Optics PDF
Document Details
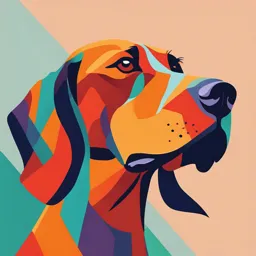
Uploaded by CrispPrairie
Tags
Summary
This document provides an overview of optics, covering topics such as reflection of light, the electromagnetic spectrum, and the law of reflection. It explains how different types of electromagnetic radiation work and their various uses.
Full Transcript
OPTICS Reflection of Light Almost all surfaces reflect some of the light that strikes them. A still water of a lake reflects almost all of the light that strikes it. The reflected light forms an image of nearby objects. An image is a copy of an object that is formed by reflected or refracted light....
OPTICS Reflection of Light Almost all surfaces reflect some of the light that strikes them. A still water of a lake reflects almost all of the light that strikes it. The reflected light forms an image of nearby objects. An image is a copy of an object that is formed by reflected or refracted light. Regular and Diffuse Reflection If a surface is extremely smooth, like very still water, then an image formed by reflection is sharp and clear. This is called regular reflection. If the surface is even slightly rough, an image may not form, or if there is an image, it is blurry or fuzzy. This is called diffuse reflection. The EM spectrum Electromagnetic waves are categorized according to their frequency f or, equivalently, according to their wavelength λ = c/f. Visible light has a wavelength range from ~400 nm to ~700 nm. Violet light has a wavelength of ~400 nm, and a frequency of ~7.5*1014 Hz. Red light has a wavelength of ~700 nm, and a frequency of ~4.3*1014 Hz. Visible light makes up just a small part of the full electromagnetic spectrum. Electromagnetic waves with shorter wavelengths and higher frequencies include ultraviolet light, X-rays, and gamma rays. Electromagnetic waves with longer wavelengths and lower frequencies include infrared light, microwaves, and radio and television waves. Type of Radiation gamma-rays X-rays ultraviolet visible near-infrared infrared microwaves radio waves image Frequency Range (Hz) 1020 - 1024 1017 - 1020 1015 - 1017 4 - 7.5*1014 1*1014 - 4*1014 1013 - 1014 3*1011 - 1013 < 3*1011 Wavelength Range < 10-12 m 1 nm - 1 pm 400 nm - 1 nm 750 nm - 400 nm 2.5 μm - 750 nm 25 μm - 2.5 μm 1 mm - 25 μm > 1 mm What can we learn by analyzing the EM spectrum emitted by a source? The velocities of particles with thermal energy are changing almost all the time. The particles are accelerating. Accelerating charged particles produce electromagnetic radiation. The power radiated is proportional to the square of the acceleration. Higher rates of velocity change result in higher frequency (shorter wavelength) radiation. The observed intensity of thermal radiation emitted by as a function of wavelength can be described by the Planck Radiation Law (Physics 221). black-body radiationThe Planck Radiation Law gives the intensity of radiation as a function of wavelength for a fixed temperature. The Planck law gives a continuous distribution, which peaks at some wavelength. The peak shifts to shorter wavelengths for higher temperatures, and the area under the curve grows rapidly with increasing temperature. The diagram below shows the intensity distribution predicted by the Plank law in J/(m2s) for blackbodies at various temperature. By observing the continuous distribution of the thermal radiation emitted by an object, we can learn its temperature. When light passes through or reflects or scatters of matter, it interacts with the atoms and molecules. Atoms and molecules have characteristic resonance frequencies. The preferentially interact with light waves of exactly those frequencies. When excited in collisions, atoms and molecules emit light with a set of characteristic frequencies. This results in a line spectrum. Only light with a discrete set of wavelengths is produced and the spectrum is not continuous, but consist of a set of emission lines. That set characterizes the atoms and molecules which produced it and can be used to identify those atoms and molecules and their environment. When light with a continuous distribution of wavelengths passes through a low-density material, the atoms and molecules of the material absorb light waves with the same set of characteristic frequencies that appear in their emission spectrum. This produces an absorption spectrum, a nearly continuous spectrum with missing lines. The absorption spectrum can also be used to identify those atoms and molecules and their environment. Law of Reflection One thing is true of both regular and diffuse reflection. The angle at which the reflected rays bounce off the surface is equal to the angle at which the incident rays strike the surface. This is the law of reflection, and it applies to the reflection of all light. The Electromagnetic Spectrum The electromagnetic (EM) spectrum is the range of all types of EM radiation. Radiation is energy that travels and spreads out as it goes – the visible light that comes from a lamp in your house and the radio waves that come from a radio station are two types of electromagnetic radiation. The other types of EM radiation that make up the electromagnetic spectrum are microwaves, infrared light, ultraviolet light, X-rays and gamma-rays. You know more about the electromagnetic spectrum than you may think. The image below shows where you might encounter each portion