Nester's Microbiology 10th Edition - Chapter 13 Lecture Slides PDF
Document Details
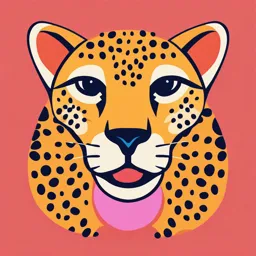
Uploaded by InexpensiveSilver
Denise Anderson, Sarah Salm, Mira Beins
Tags
Summary
These lecture slides cover viruses, viroids, and prions. They discuss viral structure, taxonomy, and replication, providing a comprehensive overview for undergraduate-level students studying microbiology.
Full Transcript
Because learning changes everything.® Chapter 13 Viruses, Viroids, and Prions Lecture Outline Nester's Microbiology A Human Perspective, Tenth Edition Denise Anderson, Sarah Salm, Mira Beins © 2022 McGraw Hill, LLC. All rights reserved. Authorized only for instructor use in the classroom. No repr...
Because learning changes everything.® Chapter 13 Viruses, Viroids, and Prions Lecture Outline Nester's Microbiology A Human Perspective, Tenth Edition Denise Anderson, Sarah Salm, Mira Beins © 2022 McGraw Hill, LLC. All rights reserved. Authorized only for instructor use in the classroom. No reproduction or further distribution permitted without the prior written consent of McGraw Hill, LLC. A Glimpse of History Tobacco mosaic disease (1890s) • Many microorganisms were identified as infectious agents, but this was caused by an unusual agent • D. M. Iwanowsky, Martinus Beijerinck determined it was caused by “filterable virus” too small to be seen with light microscope, passed through filters for bacteria • F. W. Twort and F. d’Herelle discovered “filterable virus” that destroyed bacteria • Virus means “poison” • Viruses have many features more characteristic of complex chemicals (for example, still infective following precipitation from ethyl alcohol suspension or crystallization) © McGraw Hill, LLC 2 Viruses: Introduction Viruses are genetic information — DNA or RNA — contained within protective protein coat • Inert particles: no metabolism, replication, motility • Genome hijacks host cell’s replication machinery • Inert outside cells; inside, direct activities of cell • Infectious agents, not organisms • Viruses require live organisms as hosts; cannot be grown in pure culture • Cannot be seen with light microscopy • Classified generally based on type of cell they infect: eukaryotic or prokaryotic © McGraw Hill, LLC 3 Viruses: Obligate Intracellular Parasites Viruses that infect bacteria are called bacteriophages, or phages • Easy to grow in the lab as a good model for how animal viruses interact with their hosts • Vehicle for horizontal gene transfer • Their ability to kill bacteria is important ecologically and medically © McGraw Hill, LLC 4 Relative Sizes of Viruses – Figure 13.1 Access the text alternative for slide images. © McGraw Hill, LLC 5 Viral Structure – Figure 13.2 Virion (viral particle) is nucleic acid surrounded by a capsid (protein coat) • Capsid composed of simple identical subunits called capsomeres • Capsid plus nucleic acids called nucleocapsid • Nucleic acid is either DNA or RNA but not both; may be circular or linear, single-stranded or double-stranded • Spikes attach to receptor sites on host cells; phages attach by tail fibers • Enveloped viruses are surrounded by lipid bilayer obtained from host cell • Matrix protein between nucleocapsid and envelope • Non-enveloped (naked) viruses are more resistant to disinfectants Access the text alternative for slide images. © McGraw Hill, LLC 6 Viral Structure – Figure 13.3 Three shapes: • Icosahedral • 20 flat triangles • Helical • Capsomeres arranged in helix • Complex viruses • Phage • Icosahedral nucleocapsid (head) and helical protein (tail) a: Eye of Science/Science Source; b: Science Source; c: Biophoto Associates/Science Source Access the text alternative for slide images. © McGraw Hill, LLC 7 Viral Taxonomy 1 • International Committee on Taxonomy of Viruses (ICTV) keeps online database and publishes features, classification, nomenclature of viruses • 2020 report describes 6,590 viral species belonging to 1,421 genera. • Key characteristics include genome structure (nucleic acid and strandedness) and hosts infected • Other characteristics (for example, viral shape, disease symptoms) also considered © McGraw Hill, LLC 8 Viral Taxonomy 2 Although not living organisms, classified as such • International Committee on Taxonomy of Viruses (ICTV) keeps online database