Cardiovascular System Physiology PDF
Document Details
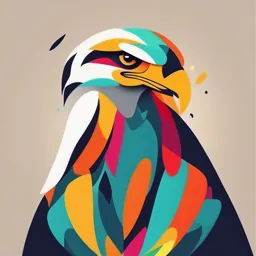
Uploaded by SafeLagrange
Cairo University Medicine
Dr / Moh. A-Aziz Dr / Ah. Fayad
Tags
Summary
These notes cover the physiology of the cardiovascular system, focusing on the heart's function as a pump, its tissue layers, and the pulmonary and systemic circulatory pathways. It also discusses fetal circulation and cardiac muscle mechanics. The document includes detailed descriptions of different components of the circulatory system with emphasis on the role of different parts of heart in pumping blood.
Full Transcript
CardioVascular System Physiology **Prepared by** **Dr / Moh. A-Aziz** **Dr / Ah. Fayad** **https://t.me/physio\_tricks** **THE HEART AS A PUMP** **Function**: pump to maintain pressure the **cardiovascular** system consists of the **heart**, which contains the base of both circuits (systemic...
CardioVascular System Physiology **Prepared by** **Dr / Moh. A-Aziz** **Dr / Ah. Fayad** **https://t.me/physio\_tricks** **THE HEART AS A PUMP** **Function**: pump to maintain pressure the **cardiovascular** system consists of the **heart**, which contains the base of both circuits (systemic and pulmonary) and oversees two pumps in one, and the **vascular** system, which is a series of blood vessels that interconnect to the heart and all other parts of the body, helping to control the flow of blood. The cardiovascular system is a closed system in that the blood never leaves the vessels, but elements of it are filtered out into the tissues and reabsorbed back into the blood vessels. The Cardiovascular System primary function is **transportation** around the body of: - O2 from the lungs to body tissues. - CO2 from body tissues to lungs. - Delivery of Nutrients to all cells and tissues of the body. - Removal of Waste (+ toxins) from cells and tissues of the body. - Circulation of Hormones. - Circulation of defense, healing, immune cells for the repair and protection of body tissues. The circulation of blood is also a key factor in the **thermoregulation** of the body. Blood is the warmest element of the body and its continuous kinetic energy offers heat to any area it heavily perfuses. **The Heart in in a Protective Sac** The heart is a muscular organ enclosed in the **pericardial sac**. The main purpose of the pericardial sac is to **reduce friction** between the heart and the other nearby structures. Since the heart is constantly beating (about 100,000 beats/day), and the nearby lungs are also constantly expanding and deflating, it is vital to have a wet slippery surface that reduces the friction between two surfaces that must constantly move across one another. **The Tissue Layers of the Heart** The heart has 3 tissue layers. The outermost layer is called the pericardium. The middle layer is called the myocardium; this is cardiac muscle and composes most (90%) of the heart. This muscle tissue contracts and generates force to pump blood. The innermost layer is the endocardium across which blood flows. It is lined with endothelium which contains a single layer of vey flat epithelial cells. This also lines all blood vessels. The heart has two separate dual pumps that have different but integrated functions. Arteries and veins definitions are not determined by the levels of oxygen in their blood, but very simply by the direction the vessels carry blood; either to the heart or away from the heart. - **Arteries** - are vessels that carry blood away from the heart. - **Veins** - are vessels that carry blood toward the heart. **Circulatory Pathways (Pulmonary and Systemic Circulation)** The blood vessels of the body are functionally divided into two distinctive circuits: pulmonary circuit and systemic circuit. The pump for the pulmonary circuit, which circulates blood through the lungs, is the right ventricle. The left ventricle is the pump for the systemic circuit, which provides the blood supply for the tissue cells of the body. C:\\Users\\hp\\Downloads\\download (33).jpeg **Pulmonary Circuit** - Pulmonary circulation transports oxygen-poor blood from the right ventricle to the lungs, where blood picks up a new blood supply. Then it returns the oxygen-rich blood to the left atrium. - It includes a network of arteries, veins, its function to exchange blood and other tissue fluids between the heart, the lungs, and back. They are designed to perform ventilation and gas exchange. - The system can be divided into the following components: - - - It is often termed the **\"right side\"** of the heart, **a low pressure, low resistance system** Illustration of the systemic circuit **The systemic circulation** - Often it is termed the **\"left side\"** of the heart. This circuit starts at the Left Ventricle (which is the Pump for the Systemic Circuit) and ends at the Right Atrium, which is the receiving room for the deoxygenated blood returning from the body. - It provides the functional blood supply to all body tissue. It carries oxygen and nutrients to the cells and picks up carbon dioxide and waste products. - Systemic circulation carries oxygenated blood from the left ventricle, through the arteries, to the capillaries in the tissues of the body. From capillaries, the deoxygenated blood returns through a system of veins to the right atrium of the heart. - The continuous movement of blood throughout the body is driven by the pumping action of the left ventricle causing blood flow downhill pressure gradient. - It is called a **high pressure, high resistance system,** - The system can be divided into the following components: - - - **Major Systemic Arteries** The aorta is divided into three portions: ***ascending aorta, aortic arch, and descending aorta.*** The descending aorta is further subdivided into the thoracic aorta and abdominal aorta. - **The *coronary*** arteries are the only vessels that branch from the ***ascending aorta***. The brachiocephalic, ***left common carotid*** and left subclavian arteries branch from the ***aortic arch.*** - Blood supply for the brain is provided by the ***internal carotid*** and vertebral arteries. - The subclavian arteries provide the blood supply for the upper extremity. The celiac, superior mesenteric, suprarenal, renal, gonadal, and inferior mesenteric arteries branch from the **abdominal aorta** to supply the abdominal viscera. - Lumbar arteries provide blood for the muscles and spinal cord. - Branches of the external iliac artery provide the blood supply for the lower extremity. The internal iliac artery supplies the pelvic viscera. **Major Systemic Veins** After blood delivers oxygen to the tissues and picks up carbon dioxide, it returns to the heart through a system of veins. The capillaries, where the gaseous exchange occurs, merge into venules and these converge to form larger and larger veins until the blood reaches either **the superior vena cava or inferior vena cava**, which drain into the right atrium. **Fetal Circulation** - Most circulatory pathways in a fetus are like those in the adult but there are some notable differences because the ***lungs, the gastrointestinal tract, and the kidneys are*** ***not functioning before birth***. The fetus obtains its oxygen and nutrients **from the mother** and also depends on maternal circulation to carry away the carbon dioxide and waste products. The umbilical cord contains *two umbilical arteries to carry fetal blood to the placenta and one umbilical vein to carry oxygen-and-nutrient-rich blood from the placenta to the fetus.* The ductus venosus allows blood to bypass the immature liver in fetal circulation. The foramen ovale and ductus arteriosus are modifications that permit blood to bypass the lungs in fetal circulation.  **Cardiac Muscle and the Heart** In the heart, there are two major types of cardiac muscle cells or myocardiocytes. There are **1) Auto rhythmic cardiomyocytes**, these are myocardial conducting cells that generate their own action potential and coordinate the sequence of the heartbeat. **2) Contractile cardiomyocytes**, these are the myocardial contractile cells that make up most of the cells in the atria and ventricles and contract to generate force. Auto rhythmic cells are specialized cells. Contractile cells are cells that cannot generate their own action potential but cause mechanical contraction. **1) Auto rhythmic cardiomyocytes** - These cells are strategically located throughout the heart to initiate, conduct and facilitate the electrical signals that orchestrate the heartbeat. - About **[1%]** of myocardial cells are **auto rhythmic** and they spontaneously and rhythmically generate their own action potentials (APs) without nervous stimulation. In this way, control of heart activity is considered to be within the heart itself, and it is called ***intrinsic myogenic control***, it is derived from within the cardiomyocytes. - In cardiac muscle, input from the autonomic nervous system (**ANS**) and **hormones** from endocrine glands can ***modify** the contraction rate* set by the pacemaker cells and can ***modify** the force of contraction*. [However, the heart will contract in the absence of all neural input]. **N.B** In fact, if a healthy heart is removed from a body and supplied with O2 and nutrients, it will continue contract, and at a **higher rate** since the resting parasympathetic modulation which keeps the heart rate lower at rest is removed. **2) Contractile cardiomyocytes** - These cells Contract to generate force and represents only about **99%** of myocardiocytes, these cells are branched, with striations, centrally located nuclei, many blood vessels and they are all connected together with intercalated discs. - A characteristic of cardiac muscle are that they contain **intercalated disks**, which are interdigitated membranes joined by ***desmosomes*** and ***gap junctions***. **Gap junctions** are simply protein channels connecting adjacent **cardiomyocytes**, they allow ions (predominantly Na+) to pass though and thus waves of depolarization to spread throughout the muscle tissue - creating nearly simultaneous contraction. C:\\Users\\hp\\Downloads\\Intercalated disc.jpeg This allows the cardiac muscle acts as **a syncytium**. There are two syncytia in the heart: atrial and ventricular. **The** **atrial** **syncytium** is separated from **the ventricular syncytium** by a fibrous ring that surrounds the AV valves. The cardiac action potential is transmitted from the atria to the ventricles only through **the AVN** which is a part of the excitatory and conductive system of the heart. **Excitation-Contraction Coupling in Cardiac Muscle is Similar to Skeletal Muscle** Contraction occurs by the same sliding filament activity as in skeletal muscle. However, an important *difference* (**Sources of Ca2+**) is that in **cardiac** contractile muscle cells, the AP opens membrane voltage-gated Ca2+ channels. This entry of Ca2+ from the **ECF- (10%**) is required to release its internal Ca2+ stores in the sarcoplasmic reticulum (SR) so it is called "Ca2+- induced Ca2+ release" **CICR** --(**90%**). **How is this organized?