Muscles Physiology PDF
Document Details
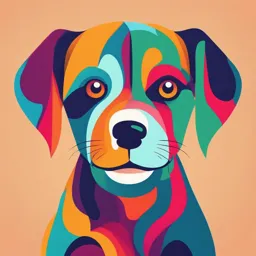
Uploaded by RecordSettingSilicon
Tags
Summary
This document provides an overview of muscle physiology, focusing specifically on the structure, function, and regulation of skeletal, cardiac, and smooth muscle. It covers topics from muscle tissue types to the detailed molecular mechanisms of contraction. This document is likely part of a course or presentation on muscular physiology.
Full Transcript
Excitable Tissue: Skeletal Muscle & smooth muscle LEC. SABA NASEER ABBAS objectives 1. Differentiate the major classes of muscle in the body. 2. Describe the molecular and electrical makeup of muscle cell excitation– contraction coupling. 3. Define elements of the sarcomere that underlie striate...
Excitable Tissue: Skeletal Muscle & smooth muscle LEC. SABA NASEER ABBAS objectives 1. Differentiate the major classes of muscle in the body. 2. Describe the molecular and electrical makeup of muscle cell excitation– contraction coupling. 3. Define elements of the sarcomere that underlie striated muscle contraction. 4. Differentiate the role(s) for Ca2+ in skeletal and smooth muscle contraction. 5. Appreciate muscle cell diversity and function. Introduction Muscle cells, like neurons, can be excited chemically, electrically, and mechanically to produce an action potential. Unlike neurons, they respond to stimuli by activating a contractile mechanism. The contractile protein myosin and the cytoskeletal protein actin are abundant in muscle, where they are the primary structural components that bring about contraction. Muscle is generally divided into three types: skeletal, cardiac, and smooth, although smooth muscle is not a homogeneous single category. Skeletal muscle Cardiac muscle Smooth muscle Makes up the great Has cross-striations Lacks cross-striations. mass of the somatic Functionally syncytial. Subdivided into two musculature. Although it can be broad types: unitary (or Well-developed cross- modulated via the visceral) smooth muscle striations. autonomic nervous and multiunit smooth Does not contract in system, it can contract muscle. the absence of nervous rhythmically in the stimulation. absence of external Generally under innervation owing to voluntary control the presence in the myocardium of pacemaker cells that discharge spontaneously. Skeletal Muscle Morphology oSkeletal muscle is made up of individual muscle fibers that are the “building blocks” of the muscular system in the same sense that the neurons are the building blocks of the nervous system. o Most skeletal muscles begin and end in tendons, and the muscle fibers are arranged in parallel between the tendinous ends. The term muscle refers to a number of muscle fibers bound together by connective tissue. oThe most striking feature seen when viewing skeletal muscle through a microscope (figure1) is a distinct series of alternating light and dark bands perpendicular to the long axis. Because cardiac muscle shares this characteristic striped pattern, these two types are both referred to as striated muscle. oThe third basic muscle type, smooth muscle, derives its name from the fact that it lacks this striated appearance. The striations The myosin and actin filaments interdigitate and cause the myofibrils to have alternate light and dark bands. The light bands are only Actin filaments called I bands, they are isotropic to polarized lights. The dark bands contain Myosin (twice molecular weight of Actin) called A bands; they are anisotropic to polarized lights. So the striations are due to difference in the refractive index of the parts of the muscle fibers. The I band is divided in the middle by darker Z line The A band is divided by the lighter H band, in the middle of it a transverse line called M line. The striations The ends of the actin filaments are attached to a so-called Z disc. From this disc, these filaments extend in both directions to interdigitate with the myosin filaments. The Z disc, which itself is composed of filamentous proteins different from the actin and myosin filaments, passes crosswise across the myofibril and also crosswise from myofibril to myofibril, attaching the myofibrils to one another all the way across the muscle fiber. Therefore, the entire muscle fiber has light and dark bands, as do the individual myofibrils. These bands give skeletal and cardiac muscle their striated appearance. The portion of the myofibril (or of the whole muscle fiber) that lies between two successive Z discs is called a sarcomere. When the muscle fiber is contracted, as shown in Figure 4, the length of the sarcomere is about 2 