and publishes features, classification, nomenclature of viruses • A 2020 report described 6,590 viral species belonging to 1,421 genera • Previously classified based on features such as genome structure, host infected, shape • New taxonomic system includes: • Taxonomic hierarchies similar to other biological disciplines • 8 principal ranks from species through realm • Realm is analogous to domain © McGraw Hill, LLC 9 Viral Taxonomy 3 • Virus families end in suffix – viridae • Names follow no consistent pattern • formal taxonomic names of viruses are written in italics, including all those above genus • Unlike bacteria, viruses are usually referred to by only by their species name or by another name • Neither of which is capitalized or italicized • E.g. rhabdovirus © McGraw Hill, LLC 10 Viral Taxonomy 4 • Viruses often referred to informally • Groups of unrelated viruses sharing routes of infection • Oral-fecal route: enteric viruses • Respiratory route: respiratory viruses • Zoonotic viruses cause zoonoses (animal to human) • Arboviruses (from arthropod borne) are spread by arthropods; often can infect widely different species • Cause diseases such as yellow fever, dengue fever, West Nile encephalitis, La Crosse encephalitis © McGraw Hill, LLC 11 Table 13.2 Grouping of Human Viruses Based on Route of Transmission Virus Group Mechanism of Transmission Common Viruses Transmitted Enteric Fecal-oral route Enteroviruses (polio); noroviruses; rotaviruses (diarrhea) Respiratory Respiratory or salivary route Influenza; measles; rhinoviruses (colds) Sexually transmitted Sexual contact Herpes simplex virus type 2 (genital herpes); HIV Zoonotic Vector (such as arthropods) West Nile encephalitis; Zika virus disease, dengue fever Zoonotic Animal to human directly Rabies; cowpox © McGraw Hill, LLC 12 Focus Your Perspective 13.1 Viruses notable for small size • Smallest viruses are approximately 10 nanometer in diameter • Viruses – group of giant viruses • Changing views on the origin of viruses and possibly life • Largest viruses are approximately 800 nanometer (Megaviridae) • Mimivirus carries about 1.2 million base pairs in its genome, but has no ribosomes • Sputnik is a parasite of mimivirus • Pandoraviruses were discovered in 2013; genome twice the size of mimivirus Dr. Raoult/Science Source © McGraw Hill, LLC 13 Bacteriophages Three general types of bacteriophages based on relationship with host • Lytic (virulent) phages • Temperate phages • Filamentous phages General strategies for phage replication • Productive infection • New viral particles are produced • Latent state • Viral genome remains silent within cell, but is replicated along with host cell genome © McGraw Hill, LLC 14 Bacteriophages – Figure 13.4 Access the text alternative for slide images. © McGraw Hill, LLC 15 Lytic Phage Infections – Figure 13.5 Lytic or virulent phages exit host by lysing and thereby killing the cell • T4 phage, with double stranded DNA, is model • Infection cycle is five step process • Attachment • Genome entry • Synthesis • Assembly • Release Access the text alternative for slide images. © McGraw Hill, LLC 16 Attachment and Genome Entry – Figure 13.5 Attachment • Phage attaches to receptors • Receptors are molecules that typically perform other functions • Cells that lack specific receptor are resistant to infection by that phage Genome entry • T4 lysozyme degrades cell wall • Tail contracts, injects genome through cell wall and membrane • Capsid remains outside the cell Access the text alternative for slide images. © McGraw Hill, LLC 17 Synthesis – Figure 13.5 Synthesis of phage proteins and genome • Early proteins translated within minutes; nuclease degrades host DNA • Phage genome synthesized • Late proteins are structural proteins (capsid, tail); produced toward end of cycle Access the text alternative for slide images. © McGraw Hill, LLC 18 Assembly and Release Figure – 13.5 Assembly (maturation) • Some components assemble spontaneously, others require protein scaffolds Release • Lysozyme produced late in infection; digests cell wall • Cell lyses, releases phage • Burst size of T4 is approximately 200 Access the text alternative for slide images. © McGraw Hill, LLC 19 lytic (virulent) bacteriophages eclipse The time from absorption to release for T-even phages