** **Step by Step of Ca2+-Induced Ca2+ Release Process** As the action potential comes down the T-tubules, ①it triggers the opening of the **voltage gated Ca2+ channels**; there is an influx of Ca2+ **downhill**. The Ca2+ that enters the cardiomyocytes from the ECF binds to **ryanodine receptors** on the SR, ②which are linked mechanically to the gates on the SR, triggering them to open. Since the concentration of Ca2+ in the SR is very high, Ca2+ rushes out of the SR into the ICF (sarcoplasm) of the contractile cardiac muscle cell. ③This causes a **'spike'** in the intracellular of Ca2+ concentration, Ca2+ diffuse and move to the sarcomere ④Where they bind with the regulatory protein **troponin**. Therefore, the troponin that sits on top of the other regulatory protein **tropomyosin** causes it to move which allows the **actin myosin cross-bridge cycling** to occur promoting **contraction and generating force**. **On relaxation**. The removal of free Ca2+ from the cytosol requires the constant activity of the **Ca2+-ATPase** pump on the SR (**SERCA**) ⑤which actively re-uptake **Ca2+** back into the SR, also removal of Ca2+back to the ECF is achieved by Na+/Ca2+ secondary active transporter ⑥, which is sometimes called the **Na+/Ca2+ exchanger**. This is an antiport membrane transporter that moves a single Ca2+ out of the cell (uphill) and at the same time imports 3 Na+ for each cycle. ATP is indirectly required. the ATP is being used directly by the **Na+/K+ pump** which creates and maintains the electrochemical gradient (thus energy is constantly being stored across the membrane). **The Stretch and Length-Tension Relationship** - Another property of cardiac muscle is that **when it is stretched, it contracts more forcefully**. This is due to the length-tension relationship. - The degree of overlap between thick and thin filaments of the sarcomere will affect the tension generated by that muscle cell. - For example by an *increase in venous return* to the heart will lead to lengthening to the *optimal sarcomere* length and can exert *a greater force*. - physical stretching of myocardial cells **opens mechanically gated (stretch-sensitive) Ca2+ channels**, more Ca2+ entry into the cardiomyocytes, which leads to a stronger contraction (more Ca2+= more force). - The degree of stretch of cardiomyocytes at any time depends on blood volume (venous return) when filling is occurring.  **Starling's Law of the Heart** - Stroke volume is the volume of blood that is ejected from a ventricle (left or right) in one beat. - If the force of contraction of a ventricle increases, stroke volume will also increase. - Starling's law of the heart expresses the relationship between **stroke volume** (force of contraction) **and end diastolic volume** (EDV= VR=initial length). - This law *states that, within limits; stroke volume increases in response to an increase in the volume of blood in the ventricles just before contraction* (if all other factors are constant). **Automaticity and Rhythmicity of the Heart** **Automaticity** is the ability of the heart to initiate its own contraction independent of external stimuli. **Rhythmicity (chronotropy)** means that the heart can beat regularly. Both automaticity and rhythmicity are due to the pacemaker action potentials that are generated spontaneously and regularly by Pacemaker cells which are present in SA node, AV node and Purkinje fibers. **Action Potentials in Auto rhythmic cardiomyocytes (slow response)** - The **pacemaker** ability of **auto rhythmic** cells results from an ***unstable membrane potential** (**diastolic prepotential or pacemaker potential**)*. It starts at **-60** mV and when it reaches the ***threshold*** value of **-40 mV**, the cell ***fires*** **an AP**. - It is caused by **\"funny\" channels** that are permeable to Na+, ca2+ and not **k+**, at -60 mV this channel allows allow (Na+ in and ca2+ into the cell through **transient Ca channels** , at the same time **close leaky k+ channels** and this induces a **current flow**. - The opening of the funny channels creates a net influx of positive charge, when it depolarizes (partial dep.) the membrane to **threshold**; this then closes these funny channels, and opens ***long lasting Ca2+ channels*** causing rapid Ca2+ influx and the ***depolarization phase***. - At the peak of the action potential, the Ca2+ channels close and K+ channels open. The ***efflux of K+ causes*** the ***repolarization phase***. **Control of heart rhythmicity by cardiac nerves** There is a balance of forces from both divisions of the ANS: 1. **Sympathetic stimulation** of these pacemaker cells **→** release **NE** on **β1-adrenoreceptors →** generation of **c-AMP** → **increase in funny current** **→** increasing the **Ca2+ influx** which **reduces the repolarization phase** and **speeds up the depolarization phase**. This increases the rate of APs the (**increases the heart rate= tachycardia=positive chronotropy)** 2. **Parasympathetic stimulation** by Vagal nerve terminals → release **Ach** → binds to **M2** receptors → decreased c-AMP → **decrease in funny current** → **increasing the K+ efflux and decreasing the Ca2+ influx** → **create hyperpolarization** → slowing of the AP rate (**slowing** of the heart rate**=** **bradycardia = negative chronotropy.** **Other factors affecting excitability and rhythmicity of the heart** Effect of potassium ions: - **Hyperkalemia causes bradycardia,** *excess **potassium*** in the ECF makes the RMP less negative and dangerous ***arrhythmia*** can occur easily. - **Hypokalemia causes tachycardia** - High potassium levels between **8 and *12 meq/L*** can **slow** the heart due to prolonged repolarization decreasing both the heart rate and the rate of conduction in the AV bundle and ***may lead to diastolic arrest***. - **Effect of calcium ions and temperature** - ***Excess calcium*** ions in the extra-cellular fluid ***decrease the cardiac excitability*** and decrease calcium ions increases excitability. - ***High temperature*** as in fever ***increases excitability and increases heart rate*** while cold slows the heart. The effect of temperature is explained by the effect of temperature on the [rate of diffusion of different ions]. - For every one degree rise; heart rate increases by about 10 beats/min. - **Ca++ channel blocking drugs:** some types of these drugs cause bradycardia by inactivation of L-type Ca++ channels in the heart. Rhythmicity of various parts of the heart \(1) S.A.N has the highest rate; about 90 impulses / min. \(2) A.V.N: about 70 impulses / minute \(3) A.V bundle: 45 impulses / minute \(4) Purkinje fibers: 35 impulses / minute \(5) Ventricular muscle fibers have a rate about 25 impulses / minute; this is called idio-ventricular rhythm. When the S.A.N is injured, the next highest rhythm (A.V.N) becomes the pacemaker, and the rhythm is called A.V. nodal rhythm, and there will be cardiac slowing. **Action Potentials in contractile cardiomyocytes (fast response)** - In **contractile** myocardiocytes, there is a stable resting membrane potential of **-90 mV and called phase 4**. These cells require a stimulus to reach **threshold** (which is **-70 to -65 mV)**. - Reaching threshold triggers the rapid **depolarization phase (phase 0)** which is due to the entry of **Na+** through the **very fast opening voltage gated Na+ channels**. - At the peak of the AP (about **+20 mV**) these fast Na+ channels close and **voltage gated K+ channels** open, the efflux of K+ brings the membrane down to about **+5 mV (early repolarization or Phase 1)**, - Then the **long lasting Ca2+ channels** **voltage gated Ca2+ channels open** and this keeps the membrane elevated at about +5 mV for a very long **plateau or Phase 2.** - When theses Ca2+ channels close and coupled with **K+ efflux** which is responsible for the **rapid(phase 1)** and **steep (slow= phase 3)** **repolarization** **phase** that takes the membrane back down to **-90 mV**, where **RMP** is restored.  **Maintenance of ionic concentrations in cardiomyocytes:** When an action potential is generated, more Na+ & Ca++ enter the cell & more K+ leaves; this will be homeostatically maintained by ionic pumps & exchangers in sarcolemma, which include: **① Na+-K+ ATPase** that pumps 3Na+ out of the myocyte and pumps 2K+ in. **② Ca++ - ATPase** pumps Ca++ out of the myocyte. **③ Na+-Ca++ exchanger** that exchange 3 Na+ for 1 Ca++. It operates in both directions depending on membrane potential and concentration gradient for ions: - If excess Ca++ ions are present inside the myocyte, it moves Ca++ out and brings Na+ in. - If excess Na+ ions are present inside the myocyte, (e.g. by inhibition of Na+-K+ ATPase by **[digitalis]**), it moves Na+ out and brings Ca++ in. **Excitability changes during action potential (Fig)** **❶ Absolute Refractory Period (ARP):** Cardiomyocytes are refractory to initiation of new action potentials (unexcitable) because the inactivation gates of fast Na+ channels are still closed. It includes phases 0, 1, 2, and part of phase 3 (till the membrane is repolarized to about -50 mv). **❷ Relative Refractory Period (RRP):** It follows the absolute refractory period and continues till the membrane potential repolarizes to about -75 mv. A [supra-threshold stimulus] elicits an action potential. **❸ Supernormal period:** It follows RRP until the membrane is fully repolarized (late part of phase 3). During this period the myocyte can respond to [a weaker stimulus]. It is called [vulnerable period] because many cardiac arrhythmias can be initiated during this period. https://i.pinimg.com/564x/2a/0a/0f/2a0a0fc89340211adab71ba74fafcc70.jpg - N.B cardiomyocytes action potential (AP) is lengthened compared to skeletal muscle AP, due to Ca2+ entry that creates the ***elongated plateau** phase* before repolarization. This creates a **very long AP** for these cardiomyocytes. The typical skeletal muscle AP duration is **1-5 msec**, but the time taken for a contractile myocardial AP is about **200** **msec** in atrial muscle fibers and for about **300** **msec** in ventricular muscle fibers. Most of the duration of this AP is also the ***absolute refractory period***. no other AP can occur during an absolute refractory period, so this helps to *[prevent any kind of temporal summation]*, and consequent **tetanus contraction**, which would be incompatible with the heart as a pump, as it **prevents the effective filling of the heart's chambers.** **The sympathetic division (SYM)** acts on the SA Node, the AV node and the contractile cardiomyocytes to increase both heart rate **(+ve chronotropic)** and forces of contraction **(+ve inotropic)** and conductivity **(+ve dromotropic**) of the heart in times of emergencies. In contrast, the parasympathetic division (PARA) acts predominantly on the SA Node to decrease the heart rate (beats/min). **Extra systole** When a stimulus is applied during diastole, the heart contracts because, diastole is the relative refractory period. This contraction of the heart is called ***extra systole** or **premature contraction*****.** Extra systole is followed by the stoppage of heart in diastole for a while. This diastole is longer than the diastole after regular contraction. Temporary stoppage of the heart before it starts contracting is called ***compensatory pause*.** ***Duration*** of extra systole and compensatory pause is *equivalent* to the duration of ***two cardiac cycles*.** ***Cause for compensatory pause*** A natural impulse from SAN arrives at the time of contraction period of extra systole. As this period is absolute refractory period, the *natural impulse cannot cause contraction* of heart. And the heart has to wait for the arrival of next natural impulse from SAN. Till the arrival of next impulse, the heart stops in diastole. **Electrical Conduction System of the Heart (chronotropy)** The impulse discharged by the **SAN** is conducted through the atria in two pathways: a\) From SAN to left atrium via **inter-atrial tract (Bachmann's bundle)** then to the right atrium. The two atria are stimulated simultaneously because of the very rapid conduction velocity through the atrial muscles. b\) From SAN to AVN via **the inter-nodal tracts** (anterior, middle and posterior) AVN is located on the right side of the inter-atrial septum at the junction of the atria and ventricles it is the **only normal path** for excitation wave from atria to ventricles. The conduction velocity is extremely slow (from 0.02 to 0.05 m/sec). This causes a delay of conduction from atria to ventricles for about 0.1 sec. - **Causes of AV nodal delay** a. More negative membrane potential b. Great resistance to the conduction of excitatory ions from one cell to the next due to the presence of few gap junction connections. - **Physiological importance of the A-V nodal delay** a. It gives a chance for complete ventricular repolarization. b. It gives a chance for adequate ventricular filling. c. It protects the ventricles against supra-ventricular high rhythm as atrial fibrillation.  **\ ** - The excitation wave then travel rapidly through the AV bundle, bundle branches at a rate of **about 1 m/sec**. - In case of *congenital or acquired loss of one of the right or left bundle branches* the impulse spreads from one ventricle to the other by **muscle to muscle conduction**. - **Purkinje** **fibers** and the ventricular muscle fibers: The rate is **4-5 meters / sec**. This rapid rate of conduction means that the excitation wave reach all parts of the ventricles at the same time, leading to contraction of the ventricles as **one unite** (**syncytium**). **Important Note**: The main purpose of the electrical conduction system of the heart is to **orchestrate** (like a conductor of a symphony) the perfect Heart Beat. Therefore, *all electrical events precede (come before) any mechanical events in the heart.* ***All-or-none law*** *All-or-none law is applicable to whole cardiac muscle. It is because of syncytial arrangement of cardiac muscle. In the case of skeletal muscle, all-or-none law is applicable only to a single muscle fiber.* **Cardiac Output is a Measure of Cardiac Performance** Cardiac Output (**CO**) specifically is the ***volume*** of blood pumped by each ventricle ***per one minute***. - Left and Right ventricular volumes should be ***equivalent***. **CO** is measured in **L/min** for each ventricle. It is calculated using the formula: - - - - so, - **Ejection fraction:** the percentage ratio of the S.V to E.D.V **Sample Calculation of Cardiac Output!** To calculate CO we will use typical values for a 70 Kg (150-lb) adult male at rest, with HR of **72** beats/min; If we use the values given in the cardiac cycle describe above, we can calculate SV. If EDV = 135 ml and ESV = 65 ml, then SV = EDV - ESV; =\> 135 ml -- 65 ml = **70** ml/beat. So; C.O. = 72 beats x 70 ml = **5,040 ml/min**, or **5.0 L/min** - Normally, both sides have equal CO; if for some reason the CO\'s become unequal, for instance in **Congestive Heart Failure**, blood will pool behind the weaker side of the heart. This is not good as the increased vascular pooling creates an increase in venous pressure which causes **edema** and makes it even more difficult for the weaker side to catch up. **Normal heart rate** varies with age, sex, sleep, emotional state and physical training. Increase heart rate (up to limits) increases COP and decrease heart rate decreases COP. ***COP increases with heart rate increase up to 180 b/min.*** If heart rate increases ***up to 200 b/min***, this will affect the ***cardiac filling*** decreasing the stroke volume and COP. **Factors affecting heart rate** - **Heart rate is increased by:** 1\. Sympathetic stimulation. 2\. Muscular exercise. 3\. Increased venous return (by impulses from right atrium bain-bridge effect). 4\. Decreased blood pressure 5\. Mild to moderate pain. 6\. during emotions by impulses from higher centers. 7\. Increase body temperature increase HR By: - Direct stimulation of SAN. - **Heart rate is decreased by:** 1\. Parasympathetic stimulation. 2\. Sleep 3\. Severe pain 4\. Increased blood pressure: heart rate is inversely proportional to blood pressure (**Mary's law**). 5\. Decreased body temperature (hypothermia). 6\. in athletes due to cardiac hypertrophy. **Effect of changes in heart rate on cardiac output** **①** Changes in heart rate are quantitatively more important than changes in stroke volume. In untrained person, HR can increase during muscular exercise by 100% to 200% while SV may increase by less than 50%. **②** Heart rate is mainly controlled by autonomic nerve supply to the heart. **③** Changing HR alone (by artificial pacemaker or during arrhythmias) from 70 to 140 beat/min does not double CO as expected. This is because doubling HR alone (from 70 to 140 beat/min) causes the SV to decrease to half its value and the CO will not change. [The decrease in SV is due to shortening of diastole (ventricular filling).] **④** If the HR alone is increased **above 180/min, CO will decrease** because the SV will be markedly decreased and the high HR will not compensate for the decrease in SV. **⑤** If the HR alone slows down to **below 60/min, CO will also decrease** because the increase in SV is limited by ventricular capacity & can\'t compensate for the low HR. **⑥** In normal physiological conditions (**exercise**), HR will double and lead to more than double CO. This is because SV also increases by physiologic mechanisms. **Factors affecting Stroke Volume** - Stroke volume isn\'t constant; it is homeostatically regulated and adjusted to the *different needs of the body* moment by moment. It can decrease when you are at rest and increase greatly during exercise. - A mean SV is **about 70 ml (from 55--100 ml) (**for one ventricle**.