micrometers. At this length, the actin filaments completely overlap the myosin filaments, and the tips of the actin filaments are just beginning to overlap one another. At this length the muscle is capable of generating its greatest force of contraction. Each muscle fiber is a single cell that is multinucleated, long, cylindrical, and surrounded by a cell membrane, the sarcolemma. The muscle fibers are made up of myofibrils, which are divisible into individual filaments. These myofilaments contain several proteins that together make up the contractile machinery of the skeletal muscle. The Sarcolemma Is a Thin Membrane Enclosing a Skeletal Muscle Fiber. At each end of the muscle fiber, this surface layer of the sarcolemma fuses with a tendon fiber. The tendon fibers in turn collect into bundles to form the muscle tendons that then insert into the bones. Myofibrils Are Composed of Actin and Myosin Filaments Each muscle fiber contains several hundred to several thousand myofibrils. Each myofibril is composed of about 1500 adjacent myosin filaments and 3000 actin filaments, which are large polymerized protein molecules that are responsible for the actual muscle contraction. The thick filaments in the diagrams are myosin, and the thin filaments are actin. The molecular structure of thick and thin filaments is shown in (Figure 2). The thick filaments are composed almost entirely of the protein myosin. The myosin molecule is composed of two large polypeptide heavy chains and four smaller light chains. These polypeptides combine to form a molecule that consists of two globular heads extend out to the sides, forming cross-bridges, which make contact with the thin filament and exert force during muscle contraction. The thin filaments are principally composed of the protein actin, as well as two other proteins— troponin and tropomyosin—that play important roles in regulating contraction. An actin molecule is a globular protein composed of a single polypeptide (a monomer) that polymerizes with other actin monomers to form a polymer made up of two intertwined, helical chains. These chains make up the core of a thin filament. Each actin molecule contains a binding site for myosin. The sarcotubular system Because the skeletal muscle fiber is so large, the action potential cannot flow deep within the muscle fiber to cause maximum muscle contraction, current must penetrate deeply into the muscle fiber, and this is by: Transverse (T) tubules. It is a system of transverse tubules in the form of letter T. Is continuous with the membrane of the muscle fiber, it starts from one side of the cell membrane to the opposite side. They contain extracellular fluid inside. Is responsible for spreading of action potential from the cell membrane to the interior of the muscle fiber, the electrical currents around them create the muscle contraction. The sarcoplasmic reticulum it forms an irregular system of tubules surrounding the myofibrils it has an enlarging ends or chambers called terminal cisterns in close contact with the T system at the junction between A and I bands. The arrangement of the T system with the ciatern of the endoplasmic reticulum at either side called Traid. The sarcoplasmic reticulum contains excess amounts of calcium ions (in the cistern) in high concentration which are released when the action potential occurs in the adjacent tubules. After the contraction has been occurred, active calcium pump located in the walls of sarcoplasmic reticulum pumps calcium back to the sarcoplasmic tubules. Note: as a result, at rest the normal Ca2+ concentration in the remaining cytoplasm is very low Skeletal Muscle Contraction The term contraction, as used in muscle physiology, does not necessarily mean “shortening.” The neurons whose axons innervate skeletal muscle fibers are known as motor neurons (or somatic efferent neurons), and their cell bodies are located in the brainstem and the spinal cord. The axons of motor neurons are myelinated and are the largest-diameter axons in the body. They are therefore able to propagate action potentials at high velocities, allowing signals from the central nervous system to travel to skeletal muscle fibers with minimal delay. Upon reaching a muscle, the axon of a motor neuron divides into many branches, each branch forming a single junction with a muscle fiber. A single motor neuron innervates many muscle fibers, but each muscle fiber is controlled by a branch from only one motor neuron. A motor neuron plus the muscle fibers it innervates is called a motor unit. Skeletal Muscle Contraction The axon terminals of a motor neuron contain vesicles similar to those found at synaptic junctions between two neurons. The vesicles contain the neurotransmitter acetylcholine (Ach). The region of the muscle fiber plasma membrane that lies directly under the terminal portion of the axon is known as the motor end plate. The junction of an axon terminal with the motor end plate is known as a neuromuscular junction. General Mechanism of Muscle Contraction The initiation and execution of muscle contraction occur in the following sequential steps. 