is about 30 minutes © McGraw Hill, LLC Temperate Phage Infections Do not necessarily lyse their host Best studied temperate phage is Lambda Double-stranded DNA phage that infects E. coli. Has linear chromosome, but ends join together to form circular chromosome after injection into cell One of two options then occurs • Lytic Infection • Lysogenic Infection © McGraw Hill, LLC 21 acteriophages - lambda (λ) phage b lambda (λ) phage: linear chromosome; complementary singlestranded overhangs at ends join inside host resulting circular molecule either directs lytic infection or integrates into E. coli chromosome phage enzyme integrase inserts DNA at specific site site specific recombination integrated phage DNA termed prophage replicates with host chromosome -can be excised by phage-encoded enzyme -results in lytic infection repressor prevents excision, maintains lysogenic state © McGraw Hill, LLC Lysogenic Infection – Figure 13.6 Phage-encoded enzyme integrase inserts phage DNA at specific site on host chromosome The integrated phage DNA is called a prophage Bacterial cell carrying prophage is called a lysogen. • Prophage replicates along with host chromosome and is incorporated into cell’s progeny • Most prophage genes are silent because a phageencoded repressor protein prevents expression Access the text alternative for slide images. © McGraw Hill, LLC 23 Lysogenic Infection A prophage can remain in latent state indefinitely But, if prophage is excised by phage encoded enzyme a lytic infection ensues • Happens about once per 10,000 divisions of lysogen • Excision can be induced by a DNA-damaging agent such as ultraviolet light Protease destroys repressor, allows prophage to be excised, enter lytic cycle Called phage induction; allows phage escape damaged host © McGraw Hill, LLC 24 temperate phages - lambda (λ) phage induction DNA excised from chromosome only about once per 10,000 divisions of lysogen -…..if DNA damaged (UV light exposure), SOS repair system turns on, activates a protease GUESS WHAT PROTEASE????! temperate phages - phage induction SOS repair system turns on, activates a protease RecA !!!!!! -protease destroys repressor, allows prophage to be excised, enter lytic cycle -called phage induction; allows phage to escape damaged host Consequences of Lysogeny Immunity to Superinfection Lysogen is immune to superinfection (infection by same phage) • Repressor maintaining integrated prophage also binds to operator on incoming phage DNA, prevents gene expression Lysogenic Conversion - lysogen may show change in phenotype due to prophage DNA • Toxins encoded by phage genes; only strains carrying prophage produce the toxins © McGraw Hill, LLC 27 Filamentous Phage Infections – M13 Phage Single-stranded DNA phages that look like long fibers • Cause productive infections • Infected cells do not die but more slowly than uninfected cells M13 is a filamentous phage that initiates infection by attaching to a protein on the F pilus of E. coli Phage genome enters a bacterial cell, the host cell DNA polymerase synthesizes the complementary strand to create a double-stranded DNA molecule referred to as the replicative form (RF). One strand of the RF is then used to make mRNA and multiple copies of the phage genome © McGraw Hill, LLC 28 M13 Phage – Figure 13.7 Phage mRNA genes are expressed to produce viral proteins. Extrusion - phage particles are assembled as they exit the cell • Phage capsomeres insert into the host’s cytoplasmic membrane • Other phage proteins create pores in cell envelope • As phage DNA is extruded through the pores capsomeres coat it to form a nucleocapsid Access the text alternative for slide images. © McGraw Hill, LLC 29 Replication of a Filamentous Phage – Figure 13.8 Access the text alternative for slide images. © McGraw Hill, LLC 30 Bacteriophages in Horizontal Gene Transfer Errors during the phage replication process play an important role in horizontal gene transfer in bacteria Generalized Transduction • Results from packaging error during phage assembly • Some phages degrade host chromosome; fragments can be mistakenly packaged into phage head creating a transduction particle • Carry bacterial DNA not Phage DNA • Transduction particles can bind to new host, inject bacterial DNA • DNA may integrate via homologous recombination, replacing