** - There are three main factors that influence stroke volume, and they are **contractility**, **preload,** and **afterload**. - SV is *directly related* to the **force generated by cardiac muscle during contraction**; this force is affected by 2 parameters: - - **Length-Tension (Stretch-Force) Relationships - Starling\'s Law of the Heart** - Stretch and force are related by the **Frank-Starling law of the heart**: "**within limits stretch of the myocardium (sarcomere length) is proportional to EDV",** which means the more stretched the cardiac muscle cells by more blood volume in the ventricles, the greater the force of contraction and the greater the stroke volume. **Preload or Venous Return (**affects mainly **EDV)** - - - - **Several Factors Affecting Venous Return** 1. **Skeletal Muscle Pump** - The skeletal muscle pump is created by skeletal muscle contractions all over the body. It becomes critically important when standing for long periods of time, as venous return from the lower limbs struggles to move up towards the heart. - Large veins have **venous valves**, when they are compressed, the blood flows in only one direction - toward the heart. - The large veins of the leg are embedded in skeletal muscle and when these skeletal muscles contract, its squeezes and compresses the low pressure veins, this pushes venous blood in the only direction it can flow -- toward the heart. 2. **Respiratory Pump** - The respiratory pump is another factor that augments venous return. Breathing in and out causes oscillatory changes in thoracic and abdominal pressures. - [During inspiration] there is a **decrease in pressure** in the thoracic cavity and therefore a [decrease in pressure in thoracic veins(**suction**)]. At that moment, the abdominal veins below them have higher pressure than the blood in the thoracic veins (pumping). This promotes greater blood flow (\'down\' its pressure gradient) towards the heart. - [During expiration] there is an **increase in pressure** of the thoracic cavity (and in thoracic veins), taking away this temporary favorable pressured gradient. However, the venous valves prevent blood from dropping too far back. The result is that the lower thoracic pressure draws more blood in from the abdominal veins intermittently. 3. **Sympathetic Activity** - 4. **Atrial suction force** - - **N.B according to Starling's Law of the Heart;** the greater the stretch of the ventricular muscle (within limits), the more powerful the contraction is, which in turn increases SV. Therefore, by increasing **preload**, you increase the second variable, **contractility**. **Cardiovascular Afterload** (affects mainly **ESV**) - Afterload refers to the force the ventricles must develop in order to pump blood effectively **against the peripheral resistance in the vascular system**. *Any condition that increases resistance requires a greater afterload* to force open the semilunar valves and pump the blood into the arteries. - *[If afterload increases]*, the heart must generate more force in order to overcome the increased resistance. - - - *[If afterload decreases]* for example, a decrease in peripheral resistance (lower blood pressure) this factor decreases ESV, and therefore increases SV. **Heart Contractility (inotropic state** affects mainly **ESV.)** - Definition : It is the contractile force of the heart *independent of preload and afterload* - It is the primary factor impacting **ESV**. The more forceful the contraction, the more blood is ejected from the heart, thus the greater the SV and less blood remain in ventricle (lower ESV). - The term inotropic describes heart contractility. Positive inotropic factors increase contractility, and negative inotropic factors decrease contractility. - It depends upon the **availability** **of Ca2+** in the cardiomyocytes, the more Ca2+ the stronger the force! - Higher concentrations of intracellular Ca2+ increase the strength of contraction. ***Excess Ca2+ above normal*** (**hypercalcemia**) also acts as a positive inotropic agent and may **stop the heart in systole.** - The drug **digitalis** is given to patients with **congestive heart failure** because it lowers HR but ***increases the force of contraction and its mechanism of action***. - It ***slows the Na+/K+ pump*** (more Na inside will be exchanged by Ca from ECF by Na/Ca exchanger) and ***slows the reuptake of Ca2+*** into the SR, - This leads to higher free intracellular Ca2+ levels, greater force of contraction, and therefore greater stroke volume, - This helps the side of the heart that is lagging behind to catch up. - In addition to NE and E from the adrenal medulla, other hormones also demonstrate **positive inotropic effects**, including **thyroid hormones and glucagon from the pancreas.** +-----------------------+-----------------------+-----------------------+ | **Major Factors | | | | Affecting Heart Rate | | | | and Force of | | | | Contraction** | | | +-----------------------+-----------------------+-----------------------+ | **Specific** | **Effect: Increases | **Effect: Decreases | | | Heart Rate and** | Heart Rate and Force | | **Factor** | | of Contraction** | | | **Force of | | | | Contraction** | | +-----------------------+-----------------------+-----------------------+ | **Sym.** Accelerator | Release of | \-\-\-\-- | | nerves | norepinephrine | | | | | Release of | | **Para.** Vagus nerve | \-\-\-\-- | acetylcholine | +-----------------------+-----------------------+-----------------------+ | **Proprioceptors** | Increased rates of | Decreased rates of | | | firing **during** | firing **following** | | | exercise | exercise | +-----------------------+-----------------------+-----------------------+ | **Chemoreceptors** | Decreased levels of | Increased levels of | | | O2; | O2; | | | | | | | Increased H+, CO2, | Decreased levels H+ | | | lactic acid | and CO2 | +-----------------------+-----------------------+-----------------------+ | **Baroreceptors** | Decreased rates of | Increased rates of | | | firing, indicating | firing, indicating | | | **falling** blood | **higher** blood | | | volume/pressure | volume/pressure | +-----------------------+-----------------------+-----------------------+ | **Limbic System** | **Anticipation** of | **Anticipation** of | | | physical Exercise or | relaxation, **calm** | | | **strong** | **Emotions** | | | **Emotions** | | +-----------------------+-----------------------+-----------------------+ | **Catecholamines** | Increased NE and E | Decreased NE and E | +-----------------------+-----------------------+-----------------------+ | **Thyroid Hormones** | Increased T3 and T4 | Decreased T3 and T4 | +-----------------------+-----------------------+-----------------------+ | **Calcium** | Increased Ca2+ | Decreased Ca2+ | +-----------------------+-----------------------+-----------------------+ | **Potassium** | Decreased K+ | Increased K+ | +-----------------------+-----------------------+-----------------------+ | **Body Temperature** | Increase in Body | Decrease in Body | | | Temperature | Temperature | +-----------------------+-----------------------+-----------------------+ | **Nicotine** and | Stimulants, increased | \-\-\-\-- | | **Caffeine** | heart rate | | | | | Calming, decreased | | **Saturated Fats** | \-\-\-\-- | heart rate | | and **nuts** | | | +-----------------------+-----------------------+-----------------------+ **Cardiac Function Curves:** - This curve describes the **relationship** between right atrial pressure (**RAP**) and cardiac output (**CO**) (Fig). - This curve is an expression of the Frank-Starling\'s law. It shows that increasing RAP leads to increase in cardiac output. **RAP represent preload** because it is the pressure that fills the ventricle to its EDV. - This relationship represents a mechanism that can increase cardiac output independent of any external nervous or hormonal stimulation, i.e. it is an **intrinsic** mechanism (**heterometric auto regulation**). - But **this mechanism has limits**. The curve reaches a plateau at **13 L/min** (2.5 times CO). The heart can pump a venous return that is 2.5 times more than normal. More VR and RAP cannot cause additional increase in cardiac output without external nervous or hormonal stimulation. **The slope** of the cardiac function curve **increases if:** - Heart rate increases (sympathetic stimulation) - Inotropy increases (sympathetic stimulation or other positive inotropes) - Afterload decreases (peripheral vasodilation & decreased arterial blood pressure) - Under such conditions, higher CO is achieved for same RAP. Maximum CO **can now exceed 13 L/min** and called **\"hyper-effective heart\".** **The slope of the relationship decreases if:** a\. Heart rate decreases (parasympathetic stimulation). b\. Inotropy decreases (myocardial ischemia). c\. Afterload increases (hypertension). **hypo -effective heart** Any factor that decreases cardiac pumping power can make the heart hypo-effective as: 1\. Coronary artery disease: due to ischemia of cardiac muscle 2\. Cardiac arrhythmias: due to irregular rate of contraction 3\. Valvular heart disease: stenotic valve diseases decreases COP 4\. Congenital heart diseases 5\. increased arterial blood pressure: due to increases after load 6\. Myocarditis: inflammation of cardiac muscle 7\. Cardiac hypoxia due to any cause of generalized hypoxia. **Hyper-effective heart** **Causes:** 1. Nervous Excitation: combination of sympathetic stimulation and parasympathetic inhibition increases the pumping effectiveness of the heart by: - It greatly increases the heart rate---sometimes, in young people, from the normal level of 72 beats/min up to 180 beats/min. - It increases the strength of heart contraction to twice its normal strength. 