1. An action potential travels along a motor nerve to its endings on muscle fibers. 2. At each ending, the nerve secretes a small amount of the neurotransmitter substance acetylcholine. 3. The acetylcholine acts on a local area of the muscle fiber membrane to open multiple "acetylcholine-gated" cation channels through protein molecules floating in the membrane. 4. Opening of the acetylcholine-gated channels allows large quantities of sodium ions to diffuse to the interior of the muscle fiber membrane. This causes a local depolarization that in turn leads to opening of voltage-gated sodium channels. This initiates an action potential at the membrane. 5. The action potential travels along the muscle fiber membrane in the same way that action potentials travel along nerve fiber membranes. 6. The action potential depolarizes the muscle membrane, and much of the action potential electricity flows through the center of the muscle fiber. Here it causes the sarcoplasmic reticulum to release large quantities of calcium ions that have been stored within this reticulum. 7. Action potential travels along the sarcolemma and down the T-tubules deep into muscle fiber. Action potential triggers Ca++ release from the SR to the sarcoplasm 8. Ca++ binds to troponin exposing myosin-binding sites. 9. After a fraction of a second, the calcium ions are pumped back into the sarcoplasmic reticulum by a Ca++ membrane pump and remain stored in the reticulum until a new muscle action potential comes along; this removal of calcium ions from the myofibrils causes the muscle contraction to cease. Characteristics of whole muscle contraction Types of contraction A. Isometric contraction: is when the muscle does not shorten during contraction i.e. no change in muscle length, but the tension will increase. e.g. trying to lift a heavy object. The work done here is zero, because no movement. B. Isotonic contraction: It is the contraction that causes shortening of the muscle length and the muscle has the same tension. e.g. lifting an object by contracting the biceps muscle. Note: Muscles can only contract (“pull”); they cannot extend with force (“push”). To move in both directions about a joint requires two sets of muscles; e.g. flexors and extensors Muscle Fatigue Prolonged and strong muscle contraction lead to muscle fatigue. This results from: 1. Depletion of glycogen stores in the muscle (inability of the contractile and metabolic processes to continue supplying the same work). 2. Diminished transmission in the NMJ (neuromuscular joint). 3. Interruption of blood supply or flow results in nutrient and O2 deficiency. Smooth Muscle Two characteristics are common to all smooth muscles: 1. They lack the cross-striated banding pattern which makes them “smooth”. 2. The nerves to them are part of the autonomic division of the nervous system rather than the somatic division. Thus, smooth muscle is not normally under direct voluntary control. Smooth muscle, like skeletal muscle, uses cross-bridge movements between actin and myosin filaments to generate force, and calcium ions to control cross-bridge activity. Smooth muscle can generally be divided into two major types: 1- Multi-unit smooth muscle and 2- Unitary (or single-unit) smooth muscle. Multi-Unit Smooth Muscle This type of smooth muscle is composed of discrete, separate smooth muscle fibers. Each fiber operates independently of the others and often is innervated by a single nerve ending. The outer surfaces of these fibers are covered by a thin layer of basement membrane-like substance, a mixture of fine collagen and glycoprotein that helps insulate the separate fibers from one another. Characteristic of multi-unit smooth muscle fibers is that each fiber can contract independently of the others, and their control is exerted mainly by nerve signals. Examples: ciliary muscle of the eye and the iris muscle of the eye. Unitary Smooth Muscle Also called syncytial smooth muscle (due to its syncytial interconnections among fibers) or visceral smooth muscle (because it’s found in the walls of most viscera of the body, including the gastrointestinal tract, bile ducts, ureters, uterus, and