host DNA • Any gene from donor cell can be transferred © McGraw Hill, LLC 31 Bacteriophages in Horizontal Gene Transfer – Figure 13.9 Specialized Transduction • Excision mistake during transition from lysogenic to lytic cycle of temperate phage • Short piece of bacterial DNA removed • Only genes adjacent to phage DNA Access the text alternative for slide images. © McGraw Hill, LLC 32 Methods Used to Study Bacteriophages – Figure 13.10 Viruses multiply only inside living cells; must cultivate suitable host cells to grow viruses Plaque assays used to count phage particles in samples: sewage, seawater, soil • Soft agar inoculated with bacterial host and phage-containing specimen • Bacterial lawn forms • Zones of clearing from bacterial lysis are plaques • Plaque-forming unit (PFU) represents single phage • Counting plaques yields the titer, which is concentration of phage in the original sample © McGraw Hill, LLC 33 Animal Virus Replication Important from a medical standpoint • Often depends on virally encoded enzymes which are potential targets of antiviral drugs • By interfering with the activities of these enzymes, antiviral medications can slow the progression of a viral infection • Gives host immune system time to eliminate the virus before illness occurs Infection cycle of animal viruses can be viewed as a five-step process: attachment, genome entry, synthesis, assembly, and release © McGraw Hill, LLC 34 animal virus replication 5-step infection cycle: 1.attachment 2. penetration and uncoating 3. synthesis (replication) antigenic shift, antigenic drift, reverse transcription 4. assembly 5. release https://www.youtube.com/watch?v=PlSvywlLuNw HIV hhmi © McGraw Hill, LLC nimal virus replication a five-step infection cycle 1. Attachment(adsorption) Virus spikes bind to receptors usually glycoproteins on cytoplasmic membrane often more than one receptor required (HIV binds to two) normal receptor function unrelated to viral attachment specific receptors (tropism) required; limits host range of virus …dogs don’t contract measles from humans… Tropism: Most viruses can only infect a single species Some animals resistant to certain viruses © McGraw Hill, LLC Measles virus uses a surface protein hemaglutinin to bind to a target receptor on the host cell Once bound, the fusion, or F protein helps the virus fuse with the membrane and ultimately get inside the cell Wikipedia The virus contacts host lung tissue, where it infects immune cells called macrophages and dendritic cells, which serve as an early defense and warning system. www.sciencemag.org › news › 2015/01 › what-does-measlesactually-do Animal Virus Replication: Attachment Attachment (Adsorption) • Virus attachment proteins (spikes) bind to receptors on host cell surface, usually glycoproteins on cytoplasmic membrane • Often more than one receptor attachment is required (for example, HIV binds to two) • Normal function of receptor molecule unrelated to viral infection • Particular viruses must attach to specific receptor; limits cell types and tissues a virus can infect • Most viruses infect a single species (dogs do not contract measles from humans), but that is not always true (rabies virus infects dogs and humans) © McGraw Hill, LLC 38 Animal Virus Replication: Entry and Uncoating – Figure 13.11 • Enveloped viruses enter by fusion or endocytosis • Non-enveloped viruses cannot fuse, enter by endocytosis • Entire virion enters cell; nucleic acid separates from protein coat in process of uncoating Access the text alternative for slide images. © McGraw Hill, LLC 39 Animal Virus Replication: Synthesis Synthesis requires two events • Expression of viral genes to produce viral structural and catalytic genes (for example, capsid proteins, enzymes required for replication) • Often synthesized as polyprotein that is cleaved by viral proteases (a target site of antiviral medications) • Synthesis of multiple copies of viral genome • Three general replication strategies depending on type of genome of virus • DNA viruses • RNA viruses • Reverse transcribing viruses © McGraw Hill, LLC 40 animal virus replication 3. Synthesis Production of viral particles require: • expression of viral genes to produce viral structural and catalytic genes (capsid proteins, enzymes required for replication) • synthesis