2. Heart Hypertrophy - Long-term physiological **increased workload** causes the heart muscle to increase in mass and contractile strength in the same way that heavy exercise causes skeletal muscles to hypertrophy. - For example, the hearts of **marathon** runners may be increased in mass by 50% to 75%. This factor increases the cardiac output, sometimes 60% to 100%, and therefore allows the heart to pump much greater than the usual normal amounts. **CARDIAC CYCLE** It is the study of the changes which occurs in the heart during one cardiac systole and one diastole. **Duration** With a heart rate 70 beats/min. each cardiac cycle has duration of (0.8 sec) Ventricular systole = 0.3 sec, Ventricular diastole =0.5 sec **The duration of the cardiac cycle is inversely related to the heart rate**. When the heart rate increases duration of cardiac cycle is decreased and this decrease is *on the expanse of diastolic time (filling time*) which becomes shorter. **The 5 Phases of the Cardiac Cycle** 1. **Atrial and Ventricular Diastole**: The heart is at 'rest' and the atria and ventricles are relaxing. The atria are filling with venous blood. The AV valves are open as ventricles relax and blood flows by gravity from atria to ventricles. During this phase, the ventricles are about **80%** filled with blood, this is termed *passive filling*. *(T-P Segment of ECG)* 2. **Atrial Systole: Completion of Ventricular Filling** - When the atria contract (systole), the remaining **20%** of blood fills the ventricles *(active filling)*. Atrial systole coincides with last 0.1 sec of ventricular diastole. Atrial systole is called "late diastole". - Atrial systole begins following depolarization of the SA node, as a wave of depolarization (electrical signal) across the atria is followed by a wave of contraction that pushes blood into the ventricles to complete ventricular filling. - Some blood is forced back into veins, creating a small retrograde blood movement, measured as a pulse in the jugular vein. *(Atrial systole follows P wave on ECG)* - At this time, just before ventricular systole, the ventricles are full of blood; this is called **End Diastolic Volume (EDV**) and represents the **maximum ventricular volume**. At rest in a 70 Kg male, this value is typically **135 ml in each ventricle.** 3. **Early Ventricular Systole (part one) and the First Heart Sound** - Ventricular systole begins at the apex (bottom) of the heart squeeze blood upward toward the base. The increasing pressure of the blood in the ventricles forces the AV valves closed - creating the 1st heart sound (S1) the \"lub\" of \"lub dup\". (QRS complex on ECG) - Both ventricles are now **closed chambers** because now **both the AV and Semilunar valves are closed**. The ventricles are continuing to contract, but because all valves are closed, the blood has nowhere to go except to generate more pressure. The heart is in **Isovolumetric Ventricular Contraction**. - The blood volume inside the ventricles remains the same, but pressure is increasing. During this phase, the atria repolarize and relax as the ventricles continue to contract. 4. **Ventricular Systole (part two): (maximum and reduced Ejection)** - When ventricular contraction generates enough pressure, it opens the semilunar valves, and blood enters arteries (RV \> pulmonary trunk/artery, LV \> aorta). - The *high-pressured blood is forced* into the arteries creates a ***driving force*** which displaces lower-pressured blood creating blood movement. - Remember that each ventricle has **equivalent** blood volumes but different pressures. The RV requires a minimum of **25 mmHg** and the LV requires a minimum of **80 mmHg** to open the semilunar valves. - On reaching these pressures it creates the **pressure gradients** which drive blood flow out of the heart into arteries. - Just after ventricular systole, the blood volume remaining in the ventricles after ejection is termed **End Systolic Volume (ESV),** at rest is about **65 ml** per ventricle that remains in the heart after ejection. - We can calculate how much blood was ejected from the heart (called Stroke Volume, SV) = EDV - ESV 5. **Ventricular Diastole and the Second Heart Sound** a. In ventricular diastole, the ventricles relax and the pressure of the blood inside decreases. b. The blood in the large arteries leaving the ventricles falls back toward the heart as the ***driving force subsides***. c. This ***reversal*** of blood toward the heart fills the cusps of the semilunar valves, ***slamming the semilunar valves closed*** - creating the ***2nd heart sound, (S2) \"dup\"*** of \"lub dup\". Sudden closure of the aortic valve against the backward moving blood causes the blood to bounce upward due to elasticity of the aorta leading to expansion then elastic recoil which prevent acute drop in blood pressure. (T wave on ECG) - ***The AV valves still remain closed*** because ventricular pressure is still greater than the atrial pressure above it. Since the ventricles began to relax and the pressure decreased allowing the semilunar valves to close (to prevent backflow), now all heart valves are closed. ***Once again the ventricles are \'closed\' chambers***. - Now the ventricles are in **Isovolumetric Ventricular Relaxation.** This is a state where **[pressure is decreasing but volume remains constant].** - When ventricular pressure finally becomes less than atrial pressure, the ***weight of the blood*** in the atria ***opens*** the AV valves and blood moves into ventricles from the atria above. **Mechanical (Cardiac Cycle) and Electrical (ECG) Events** The mechanical events of the contraction and relaxation during the cardiac cycle **lag** slightly behind the electrical signals **(20 msec).** Atrial contraction begins really as the P wave ends and continues during P-R segment. Ventricular contraction begins just after Q wave and continues through S-T segment. **Table 14.2 The Electrical and Mechanical events for the various portions of a normal ECG.** +-----------------------+-----------------------+-----------------------+ | **Segment, Wave or | **Electrical** | **Mechanical** | | Interval** | | | | | **Event** | **Event** | +-----------------------+-----------------------+-----------------------+ | P-Wave | Depolarization of the | Late ventricular | | | atria. | diastole | | | | (relaxation). Passive | | | | filling of the | | | | ventricles (AV valves | | | | open). | +-----------------------+-----------------------+-----------------------+ | PR Interval | Reflects delayed | Atrial systole | | | conduction through AV | (contraction), top | | | node, or the 'AV | off of ventricular | | | nodal delay'. | volume (AV valves | | | | still open). | +-----------------------+-----------------------+-----------------------+ | QRS Complex | Depolarization of | Early ventricular | | | ventricles | systole | | | | (contraction). | | | | Closure of AV Valves | | | | (1st heart sound). | | | | Increased ventricular | | | | pressure | +-----------------------+-----------------------+-----------------------+ | ST Segment | No net electrical | Late ventricular | | | events. | systole. Opening of | | | | semilunar valves. | | | | Ejection of blood | | | | from ventricles into | | | | arteries. | +-----------------------+-----------------------+-----------------------+ | T-Wave | Repolarization of the | Early ventricular | | | ventricles. | diastole.Decreasing | | | | pressure, closure of | | | | semilunar valves (2nd | | | | heart sound). | +-----------------------+-----------------------+-----------------------+ | TP Segment | No net electrical | Ventricles continue | | | events. | to relax as pressure | | | | falls even lower, AV | | | | valves open. | +-----------------------+-----------------------+-----------------------+ +-----------------------+-----------------------+-----------------------+ | | **First Heart Sound | **Second Heart Sound | | | (S1)** | (S1)** | +-----------------------+-----------------------+-----------------------+ | Duration | Relatively long | Shorter duration | | | duration (0.15 sec.) | (0.12 sec). | +-----------------------+-----------------------+-----------------------+ | Timing | During isovolumetric | During isovolumetric | | | contraction and early | relaxation phase | | | | | | | ejection period | | +-----------------------+-----------------------+-----------------------+ | Cause | closure of mitral and | closure of aortic & | | | tricuspid valves | pulmonary valves | +-----------------------+-----------------------+-----------------------+ | Characters | Low pitched sound (30 | High pitched sound | | | Hz) as word "Lub". | (50 Hz) as word | | | | "Dup". | | | It has two | | | | components: | It has two | | | | components: | | | ❶ Mitral component: | | | | due to closure of | ❶ Aortic component | | | mitral valve. It is | due to closure of | | | best heard at the | aortic valve. It is | | | apex of the heart | best heard at the 2nd | | | (left 5th intercostal | right costo-sternal | | | space at | junction | | | midclavicular line). | | | | | ❷ Pulmonary | | | ❷Tricuspid component: | component**:** due to | | | due to closure of | closure of pulmonary | | | tricuspid valve. It | valve. It is best | | | is best heard at the | heard at 2nd left | | | lower end of the | intercostal space in | | | sternum. The 1st HS | parasternal line. | | | is normally split | | | | Mitral valve closes | Physiological | | | 0.04 sec before the | splitting of 2nd HS: | | | tricuspid valve. | Aortic valve closes | | | Mitral component | before pulmonary | | | occurs before | valve (left | | | tricuspid component. | ventricular ejection | | | | ends before right | | | | ventricular | | | | ejection). Splitting | | | | of 2nd sound | | | | increases and more | | | | audible during | | | | inspiration. | +-----------------------+-----------------------+-----------------------+ **The Third Heart Sound (S3):** - It occurs during **rapid filling phase** of the cardiac cycle. - It is due to **rapid flow of blood** from atria to ventricles. - It is not normally audible in adults but can be **audible normally in children.** **The Fourth Heart Sound (S4):** - It occurs during **atrial systole**. - It is due to rapid flow of blood from atria to ventricles. - It is very faint and is **not normally audible.