many blood vessels). The term "unitary" is confusing because it does not mean single muscle fibers. Instead, it means a mass of hundreds to thousands of smooth muscle fibers that contract together as a single unit. The fibers usually are arranged in sheets or bundles, and their cell membranes are adherent to one another at multiple points so that force generated in one muscle fiber can be transmitted to the next. In addition, the cell membranes are joined by many gap junctions through which ions can flow freely from one muscle cell to the next so that action potentials or simple ion flow without action potentials can travel from one fiber to the next and cause the muscle fibers to contract together. o Smooth muscle contains both actin and myosin filaments. o It does not contain the normal troponin complex that is required in the control of skeletal muscle contraction, so the mechanism for control of contraction is different. o The thin filaments are anchored either to the plasma membrane or to cytoplasmic structures known as dense bodies, which are functionally similar to the Z lines in skeletal muscle fibers. Smooth Muscle Contraction and Its Control Because smooth muscle lacks the Ca++-binding protein troponin, tropomyosin is never held in a position that blocks cross-bridge access to actin. Thus, the thin filament is not the main switch that regulates crossbridge cycling. Instead, cross-bridge cycling in smooth muscle is controlled by a Ca++-regulated enzyme that phosphorylates myosin. Only the phosphorylated form of smooth muscle myosin can bind to actin and undergo cross-bridge cycling. The following sequence of events occurs after an increase in cytosolic Ca++ in a smooth muscle fiber: 1. Ca++ binds to calmodulin, a Ca++-binding protein that is present in the cytosol of most cells and whose structure is related to that of troponin. 2. The Ca++–calmodulin complex binds to another cytosolic protein, myosin light-chain kinase, thereby activating the enzyme. 3. Active myosin light-chain kinase then uses ATP to phosphorylate myosin light chains in the globular head of myosin. 4. Phosphorylation of myosin drives the cross-bridge away from the thick filament backbone, allowing it to bind to actin. 5. Cross-bridges go through repeated cycles of force generation as long as myosin light chains are phosphorylated. A key difference here is that Ca++-mediated changes in the thick filaments turn on cross-bridge activity in smooth muscle, whereas in striated muscle, Ca++ mediates changes in the thin filaments Smooth muscle relaxation To relax a contracted smooth muscle, myosin must be dephosphorylated because dephosphorylated myosin is unable to bind to actin. This dephosphorylation is mediated by the enzyme myosin light-chain phosphatase, which is continuously active in smooth muscle during periods of rest and contraction. When cytosolic Ca++ concentration increases, the rate of myosin phosphorylation by the activated kinase exceeds the rate of dephosphorylation by the phosphatase and the amount of phosphorylated myosin in the cell increases, producing an increase in tension. When the cytosolic Ca++ concentration decreases, the rate of phosphorylation decreases below that of dephosphorylation and the amount of phosphorylated myosin decreases, producing relaxation. Latch state In some smooth muscles, when stimulation is persistent and the cytosolic Ca++ concentration remains elevated, the rate of ATP hydrolysis by the cross-bridges declines even though isometric tension is maintained. This condition is known as the latch state and a smooth muscle in this state can maintain tension in an almost rigor like state without movement. Dissociation of cross-bridges from actin does occur in the latch state, but at a much slower rate. The net result is the ability to maintain tension for long periods of time with a very low rate of ATP consumption. A good example of the usefulness of this mechanism is seen in sphincter muscles of the gastrointestinal tract, where smooth muscle must maintain contraction for prolonged periods. Local Factors Oxygen and carbon dioxide concentration, osmolarity, and the ionic composition of the extracellular fluid, can also alter smooth muscle tension. Some smooth muscles can also respond by contracting when they are stretched. Stretching opens mechanically gated ion channels, leading to membrane depolarization. The resulting contraction opposes the forces acting to stretch the muscle Many of local factors induce smooth muscle relaxation. Nitric oxide (NO) is one of the most commonly encountered paracrine compounds that produce smooth muscle relaxation.