ More cohesive powders create a less compacted powder bed. ▪ The feedstock should have high flowability and low cohesion so PT it fluidizes when sheared by the powder delivery mechanism. ▪ This is vital to producing a high-density item with a uniform, homogeneous layer. N ▪ A densely packed powder bed decreases thermal stress and manufacturing problems like warpage. ▪ Layer density affects BJ binder saturation and surface roughness. 1. Water atomization EL 2. Gas atomization 3. Plasma atomization 4. PT Centrifugal atomization N 1. WATER ATOMIZATION EL ▪ In this technique, water is sprayed at high pressure (500– 1500 bar) over melted metal to form micro-size particles. PT ▪ This technique has a high yield and large particle sizes up to 500 m at a cheaper cost. N ▪ The technique yields irregular-shaped particles and can't handle reactive compounds. ▪ The irregular shape of particles as well as the presence of satellites are two major challenges when it comes to AM adoption. EL PT N WATER ATOMIZATION Asgarian, Ali, et al. "Experimental and computational analysis of a water spray; application to molten metal atomization." Advances in powder metallurgy and particulate materials (Proceedings of the 2018 International Conference on Powder Metallurgy & Particulate Materials). 2018. 2. GAS ATOMIZATION EL ▪ In this process, a high-velocity gas impacts a stream of melted material, generating dispersal and particle creation. ▪ Gas pressure is 5–50 bar lower than water atomization. ▪ Gas-atomized particles have PT N spherical forms and small satellites up to 500 m. ▪ The high-throughput method works well with reactive materials. Qiu, Zhiwei. "Improving gas flow in gas atomization." (2021). Gas atomization 3. PLASMA ATOMIZATION EL ▪ Argon plasma torches melt and atomize feedstock into micro- droplets. ▪ The feedstock (wire or irregular particles) melts in >10 000 0C plasma to generate 200 µm droplets. PT N ▪ This method creates spherical particles with smaller size distribution. ▪ Costly process. Plasma atomization Baskoro, Ario Sunar, and Sugeng Supriadi. "Review on plasma atomizer technology for metal powder." MATEC Web of Conferences. Vol. 269. EDP Sciences, 2019. 4. CENTRIFUGAL ATOMIZATION EL ▪ Spinning discs interact with molten material to create micron-sized droplets. ▪ ▪ PT It makes fine powders. In an inert gas, it requires less energy than gas and water atomization. N ▪ The product has a lower oxide formation. ▪ Low yield and high system cost due to tungsten contamination. ▪ Plasma rotating electrode atomization produces exceptionally pure particles. EL PT N Centrifugal atomization Sungkhaphaitoon, Phairote, Sirikul Wisutmethangoon, and Thawatchai Plookphol. "Influence of process parameters on zinc powder produced by centrifugal atomisation." Materials Research 20 (2017): 718-724. ▪ Wire Fed DED techniques with laser or electron beam energy sources utilize metal wire filaments. EL ▪ Similar to powders, the wires' chemical composition, and dimensional uniformity might affect the melt pool. parameters. PT ▪ Wire feed rate and orientation are significant wire-fed process ▪ Wire feed rate is how fast wire is supplied from the nozzle into N the deposition region. ▪ In Wire Fed -EB-DED, the wire feed rate restrictions are set by the welding power since an extremely high feed rate results in EL incompletely melted material deposition. ▪ Feed rate, welding speed, and power determine deposit process parameters. PT dimensions. Controlling this geometry requires knowledge of ▪ Increasing power widens the deposit while decreasing its height. N ▪ Welding speed decreases width and increases height. ▪ It is the wire feed rate to welding speed ratio that affects deposition area. Influence of increasing process parameters on dimensions of a single bead produced by EB-DED EL PT N ▪ The layer thickness of a PBF or BJ powder bed. DED layer EL thickness is track height. ▪ This parameter is important for powder bed-based procedures. ▪ Layer thickness affects resolution, surface quality, and build time. PT N ▪ On curved or non-vertical surfaces, thicker layers cause the staircase effect. ▪ Smaller layer thickness can Staircase effect better resemble the part's surface but slows production. H. Brooks, A. Rennie, T. Abram, J. McGovern, and F. Caron, “Variable fused deposition modelling: analysis of benefits, concept design and tool path generation,” in: 5th International Conference on Advanced Research in Virtual and Rapid Prototyping, pp. 511–517, 2011. ▪ The minimum layer thickness is governed by EL 1. the powder bed's vertical movement precision and 2. the feed material's largest particle. PT ▪ PBF layer thickness varies on beam power, shape, and speed. ▪ The maximum layer thickness should be less than the height of the melt pool formed by the parameters; otherwise, some particles will remain unfused and the layers won't fuse entirely. N ▪ The hatch distance determines the maximum value. EL PT ▪ The maximum layer thickness (tmax) is equal to the distance between the surface and the lowest point of overlap between two adjacent melt pools 𝑀𝑑0 N ▪ The larger the hatch distance, the smaller the layer thickness needs to be to avoid having unfused particles. ▪ For the BJ process, a layer thickness must be chosen to guarantee binders moisten the entire layer. ▪ This depends on droplet nozzle spacing, printhead velocity, jetting frequency, wetting angle, and binder viscosity. J. P. Oliveira, A. D. LaLonde, and J. Ma, “Processing parameters in laser powder bed fusion metal additive manufacturing,” Mater. Des., vol. 193, p. 108762, 2020 ▪ The considerable energy required for metal processing in AM systems requires more monitoring and control of EL environmental conditions. ▪ Build chamber temperature and shield gas system are the most PT crucial ambient characteristics affecting printed items. ▪ The distribution of process temperature at many levels, such as the build chamber, powder bed surface, powder bed core, and local beam/powder interaction area, affects the quality of N printed components, melt pool size and type, etc. ▪ Build chamber temperature affects the microstructure and mechanical characteristics of metal components. ▪ Temperature cannot be investigated as a separate parameter since it interacts with others, especially beam source power. EL ▪ Low powder bed temperature and high power cause distortion from residual stress concentration. PT ▪ Increasing the powder bed temperature helps eliminate this issue at the expense of geometrical integrity as the loose powder particles fuse together, especially near the build part, causing part expansion. This reduces powder recycling. N ▪ A high powder bed temperature gradient can initiate and accelerate crack formation. ▪ Given the relevance of ambient temperature in process reliability and repeatability, sensors and metrology equipment EL like as infrared cameras, thermo-couple embedded build beds, etc. must be included in PBF machines for in-situ monitoring of the powder bed temperature profile. PT ▪ Shielding gas blocks the contact of the melt pool with reactive gases and removes spatters. ▪ The type of shielding gas and its flow affect melt pool size, N homogeneity, porosity, and mechanical performance. ▪ Varied shielding gases have different thermal conductivities, which affect the heat transmission dynamics surrounding the melt pool. ▪ Nitrogen's increased thermal conductivity results in a lower powder bed surface temperature and faster cooling rate than EL argon, altering part microstructure and mechanical properties. ▪ A homogeneous gas flow is necessary for removing print by- melt pool. PT products that could impact laser-material interaction near the ▪ EB-based technologies require a high vacuum to prevent electron beam dispersion. N ▪ To prevent electrical charges from forming in powders during melting, a low-pressure inert gas, usually helium, is supplied. After printing, helium is used to cool the component. ▪ Beam strength, velocity, and scanning approach are not static throughout the construct parts' volume. EL ▪ They depend on the part's geometry and position. ▪ In commercial systems, changing parameters at contours, up- PT skin, down-skin, and core is typical. N ▪ Contour is the outer edge of each layer, which corresponds to the part's interior and external surfaces. One or two contours EL are optional. ▪ Different process settings can be selected for printing a part's up-skin and down-skin, which solely include loose powder. PT Between is the part’s center. ▪ For complex geometries, each layer can have up-skin, core, and down-skin regions. N ▪ Commercial devices calculate overlap to guarantee appropriate bonding. EL PT N G. Nicoletto, “Directional and notch effects on the fatigue behavior of as-built DMLS Ti6Al4V,” Int. J. Fatigue, vol. 106, pp. 124–131, 2018. ▪ Overhanging, hole, and bridge features necessitate support structures for PBF. EL ▪ Complex AM components require various support structures. ▪ Post-processing procedures (e.g., snapping, EDM) are used to remove them. PT ▪ This wastes and costs money. ▪ Optimal process settings are used to print overhanging N features without support for particular materials. ▪ BJ and DED don't need support structures. EL ▪ In DED, support structures can be avoided through trajectory optimization with a high-degree-of-freedom motion system. ▪ Support structures dissipate heat. PT ▪ PBF supports facilitate heat conduction to prevent residual stress concentration. ▪ Different densities and dispersion of support structures are N added between the construction plate and the main part. This interface is a high-stress area. ▪ The concept of supports structures, three different support shapes, and densities EL a) Medium density b) Low density