** **Cardiac Output is a Measure of Cardiac Performance** Cardiac Output (**CO**) specifically is the ***volume*** of blood pumped by each ventricle ***per one minute***. - Remember that Left and Right ventricular volumes should be ***equivalent***. **CO** is measured in **L/min** for each ventricle. It is calculated using the formula: - **The heart rate** (**HR**) is contractions per minute (b**/**m). - **Stroke Volume** **(SV)** is the amount (volume) of blood pumped by one ventricle during a single contraction (ml/beat). It is calculated by the difference between End Diastolic Volume (**EDV:** maximum volume at the end of the resting for 'filling' phase of the ventricles) and End Systolic Volume (**ESV:** volume remaining in ventricles after contraction or 'ejection' phase of the ventricles), - so, **SV = EDV - ESV** - **Ejection fraction:** It is the percentage ratio of the S.V to E.D.V **Sample Calculation of Cardiac Output!** To calculate CO we will use typical values for a 70 Kg (150-lb) adult male at rest, with HR of **72** beats/min; If we use the values given in the cardiac cycle describe above, we can calculate SV. If EDV = 135 ml and ESV = 65 ml, then SV = EDV - ESV; =\> 135 ml -- 65 ml = **70** ml/beat. C.O. = 72 beats x 70 ml = **5,040 ml/min**, or **5.0 L/min** - Normally, both sides have equal CO; If for some reason the CO\'s become unequal, for instance in **Congestive Heart Failure**, blood will pool behind the weaker side of the heart. This is not good as the increased vascular pooling creates an increase in venous pressure which causes edema and makes it even more difficult for the weaker side to catch up. **Normal heart rate** varies with age, sex, sleep, emotional state and physical training. Increase heart rate (up to limits) increases COP and decrease heart rate decreases COP. ***COP increases with heart rate increase up to 180 b/min.*** If heart rate increases ***up to 200 b/min***, this will affect the ***cardiac filling*** decreasing the stroke volume and COP. **Factors affecting heart rate** **Heart rate is increased by:** 1\. Sympathetic stimulation. 2\. Muscular exercise. 3\. Increased venous return (by impulses from right atrium bain-bridge effect). 4\. Decreased blood pressure 5\. Mild to moderate pain. 6\. During emotions by impulses from higher centers. 7\. Increase body temperature increase HR By: - Direct stimulation of SAN. **Heart rate is decreased by:** 1\. Parasympathetic stimulation. 2\. Sleep 3\. Severe pain 4\. Increased blood pressure: heart rate is inversely proportional to blood pressure (Mary's law). 5\. Decreased body temperature (hypothermia). 6\. In athletes due to cardiac hypertrophy. **Effect of changes in heart rate on cardiac output** **①** Changes in heart rate are quantitatively more important than changes in stroke volume. In untrained person, HR can increase during muscular exercise by 100% to 200% while SV may increase by less than 50%. **②** Heart rate is mainly controlled by autonomic nerve supply to the heart. **③** Changing HR alone (by artificial pacemaker or during arrhythmias) from 70 to 140 beat/min does not double CO as expected. This is because doubling HR alone (from 70 to 140 beat/min) causes the SV to decrease to half its value and the CO will not change. [The decrease in SV is due to shortening of diastole (ventricular filling).] **④** If the HR alone is increased **above 180/min, CO will decrease** because the SV will be markedly decreased and the high HR will not compensate for the decrease in SV. **⑤** If the HR alone slows down to **below 60/min, CO will also decrease** because the increase in SV is limited by ventricular capacity & can\'t compensate for the low HR. **⑥** Under normal physiological conditions (**exercise**), HR will double and lead to more than double CO. This is because SV also increases by physiologic mechanisms. **Factors affecting Stroke Volume** - Stroke volume isn\'t constant; it is homeostatically regulated and adjusted to the different needs of the body moment by moment. It can decrease when you are at rest and increase greatly during exercise. A mean SV is about 70 ml (for one ventricle); **the normal range for SV is from 55--100 ml.** - There are three main factors that influence stroke volume, and they are **contractility**, **preload,** and **afterload**. - SV is *directly related* to the **force generated by cardiac muscle during contraction**; this force is affected by 2 parameters: - The length of muscle fiber at beginning of contraction - The amount of Ca2+ in the cardiomyocytes, the more Ca2+ the stronger the force! **Length-Tension (Stretch-Force) Relationships - Starling\'s Law of the Heart** - Stretch and force are related by the **Frank-Starling law of the heart**: "within limits stretch of the myocardium (sarcomere length) is proportional to EDV", which means the more stretched the cardiac muscle cells by more blood volume in the ventricles, the greater the force of contraction and the greater the stroke volume. **Preload or Venous Return (affects mainly EDV)** - The blood that returns to the heart from the venous circulation is termed \'**venous return**\' and - **The greater the venous return; the more forcefully the heart will contract.** This is because when the additional blood enters the heart, it stretches the cardiomyocytes and this in turn causes the cardiomyocytes to contract more forcefully. **Venous return determines EDV (EDP) and this determines stroke volume.** - **Preload can be increased** by increased blood volume (**hypervolemia**), physical activity (exercise), sympathetic stimulation (fight or flight) and an increase in the skeletal pump activity. **Several Factors Affecting Venous Return** 1. **Skeletal Muscle Pump** - The skeletal muscle pump is created by skeletal muscle contractions all over the body. It becomes critically important when standing for long periods of time, as venous return from the lower limbs struggles to move up towards the heart. - Large veins have **venous valves**, when they are compressed, the blood flows in only one direction - toward the heart. - The large veins of the leg are embedded in skeletal muscle and when these skeletal muscles contract, its squeezes and compresses the low pressure veins, this pushes venous blood in the only direction it can flow -- toward the heart. 2. **Respiratory Pump** - The respiratory pump is another factor that augments venous return. Breathing in and out causes oscillatory changes in thoracic and abdominal pressures. - [During inspiration] there is a **decrease in pressure** in the thoracic cavity and therefore a [decrease in pressure in thoracic veins]. At that moment, the abdominal veins below them have higher pressure than the blood in the thoracic veins. This promotes greater blood flow (\'down\' its pressure gradient) towards the heart. - [During expiration] there is an **increase in pressure** of the thoracic cavity (and in thoracic veins), taking away this temporary favorable pressured gradient. However, the venous valves prevent blood from dropping too far back. The result is that the lower thoracic pressure draws more blood in from the abdominal veins intermittently. 3. **Sympathetic Activity** - The **sympathetic** division of the ANS causes **constriction of veins** in the systemic circulation (**venomotor tone**); this will immediately move more blood into heart, so it increases venous return. 4. **Atrial suction force** - During rapid ejection phase of the cardiac cycle, the atrioventricular ring is pulled down. This leads to sharp drop of atrial pressure with subsequent sucking of the blood into the atria from the great veins. 5. **Venous pressure gradient:** **N.B** **Mean circulatory filling pressure** **(MCFP)** is the pressure that would be measured at all points in the entire circulatory system if the heart was stopped suddenly and the blood was redistributed instantaneously in such a manner that all pressures were equal, it is usually about **7-8 mmHg** - **EDV** is also affected by **ventricular filling (compliance and stiffness)**: The EDV is directly proportional to the former and inversely proportional to the latter). If Intrapericardial pressure e.g. as a result of fluid or blood accumulation (= cardiac temponade) decreases the EDV. - **Filling** occur during diastole and it is affected by diastole time (heart rate) and Passive relaxation property of the ventricles **DIASTOLIC (RELAXATION) PROPERTIES OF THE VENTRICLES** Ventricular relaxation is important for normal cardiac function (like ventricular contraction) since deficient relaxation also decreases the SV and CO (due to impairment of ventricular filling). The process of ventricular relaxation is determined by both active and passive processes: I. **Active relaxation property** of the ventricles: This refers to the relaxation that occurs as a result of ***ca+2 reuptake*** by the sarcoplasmic reticulum and is known as **lusitropy**. It is responsible for most of ventricular filling during early diastole, so positive lusitropic factors (e.g. most positive inotropic factors) increase ventricular filling. II. **Passive relaxation property of the ventricles:** This refers to the various **mechanical** factors that passively affect ventricular relaxation. These factors affect the extent of ventricular filling and include especially ventricular **compliance (=dispensability**) and **stiffness**. The extent of ventricular relaxation is decreased if ventricular compliance is decreased e.g. as a result of cardiac temponade (see above) or increased ventricular stiffness (as occurs in cases of infarction and hypertrophy).  **Cardiovascular** Afterload (**affects mainly ESV**) - Afterload refers to the force the ventricles must develop in order to pump blood effectively **against the peripheral resistance in the vascular system**. *Any condition that increases resistance requires a greater afterload* to force open the semilunar valves and pump the blood into the arteries. - *[If afterload increases]*, then the heart must generate more force in order to overcome the increases resistance that more afterload brings. - Damage to the semilunar valves, such as **stenosis** will **increase afterload**. - Afterload may also be raised due to **increased vascular resistance** (e.g., **hypertension**). - *[If afterload decreases]* for example, a decrease in peripheral resistance (**hypotension**) this factor decreases ESV, and therefore increases SV. **Starling's Law of the Heart** Starling's Law of the Heart states that, within physiological limits, the force of heart contraction is directly proportional to the initial length of the muscle fiber. This means that the greater the stretch of the ventricular muscle (within limits), the more powerful the contraction is, which in turn increases SV. Therefore, by increasing **preload**, you increase the second variable, **contractility**. **Heart Contractility (inotropic state affects mainly ESV = homeometric regulation of COP)** - Definition : It is the contractile **force** of the heart independent of preload and afterload - It is the **primary factor impacting ESV**. The more forceful the contraction, the **more blood is ejected** (pumped) from the heart, thus the greater the SV, and **less blood remains** in ventricle (lower ESV). - The term **inotropic** describes heart contractility. Positive inotropic factors increase contractility, and negative inotropic factors decrease contractility. - It depends upon the availability of Ca2+ in the cardiomyocytes, the more Ca2+ the stronger the force! - Higher concentrations of intracellular Ca2+ increase the strength of contraction. Excess Ca2+ above normal (**hypercalcemia**) also acts as a positive inotropic agent and may **stop the heart in systole.** - The drug **digitalis** is given to patients with **congestive heart failure** because it lowers HR but ***increases the force of contraction***. - It ***slows the Na+/K+ pump*** (more Na inside will be exchanged by Ca from ECF by Na/Ca exchanger) and ***slows the reuptake of Ca2+*** into the SR, - This leads to higher free intracellular Ca2+ levels, greater force of contraction, and therefore greater stroke volume, - This helps the side of the heart that is lagging behind to catch up. - In addition to NE and E from the adrenal medulla, other hormones also demonstrate **positive inotropic effects**, including **thyroid hormones and glucagon from the pancreas.** +-----------------------+-----------------------+-----------------------+ | **Table** | | | | | | | | **Major Factors | | | | Affecting Heart Rate | | | | and Force of | | | | Contraction** | | | +-----------------------+-----------------------+-----------------------+ | **Specific** | **Effect: Increases | **Effect: Decreases | | | Heart Rate and** | Heart Rate and Force | | **Factor** | | of Contraction** | | | **Force of | | | | Contraction** | | +-----------------------+-----------------------+-----------------------+ | **Sym.** Accelerator | Release of | \-\-\-\-- | | nerves | norepinephrine | | | | | Release of | | **Para.** Vagus nerve | \-\-\-\-- | acetylcholine | +-----------------------+-----------------------+-----------------------+ | **Proprioceptors** | Increased rates of | Decreased rates of | | | firing during | firing following | | | exercise | exercise | +-----------------------+-----------------------+-----------------------+ | **Chemoreceptors** | Decreased levels of | Increased levels of | | | O2; | O2; | | | | | | | Increased H+, CO2, | Decreased levels H+ | | | lactic acid | and CO2 | +-----------------------+-----------------------+-----------------------+ | **Baroreceptors** | Decreased rates of | Increased rates of | | | firing, indicating | firing, indicating | | | falling blood | higher blood | | | volume/pressure | volume/pressure | +-----------------------+-----------------------+-----------------------+ | **Limbic System** | Anticipation of | Anticipation of | | | physical Exercise or | relaxation, calm | | | strong Emotions | Emotions | +-----------------------+-----------------------+-----------------------+ | **Catecholamines** | Increased NE and E | Decreased NE and E | +-----------------------+-----------------------+-----------------------+ | **Thyroid Hormones** | Increased T3 and T4 | Decreased T3 and T4 | +-----------------------+-----------------------+-----------------------+ | **Calcium** | Increased Ca2+ | Decreased Ca2+ | +-----------------------+-----------------------+-----------------------+ | **Potassium** | Decreased K+ | Increased K+ | +-----------------------+-----------------------+-----------------------+ | **Body Temperature** | Increase in Body | Decrease in Body | | | Temperature | Temperature | +-----------------------+-----------------------+-----------------------+ | **Nicotine** and | Stimulants, increased | \-\-\-\-- | | **Caffeine** | heart rate | | | | | Calming, decreased | | **Saturated Fats** | \-\-\-\-- | heart rate | | and **nuts** | | | +-----------------------+-----------------------+-----------------------+ C:\\Users\\hp\\Downloads\\download (39).jpeg **Cardiac Function (COP) Curves:** - This curve describes the **relationship** between right atrial pressure (**RAP**) and cardiac output (**CO**) (Fig 56). - This curve is an expression of the Frank-Starling\'s law. It shows that increasing RAP leads to increase in cardiac output. **RAP represent preload** because it is the pressure that fills the ventricle to its EDV. - This relationship represents a mechanism that can increase cardiac output independent of any external nervous or hormonal stimulation, i.e. it is an **intrinsic** mechanism (**heterometric auto regulation**). - but **this mechanism has limits**. The curve reaches a plateau at **13 L/min** (2.5 times CO). The heart can pump a venous return that is 2.5 times more than normal. More VR and RAP cannot cause additional increase in cardiac output without external nervous or hormonal stimulation. **The slope of the cardiac function curve (enhanced) increases if:** - Heart rate increases (sympathetic stimulation) - Inotropy increases (symp. Stim. or positive inotropes) - Afterload decreases (peripheral V.D. & decreased ABP) - Under such conditions, higher CO is achieved for same RAP. Maximum CO **can now exceed 13 L/min** and called **\"hyper-effective heart\".** **The slope of the relationship decreases (depressed) if:** - Heart rate decreases (parasympathetic stimulation). - Inotropy decreases (myocardial ischemia). - Afterload increases (hypertension). **[Hypo-effective heart]** Any factor that decreases the cardiac pumping power can make the heart hypo-effective as: 1\. Coronary artery disease: due to ischemia of cardiac muscle 2\. Cardiac arrhythmias: due to irregular rate of contraction 3\. Valvular heart disease: stenotic valve diseases decreases COP 4\. Congenital heart diseases 5\. increased arterial blood pressure: due to increases after load 6\. Myocarditis: inflammation of cardiac muscle 7\. Cardiac hypoxia due to any cause of generalized hypoxia. **[Hyper-effective heart]** **Causes:** 3. Nervous Excitation: combination of sympathetic stimulation and parasympathetic inhibition increases the pumping effectiveness of the heart by: - It greatly increases the heart rate---sometimes, in young people, from the normal level of 72 beats/min up to 180 beats/min. - It increases the strength of heart contraction to twice its normal strength. 4. Heart Hypertrophy - Long-term physiological **increased workload** causes the heart muscle to increase in mass and contractile strength in the same way that heavy exercise causes skeletal muscles to hypertrophy. - For example, the hearts of **marathon** runners may be increased in mass by 50% to 75%. This factor increases the cardiac output, sometimes 60% to 100%, and therefore allows the heart to pump much greater than the usual normal amounts. **Cardiac Reserve** It is the **maximum percentage increase in cardiac output** that can be achieved above normal in response to increased body demands. - In normal young adult cardiac reserve is 300% - 400%. Cardiac output can be increased (maximal exercise) from resting value of 5 L/min to 20-25 L/min. - In well-trained athletes, cardiac reserve can be increased 600% (35 L/min) or even more. - In elderly people, cardiac reserve decreases to 200% or even less. - In heart failure, cardiac reserve may decrease down to zero%. **Cardiac reserve depends on the following mechanisms:** 1. **Heart rate reserve**: - The maximal heart rate that can be attained during maximal exercise can be estimated by: **Maximal heart rate = 220 - age in years**. - This equals approximately 200 beats/min in normal young adults. The normal resting heart rate is about 75 /min. So, there is a reserve for the heart rate to increase during exercise from 75 to 200 per minute. 2. **Stroke volume reserve**: - This can be achieved by: - ***Increasing EDV*** (Frank-Starling\'s mechanism). - ***Decreasing ESV*** (by sympathetic stimulation or other positive inotropic stimuli). 