of the EM spectrum in your day-to-day life. Radio: Your radio captures radio waves emitted by radio stations, bringing your favorite tunes. Radio waves are also emitted by stars and gases in space. Microwave: Microwave radiation will cook your popcorn in just a few minutes, but is also used by astronomers to learn about the structure of nearby galaxies. Infrared: Night vision goggles pick up the infrared light emitted by our skin and objects with heat. In space, infrared light helps us map the dust between stars. Visible: Our eyes detect visible light. Fireflies, light bulbs, and stars all emit visible light. Ultraviolet: Ultraviolet radiation is emitted by the Sun and are the reason skin tans and burns. "Hot" objects in space emit UV radiation as well. X-ray: A dentist uses X-rays to image your teeth, and airport security uses them to see through your bag. Hot gases in the Universe also emit X-rays. Gamma ray: Doctors use gamma-ray imaging to see inside your body. The biggest gamma-ray generator of all is the Universe. Vocabulary Aperture: Describes how much light will be intercepted by the mirror. Center of curvature: Useful when locating images, the center of the imaginary sphere upon which a curved mirror rests. The flatter the mirror, the farther away the center of curvature. Concave mirror: Converging mirror which forms either real or virtual images which may be magnified. Convex mirror: Diverging mirror which forms virtual images which may be magnified. Focal length: The distance from the lens or mirror to the principal focus. Principal axis: The main line drawn through the center of the mirror or lens upon which information such as center of curvature or principal focus is given. Principal focus: The point where light rays parallel and close to the principal axis converge or appear to diverge. Ray diagram: A tracing of the light rays to show where the image forms after being reflected off a mirror or refracted through a lens. Real image: An image that can be projected onto a screen at its location, inverted relative to the object, and can be magnified. Secondary axis: Any line drawn through the center of curvature to the mirror or lens. Vertex: The center of a lens or mirror. Virtual image: An image that cannot be projected onto a screen, is not inverted relative to an object, and can be magnified. The Eye You may be asked several questions concerning the eye. Here's a simple definition of what different parts of the eye do. Cones: The color receptors of the eye. Cones are used more during daytime when colors are more vibrant and pronounced. There are 7 million cones in the human eye. Cornea: The transparent part of the eye covering the iris and pupil. The cornea refracts light, providing about two-thirds of the eye's optical power. Iris: The iris controls the size and diameter of the pupils, which serves to adjust the amount of light that enters the retina. Lens: The lens helps to refract light and focus it onto the retina. It changes shape to adjust its focal distance so that the eye can focus on objects at different distances. Macula The macula in the human eye is the place where light is focused by the structures in the front of the eye (cornea & lens). It takes the picture that is sent to the brain, where vision is completed. The macula provides us with the ability to read and see in great detail whereas the rest of the retina provides peripheral vision. Optic Nerve: The optic nerve sends information from the retina to the brain so that it can be processed. Optic Disc: The raised disk on the retina at the point of entry of the optic nerve, lacking visual receptors and so creating a blind spot. Retina: The retina lines the inner surface of the eye and creates images based on the light passing through the eye. These images are then sent to the brain via the optic nerve. Rods: The photoreceptors of the eye. Rods sense brightness and are favored during nighttime, when color is mostly absent and objects are viewed on a grayscale. There are 120 million rods in the human eye. Sclera The white outer layer of the eyeball. At the front of the eye it is continuous with the cornea. Colors Many optics tests will include sections about various forms of color mixing. It is critical to understand these concepts. The primary colors of light are red, green, and blue. The secondary colors, meanwhile, are yellow, magenta, and cyan. Colors can be mixed in two different ways - additive and subtractive. Additive mixing occurs in light, such as on a computer screen where red, green, and blue are mixed in different proportions to create all the other colors. Yellow is made of red and green, magenta is made of red and blue, and cyan is made of green and blue. Many optics questions will describe shining multiple lights onto a single spot, which is another form of additive color mixing. Subtractive mixing occurs with physical dyes, like in printing or in paints. When cyan, magenta, and yellow dyes are mixed, only the primary colors in common are visible. Thus, yellow and magenta form red, cyan and yellow form green, and cyan and magenta form blue. Another consideration is colored filters. A colored filter will transmit only the colors of light required to form that color. Therefore, a cyan filter would absorb red light, while transmitting blue and green light, and a red filter would absorb both blue and green light while transmitting red light.