of multiple copies of genome most DNA viruses multiply in nucleus enter through nuclear pores using NLS three general replication strategies depending on type of genome of virus 1. DNA viruses 2. RNA viruses 3. reverse transcribing viruses © McGraw Hill, LLC animal virus replication 3. synthesis replication of DNA viruses usually in nucleus (poxviruses - exception: replicate in cytoplasm, encode all enzymes for DNA, RNA synthesis) dsDNA replication straightforward - follows central dogma animal virus replication 3. synthesis replication of ssDNA viruses ssDNA similar except complement first synthesized to generate doublestranded DNA. Newly synthesized strand acts as template to produce more ssDNA animal virus replication 3. synthesis replication of RNA viruses majority single-stranded; replicate in cytoplasm require virally encoded RNA polymerase (replicase - RNA directed RNA polymerase), - uses RNA template to synthesize new RNA strand which lacks proofreading, allows antigenic drift ->influenza viruses animal virus replication 3. synthesis replication of RNA viruses majority single-stranded; replicate in cytoplasm require virally encoded RNA polymerase (replicase - RNA directed RNA polymerase), ss (+) RNA genomes are functional mRNAs Flaviviruses, Polio virus, Rhinovirus, Coronavirus Viral RNA binds host ribosomes and gets translated to make viral proteins, including a viral replicase that is used to synthesize more copies of the viral genome. To do this, it 1. makes multiple copies of the complementary (-) strand using the original (+) strand as a template. 2. these (-) strands act as templates to produce more viral (+) strand RNA packaged as genomes into new virons forming. animal virus replication 3. synthesis replication of RNA viruses require virally encoded RNA polymerase (replicase - RNA directed RNA polymerase), - lacks proofreading – generates mutations during replication. ss (–) RNA cannot be directly translated – must be copied into a (+) strand. Influenza virus, Measles virus, Ebola virus A replicase is carried into host cell. Once (+) strand produced, it can also be translated to make viral proteins and used as template for synthesizing new (-) RNA strands. dsRNA viruses Rotaviruses Also carry RNRP to synthesize (+) strand uses (-) RNA strand to make (+)strand RNA Which gets translated to make more replicase antigenic drift antigenic drift: mechanism for variation in viruses involves accumulation of mutation in genes that code for antibody-binding sites. antigenic drift: mechanism for variation in viruses involves accumulation of mutations in genes that code for antibody-binding sites. example: influenza viruses can change through antigenic drift, a process in which mutations to the virus genome produce changes in the viral H or N. Influenza virus: ss (-) strand virus antigenic drift: mechanism for variation in viruses involves accumulation of mutations in genes that code for antibody-binding sites. example: influenza viruses can change through antigenic drift, a process in which mutations to the virus genome produce changes in the viral H or N. Influenza virus: ss (-) strand virus Hemaglutinin - glycoprotein on surface, binds cells with sialic acid on membrane Neuraminidase - surface protein - enables viral release from cell. Oseltamivir (Tamiflu) - neuraminidase inhibitor antigenic drift: mechanism for variation in viruses involves accumulation of mutations in genes that code for antibody-binding sites. example: influenza viruses can change through antigenic drift, a process in which mutations to the virus genome produce changes in the viral H or N. antigenic drift - continuous, ongoing process resulting in emergence of new strain variants. antigenic drift - influenza virus seasonal changes cause gradual change in the HA and/or NA spikes antigenic shift - influenza virus (acute infection) Reassortment of segmented viruses genomes causes antigenic shift – there is a sudden change in spikes because the virus acquires a new genome segment. reassortment Why RNA viruses recombine Recombination in RNA viruses involves the formation of chimeric molecules from parental genomes of mixed origin. – requires that 2 or more viruses infect the same host cell. Co-infection of a cell by genetically distinct viral strains can lead to the generation