3. **Increased size of the heart (cardiac hypertrophy):** a. ***Eccentric hypertrophy***: In well-trained athletes who perform endurance exercise (long-distance runners) the [heart mass increases by about 50-60%.] This is accompanied by increased ventricular EDV. It is also known as **\"[volume overload]** [ **hypertrophy**\"] that enables the heart to pump greater stroke volume. b. ***Concentric hypertrophy***: it occurs when the heart is exposed to prolonged high afterload (strength training, or untreated hypertension). It is also known as **\"pressure overload hypertrophy\"** where the thickness of the ventricle increases while ventricular volume is not increased. The ventricle can pump with greater strength without an increase in wall stress. However, ventricular compliance decreases (ventricle becomes stiffer) and ventricular filling may become deficient. **Electrocardiography (ECG)** **Electrocardiography & Electrocardiogram (ECG)** The ECG (EKG) is a recording of electrical fluctuations that represent sum of the electrical activity generated by the myocardial fibers during depolarization and repolarization. (**Not contractions**) These changes are detectable on the surface of the body because body fluids can conduct electrical current  There are two major components of an ECG: waves and segments. **Waves** appear as deflections above or below the baseline. **Segments** are sections of baseline between two waves. **Intervals** are combinations of waves and segments. - ECG provides information on heart rate and rhythm, conduction velocity, and even the condition of tissues in the heart. - The interpretation of an ECG begins with the following questions: - - - - - The more difficult aspects of interpreting an ECG include looking for subtle changes, such as alterations in the shape, timing, or duration of various waves or segments **ECG Recording** - The ECG can be recorded by using an active or exploring electrode connected to an indifferent electrode at zero potential (unipolar recordings) or by using two active electrodes (bipolar recordings).  Chest leads (unipolar) \ The Limb Leads (Bipolar) In the augmented limb leads, the negative electrodes are a combination of two electrodes allowing an amplification of the signal recorded by the positive electrode. (Compared to measurement against Wilson's central terminal) These electrode pairs are used to determine the heart vector in the frontal plane **Precordial (Chest) Leads** **Standard position of positive electrodes:** **V1** - the fourth intercostal space to the right of the sternum **V2** - the fourth intercostal space to the left of the sternum **V3** - directly between leads V2 and V4 (in the middle) - *The activity of the Rt ventricle and atria is better visible on these leads* **V4** - the fifth intercostal space in the midclavicular line **V5** - horizontally with V4 in the anterior axillary line **V6** - horizontally with V4 and V5 in the midaxillary line. - *The activity of the left ventricle is better visualized on these leads* **Unipolar leads**  **Limb electrodes are relocated to the trunk** aVL aVR  I II III aVF **Different leads \"view\" the heart from different angles.** **Vectoral Representation** A vector is an entity that has both magnitude and direction. At any given moment during cardiac cycle a vector may represent the net electrical activity seen by a lead. A vector is commonly visualized as an arrow: - The length of the shaft represents the magnitude of the electrical current - The orientation of the arrow represents the direction of the current flow - The tip of the arrow represents the positive pole of the electrical current - The tail of the arrow represents the negative pole of the electrical current **[Einthoven\'s Triangle]** The three standard limb leads (I, II, III) can be transported into an equilateral triangle called the **Einthoven\'s triangle** Walter Einthoven is the father of the modern ECG. He named the parts of the ECG and created "Einthoven's triangle".  Einthoven\'s Law: Lead II = Lead I + Lead III **Cardiac Vector** - The propagation of action potential in the heart cells is in a certain direction: **vectoral** - The common vector of depolarization waves is called **cardiac vector** (axis). Calculation of mean QRS vector in each lead: distances equal to the height of the R wave minus the height of the largest negative deflection in the QRS complex are measured **Reflection of depolarization waves to standard limb lead II** Depolarization moving toward an active electrode (+) in a volume conductor produces a positive deflection (R wave) Depolarization moving in the opposite direction produces a negative deflection (S wave)  **Repolarization moving opposite direction to active electrode** **Produce** positive deflection. (e.g., T wave) **Atrial depolarization and generation of P wave**  At each point along the electrical activity, a small charge separation exists in the extracellular fluid between polarized membranes (positive outside) and depolarized membranes (negative outside). Thus, the wave front may be thought of as a series of individual *electrical dipoles* (regions of charge separation). Einthoven's law: at any instant the potential in lead II is equal to sum of the potentials in leads I and III 25 **Ventricular depolarization and the generation of the QRS complex** The initial ventricular depolarization usually occurs on the left side of the intraventricular septum Produce a negative component on lead I, a small negative component on lead II, and a positive component on lead III, During **ventricular depolarization** the number of individual dipoles is greatest and/or their orientation is most similar Produce a large net cardiac dipole which produce **R wave**. **Ventricular depolarization and the generation of the QRS complex** Late activation of posterobasal portion of the left ventricle Produce an **S wave**, During the **ST segment**, all ventricular muscle cells are depolarized, there is no electrical activity moving through the heart tissue. 1. Atria begin to depolarize. 2. Atria depolarize. 3. Ventricles begin to depolarize at apex , atria repolarize. 4. Ventricles depolarize. 5. Ventricles begin to repolarize at apex. 6. Ventricles repolarize.  Normal Fast Slow Irregular  Amplitude: 1 mV = 10 small squares = 10 mm and paper speed: 25 mm/sec. Heart rate can be calculated: distance between two QRS complex (e.g.0.84 sec) heart rate per minute (60 / 0.84 = 71).  **The P-wave duration**; how long atrial depolarization takes. **The PR interval;** indicates how long it takes the action potential to conduct through the AV node before activating the ventricles. **The QRS duration; the** wave of depolarization to spread throughout the ventricles. **The QT interval;** indicates how long the ventricles remain depolarized and is thus a rough measure of the duration of the overall "ventricular" action potential. **The QT segment** gets shorter as the heart rate increases, which reflects the shorter action potentials that are observed at high rates. Plotting the mean electrical axis of the ventricles from two electrocardiographic leads (leads I and III).  Left axis deviation in a ***hypertensive heart** (hypertrophic left ventricle)* **COMMON MISCONCEPTIONS ABOUT THE ECG** - The PR interval is **NOT** measured from the P wave to the R wave. It is defined to be the time from the beginning of the P-wave to the beginning of the QRS complex. - The QT interval is **NOT** measured from the Q-wave to the T-wave. It is defined as the time from the beginning of the QRS complex to the end of the T-wave. - The P-wave (QRS complex) is **NOT** generated by the contraction of the atria (ventricles). It is generated by electrical activity (more specifically depolarization or activation) of the muscle. - Purkinje fiber cells are NOT nerve cells. Rather, they are specialized cardiac muscle cells. The Sino atrial node, atrioventricular node, bundle of His, and bundle branches are also made up of specialized cardiac muscle cells. ***a wave** -* atrial contraction ***c wave**-* ventricles begin to contract ***v wave**-* slow flow of blood into the atria **The Heart is a Secondary Endocrine Gland** The heart is known to release at least 2 hormones; **atrial natriuretic peptide** (**ANP**); and **brain natriuretic peptide** (**BNP**). The hormone ANP is secreted by cardiomyocytes in the walls of the **atria** in response to elevated blood pressure. The atria make the **ANP** (atrial for atria; natri- for Na+; and uretic for urine) and this hormone makes the renal system get rid of more sodium in the urine. The atrial cardiomyocytes have ***mechanoreceptors*** which ***stretch*** when there is an ***increase in atrial blood volume***. This triggers ***release of ANP***. The ANP travels to the ***kidneys*** and acts to ***increase the excretion of Na+ and water*** in the renal system. By doing this leads to a reduction in the extracellular fluid (ECF) volume in the body, and this ***lowers systemic blood pressure.*** The brain natriuretic peptide (BNP) is not made in the brain but by the cardiomyocytes of the **ventricles** of the heart. It has similar effects to ANP, though it is ***less intense*** and has a ***shorted half-life***. These two cardiac hormones, ANP and BNP, **lower blood pressure**. They do so by **inhibiting** the release of **renin** and **aldosterone**. In addition, ANP and BNP ***both promote vasodilation in arterioles, which lowers blood pressure***. **Blood Vessels: Resistance and Pressure** **Blood Vessels of the Body** The blood that is pumped by the heart is transported to and from the body by blood vessels. There are five basic types of blood vessels in the body. **1. Arteries**: Both large **elastic** and **muscular** arteries have 3 layers. Role is for **transporting** High Pressure Blood and **maintaining** Blood Pressure. **2. Arterioles**: Have 2 layers, including **vascular smooth muscle** (VSM), can exhibit large changes in diameter = '**Resistance**' vessels. **3. Capillaries**: Have only 1 layer, 1 cell thick, called endothelium, for **exchange**. **4. Venules**: Have 2 layers, for **collection** of blood from capillary beds. **5. Veins**: Have 3 layers and **venous valves**, for **return of Low Pressure blood** to heart.