c) High density. PT N ▪ Major process parameters affecting print quality for different AM processes. EL ▪ Common scanning strategies used in MAM ▪ Methods for manufacturing metal powders and powder PT properties for MAM ▪ Wire properties in DED AM technique ▪ Ambient properties and geometric specific properties for N printing ▪ Support structures in MAM. ▪ Make a fishbone diagram for different process parameters in the PBF process. EL ▪ Make a comparison of powder properties with wire properties affecting print quality. PT N N PT EL EL PT N Dr. J. Ramkumar Professor Department of Mechanical Engineering and Design IIT Kanpur ▪ Mechanical properties of AM printed parts EL ▪ Hardness of AM printed alloys ▪ Tensile and static strength of AM printed alloys PT ▪ Fatigue Behavior of AM-Manufactured Alloys ▪ Common defects in AM printed alloys N In some materials and process parameter combinations, AM- made parts now have mechanical properties comparable to conventionally made parts. EL Porosity affects cracking and mechanical properties. A density above 99.5% is the first priority for AM technique PT optimization, especially if the AM-made parts are used in critical mission applications like jet engine components, etc. In addition to other effects, AM product density depends on N the energy volume given to the material. Insufficient energy flux can cause unmelted material, resulting in irregular voids that reduce density. EL PT N https://www.azom.com/article.aspx?ArticleID=17901 An excessive energy input may cause melt pool dynamics EL instability, which may enter a keyhole mode that decreases density due to trapped gas pores. These spherical pores are likely caused by trapped gasses PT (inter gas and material evaporation) due to melt pool instabilities and keyhole phenomena. The higher density achievable with AM for different materials suggests a discrete optimization guideline for AM parameters N to achieve optimal volume energy. Optimizing parameters to maximize density by reducing porosities is done because pore size and distribution affect mechanical properties. EL PT N https://www.insidemetaladditivemanufacturing.com/blog/visual-guide-to-the-most-common-defects-in-powder-bed-fusion-technology EL 1. Hardness 2. Tensile Strength and Static Strength 3. PT Fatigue Behavior of AM-Manufactured Alloys N AM product hardness shows mechanical strength. EL The microhardness of AM-processed alloys follows the Hall- Petch relationship. The microstructure coarsens with a larger cross-sectional area, PT causing the hardness to drop. This microstructure coarsening is due to more thermal input in a larger area, which slows cooling. N The thermal history of each layer affects the layer's hardness. Using different process parameters for the cross-sectional area may improve hardness heterogeneity. HARDNESS OF AM-PROCESSED FERROUS ALLOYS Hardness development in AM-processed ferrous alloys is EL influenced by process input parameters. For multilayer deposited steels, micro-hardness drops from the initially deposited layers and then increases. PT This happens when previous layers are repeatedly heated and partially annealed. N This inconsistency is due to the liquid melt's time-dependent cooling rate and the middle area's slow solidification rate. Top and bottom AM parts have higher hardness than the middle. In low alloy steels, such as 41XX series steels, alloying components, and carbon effect phase formation and hardness. LOW ALLOY STEEL FOR AM EL PT N https://www.gknpm.com/en/our-businesses/gkn-additive/low-alloy-steels-for-additive-manufacturing/ HARDNESS OF AM-PROCESSED FERROUS ALLOYS EL Rapid cooling in AM creates hard martensite phases in high- carbon steel, which increases hardness. Plain carbon and low alloy steels are not commonly used in PT AM applications due to higher manufacturing costs. Most AM steels are tool steels with high strength and wear resistance for repair purposes. N M2, P20, and H13 tool steels are stronger because martensite forms at lower cooling rates. These alloys contain more carbon, which accelerates carbide formation and boosts strength and hardness. N PT EL HARDNESS OF AM-PROCESSED FERROUS ALLOYS Structures of AM-processed tool steels show martensitic EL matrix with carbide precipitates and austenite. Post-AM heat treatment improves ductility and toughness through tempered martensitic structure with carbide precipitates. PT Austenitic stainless steels don't have secondary phases or solid-state phase transformations. N The finer solidification structure and alloy's chemical composition determine strength and hardness. The hardness of LDED-processed austenitic stainless steels (316, 316L) as a function of Secondary Dendritic Arm Spacing (SDAS). HARDNESS OF AM-PROCESSED FERROUS ALLOYS Both alloys have identical dendritic structures and chemical EL compositions. A slight difference in carbon content (316L