of recombinant viruses. Coronavirus is an unsegmented RNA virus. natural selection acts on recombinants some RNA viruses segmented reassortment results in antigenic shift Two different segmented viruses infect the same cell Reassortment of the segments results in recombinants Influenza virus has a segmented genome antigenic shift: -two or more different strains of a virus, or strains of two or more different viruses, combine to form new subtype having mixture of surface antigens of original strains. REASSORTMENT http://www.hhmi.org/biointeractive/recombination-viral-genome Replication of DNA Viruses – Figure 13.12 Replication of double stranded DNA viruses • DNA (+/ −) is replicated to form viral genome • (−) strand transcribed to produce mRNA; translated to make viral proteins Replication of single stranded DNA viruses • Complement to DNA synthesized first; then acts as template to produce more copies of viral genome • (−) strand transcribed to produce mRNA Access the text alternative for slide images. © McGraw Hill, LLC 62 Replication of RNA Viruses 1 Replicate in cytoplasm Require virally encoded RNA-dependent RNA polymerase (replicase) Allows use of RNA template to make new strand of RNA Replication strategy varies with viral genome • (+) single stranded RNA genome also serves as mRNA • (−) single stranded RNA genome is complement to mRNA • (+/ −) double stranded RNA genome contains both © McGraw Hill, LLC 63 Replication of (+) Single Stranded RNA Viruses – Figure 13.13 Viral RNA binds to ribosome; produces viral replicase Viral replicase produces multiple copies of complementary (−) RNA strand These act as templates to produce more (+) strands • Can serve as viral genome or be translated to produce viral proteins Access the text alternative for slide images. © McGraw Hill, LLC 64 Replication of (-) Single Stranded RNA Viruses – Figure 13.13 • Viral RNA and replicase both enter host cell to make complementary (+) RNA strand • (+) RNA strand can serve as mRNA to make viral proteins • Multiple copies of complementary (−) RNA strand produced to serve as viral genome Access the text alternative for slide images. © McGraw Hill, LLC 65 Replication of Double Stranded RNA Viruses – Figure 13.13 • Replicase enters host cell with dsRNA because host cell cannot translate dsRNA • Replicase uses (−) RNA strand to produce (+) RNA strand • (+) RNA strand can serve as mRNA to make viral proteins Access the text alternative for slide images. © McGraw Hill, LLC 66 Replication of RNA Viruses 2 Most replicases lack proofreading ability • Generates mutations during replication • Results in antigenic variation called antigenic drift • Mutations in surface proteins may not be recognized by immune system Some RNA viruses have segmented genomes • When two different viruses or strains infect a host, new viral particles may contain segments from each virus • New subtype results from this reassortment – process called antigenic shift © McGraw Hill, LLC 67 Replication of Reverse-Transcribing Viruses – Figure 13.14 • Encode reverse transcriptase: makes DNA from RNA • Retroviruses have (+) ssRNA genome (such as HIV) • Reverse transcriptase enters the cell and synthesizes a single DNA strand from RNA template • Complementary DNA strand synthesized; dsDNA integrated into host cell chromosome • Can be transcribed to produce new viral genome, mRNA Access the text alternative for slide images. © McGraw Hill, LLC 68 Viral Assembly and Release Assembly (Maturation) • Involves packaging the nucleic acid into the capsid to form the nucleocapsid. • Spontaneous self-assembly when viral nucleic acid and capsid proteins accumulate in host cell • Site of assembly varies with virus type and affects release Release • Most enveloped viruses leave via budding • Viral protein spikes insert into host cell membrane; matrix proteins accumulate in same area; nucleocapsids extruded • Covered with matrix protein and lipid envelope • Some obtain envelope from organelles • Non-enveloped viruses released when host cell dies, often by apoptosis initiated by virus or host Virions must be transmitted to another host via urine blood etc. © McGraw Hill, LLC 69 Animal Virus Replication – Figure 13.15 b: Centers for Disease Control and Prevention Access the text alternative for slide images. © McGraw Hill, LLC 70 Categories of Animal Virus Infections Acute Infections • Rapid onset; short duration • Burst of virions released from infected host cell • Immune system gradually eliminates virus • Influenza, mumps, and poliomyelitis Persistent Infections • Continue for years or lifetime • May or may not have symptoms Some viruses do not fall neatly into categories (e.g. HIV) © McGraw Hill, LLC 71 Persistent Infections May be chronic or latent • Chronic infections: continuous production of low levels of virus particles • Carriers may lack symptoms, but still transmit virus • Latent infections: viral genome remains silent in host cell • Can reactivate to cause productive infection • Viral genome may be integrated into host cell chromosome as a provirus. © McGraw Hill, LLC 72 Categories of Animal Virus Infections – Figure 13.16 Access the text alternative for slide images. © McGraw Hill, LLC 73 Categories of Animal Virus Infections – Figure 13.17 Latent infections • Provirus integrates into host chromosome or replicates separately, much like a plasmid • Cannot be eliminated; can later be reactivated • Examples are varicella zoster virus (VSV) and herpes simplex type 1 virus (HSV-1) Access the text alternative for slide images. © McGraw Hill, LLC 74 Viruses and Human Tumors Tumor is abnormal growth • Cancerous or malignant tumor can metastasize; benign do not • Proto-oncogenes stimulate cell growth and division; tumor suppressor genes inhibit growth division • Mutations in these genes cause abnormal and/or uncontrolled growth • Multiple changes at different sites required • Oncogene is proto-oncogene that has been changed to promote uncontrolled growth • Virus may carry an oncogene • Numerous events, including spontaneous and induced mutations, can lead to conversion into an oncogene. • Some viruses offer potential for treating cancers © McGraw Hill, LLC 75 Cancer-Causing Viruses Oncoviruses – Viruses that can cause cancer in humans • May arise directly from viral infection • Or when viral genome acts as an oncogene • Or when viral genome inserts into the host chromosome in such a way that it converts a proto-oncogene to an oncogene • Most virus-induced tumors associated with DNA viruses • Some RNA viruses also oncogenic • Currently seven recognized human oncogenic viruses Cancers caused by the viruses do not develop immediately • Present 15 to 40 years after infection • Small percentage of oncovirus-infected people develop the associated cancer. • Some virus-associated cancers are preventable by vaccines (HPVs) © McGraw Hill, LLC 76 Table 13.5 Viruses Associated with Cancers in Humans Virus Type of Nucleic Acid Kind of Tumor Epstein-Barr DNA Burkitt’s lymphoma; nasopharyngeal carcinoma; B-cell lymphoma Hepatitis B DNA Hepatocellular carcinoma Human herpesvirus type 8 DNA Kaposi’s sarcoma Human papillomaviruses (HPVs) DNA Different kinds of tumors, caused by different HPV types Merkel cell polyomavirus (MCPyV) DNA Merkel cell carcinoma (skin cancer) Hepatitis C RNA Hepatocellular carcinoma HTVL-1 RNA (retrovirus) Adult T-cell leukemia (rare) © McGraw Hill, LLC 77 Various Effects of Animal Viruses on the Cells They Infect– Figure 13.18 Access the text alternative for slide images. © McGraw Hill, LLC 78 Cancer-Fighting Viruses Oncolytic viruses specifically target and kill cancer cells • Destroy the cancer cells directly by multiplying within them and causing lysis • Others act indirectly by stimulating the host’s cancer-fighting immune cells. • Genetically engineering other well-characterized viruses to develop oncolytic versions for therapy In 2015, the Food and Drug Administration (FDA) approved a genetically engineered herpes simplex virus type 1 to treat advanced inoperable melanoma • Engineered virus is injected directly into melanoma lesions • Preferentially infects and multiplies in the tumor cells, ultimately causing them to lyse © McGraw Hill, LLC 79 Cultivating and Quantitating Animal Viruses – Figure 13.19 Viruses must be grown in appropriate host • Once done by inoculating live animals; or preparing embryonated (fertilized) chicken eggs • Cell culture or tissue culture now commonly used • Process animal tissues to obtain primary cultures • Cells divide slowly and only a limited number of times • Tumor cells often used, multiply indefinitely Dr. Cecil Fox/National Cancer Institute (NCI) Access the text alternative for slide images. © McGraw Hill, LLC 80 Effects of Viral Replication of Cell Cultures – Figure 13.20 • Many viruses cause distinct morphological alterations called cytopathic effect • Cells may change shape, detach from surface, lyse, fuse into giant multinuclear cell (syncytium), or form inclusion body (site of viral replication) ©Modified from “The Genome Sequence of Lone Star Virus, a Highly Divergent Bunyavirus Found in the Amblyomma americanum Tick,” PLOS ONE, April 2013, vol. 8, Issue 4, e62083, with permission from University of California, San Francisco and the Centers for Disease Control and Prevention. © McGraw Hill, LLC 81 Quantitating Animal Viruses – Figure 13.21 Plaque assay using monolayer of tissue culture cells Direct count via electron microscopy Quantal assay: dilutions of virus given to hosts • Titer is dilution at which 50% of hosts are infected (ID50– infective dose) or killed (LD50– lethal dose) CDC/ Dr. Erskine Palmer © McGraw Hill, LLC 82 Quantitating Animal Viruses – Figure 13.22 • Hemagglutination: some viruses cause red blood cells to agglutinate (clump) • Mix virus with dilutions of red blood cells • Highest dilution with maximum agglutination is titer of the virus Access the text alternative for slide images. © McGraw Hill, LLC 83 Plant Viruses Economically important, particularly when they affect crops Recognized through visible signs: • Yellowing of foliage, irregular lines on leaves and fruit • Individual cells or organs may die • Tumors may appear • Stunted growth • But growth may be stimulated leading to deformed structures Plants generally do not recover from viral infections • Cannot develop specific immunity © McGraw Hill, LLC 84 Tobacco Mosaic Virus Virions may accumulate in enormous numbers • 10% the dry weight of a tobacco mosaic virus-infected plant may consist of virus Serious disease of tobacco Difficult to eliminate from contaminated area because virions remain infective for at least 50 years Other plant viruses are also extraordinarily stable in the environment. © McGraw Hill, LLC 85 Plant Viruses – Figures 13.23 • Do not attach to cell receptors • Enter via wounds in cell wall, spread through cell openings (plasmodesmata) • Transmitted by soil; humans; insects; contaminated seeds, tubers, pollen; grafting • Difficult to eliminate © McGraw Hill, LLC 86 Other Infectious Agents: Viroids Viroids are small single-stranded RNA molecules that form a closed ring • Thus far only found in plants; cause serious disease • Examples include potato spindle tuber, chrysanthemum stunt, citrus exocortis, cucumber pale fruit, and cadangcadang • Enter through wound sites • Many questions remain: • How do they replicate? • How do they cause disease? • How did they originate? © McGraw Hill, LLC 87 Other Infectious Agents: Prions Prions are proteinaceous infectious agents • Composed solely of protein; no nucleic acids • Linked to slow, fatal diseases in humans and animals Human diseases: Creutzfeldt-Jakob disease, fatal familial insomnia, and kuru Animal diseases: scrapie (sheep and goats), mad cow disease or bovine spongiform encephalopathy (cattle), and chronic wasting disease (deer and elk) © McGraw Hill, LLC 88 Other Infectious Agents: Prions – Figure 13.25 Prion proteins accumulate in neural tissue • Neurons die • Tissues develop holes • Brain function deteriorates • Characteristic appearance gives rise to general term for all prion diseases transmissible spongiform encephalopathies a: M. Abbey/Science Source; b: Source: Sherif Zaki; MD; PhD; Wun-Ju Shieh; MD; PhD; MPH/CDC © McGraw Hill, LLC 89 Other Infectious Agents: Prions – Figure 13.26 Cells produce normal form of neuronal protein PrPc Infectious prion proteins are PrPs Resistant to proteases Insoluble, forms aggregates Appears that PrPsc changes folding of PrPc converting it to PrPsc P Prions are resistant to heat and chemical treatments b: Eye of Science/Science Source Access the text alternative for slide images. © McGraw Hill, LLC 90 Because learning changes everything. www.mheducation.com © 2022 McGraw Hill, LLC. All rights reserved. Authorized only for instructor use in the classroom. No reproduction or further distribution permitted without the prior written consent of McGraw Hill, LLC. ®