Module 2 Notes PDF
Document Details
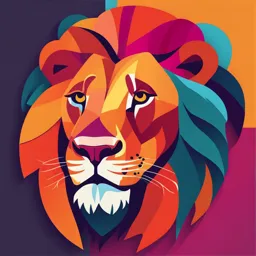
Uploaded by MagnanimousStonehenge
Tags
Summary
This document provides an overview of biomolecules, focusing on carbohydrates and their applications. It also covers cellulose-based water filters and their properties.
Full Transcript
Module 2 BIOMOLECULES AND THEIR APPLICATIONS 1.1 Carbohydrates: Carbohydrates are a class of organic compounds that play a crucial role in biology and are an important source of energy for living organisms. They are composed of carbon (C), hydrogen (H), and oxygen (O) atoms and are cl...
Module 2 BIOMOLECULES AND THEIR APPLICATIONS 1.1 Carbohydrates: Carbohydrates are a class of organic compounds that play a crucial role in biology and are an important source of energy for living organisms. They are composed of carbon (C), hydrogen (H), and oxygen (O) atoms and are classified based on their molecular structure and function. General formula is Cn(H2O)n. Monosaccharides These are the simplest form of carbohydrates and include glucose and fructose. They are easily soluble in water and serve as the primary source of energy for the body. Figure: Structural formula of glucose Figure: Ring structural formula of glucose, fructose, and galactose Disaccharides These are formed by the condensation of two monosaccharides and include sucrose, lactose, and maltose. They are commonly found in sugar and are broken down into monosaccharides during digestion. Figure: Structural formula of sucrose, lactose, and maltose Polysaccharides These are long chains of monosaccharides linked together. They serve as storage molecules for energy, such as glycogen in animals and starch in plants, and also provide structure and support, such as cellulose in plant cell walls. In addition to their role as energy sources, carbohydrates also play important roles in cellular processes, such as cellular signaling and recognition, and in regulating gene expression. Figure: Ring structural formula and line structural formula of starch Figure: Ring structural formula and line structural formula of cellulose Figure: Ring structural formula and line structural formula of glycogen Overall, carbohydrates are essential components of biological systems and play a crucial role in maintaining the health and survival of living organisms. Industrial Applications of Carbohydrates Carbohydrates have a wide range of applications in various industries, including: Food and Beverage: Carbohydrates are widely used as sweeteners, thickeners, and stabilizers in food and beverage products. They are also used as energy sources in sports drinks and energy bars. Pharmaceuticals: Carbohydrates are used as excipients in pharmaceutical formulations to improve the stability, solubility, and bioavailability of drugs. They are also used as a sourceof energy in medical nutrition products. Cosmetics: Carbohydrates are used in cosmetic products, such as moisturizers, shampoos, and conditioners, to provide hydration and improve skin and hair health. Biotechnology: Carbohydrates are widely used in the production of biodegradable plastics, biofuels, and other renewable energy sources. Research: Carbohydrates are widely used as research tools in the fields of immunology, virology, and cellular biology. They are used as ligands in protein-carbohydrate interactions and as probes to study cellular signaling pathways. 1.1.1 Cellulose-Based Water Filters Cellulose-based water filters are filters made from cellulose, a carbohydrate polymer found in plant cell walls. They are used to remove impurities and contaminants from water and are an alternative to traditional synthetic polymer filters. The high mechanical strength and hydrophilic properties of cellulose make it an ideal material for water filtration. Cellulose filters can effectively remove particles, pathogens, and other contaminants from water, making it safer and more potable. Cellulose-based water filters are widely used in both developed and developing countries for household, industrial, and agricultural applications. They are also an environmentally friendly alternative to traditional filters, as they are biodegradable and can be produced from renewable resources. Properties of cellulose based water filter Cellulose-based water filters have several properties that make them an attractive choice for water filtration: High Porosity: Cellulose-based water filters have a high porosity structure, which allows them to efficiently remove impurities and contaminants from water. Biodegradability: Cellulose-based water filters are made from a biodegradable material, cellulose, which reduces their impact on the environment compared to synthetic polymer filters. Cost-effective: Cellulose-based water filters are often more affordable than traditional synthetic polymer filters, making them accessible to a wider range of consumers and communities. Renewable resource: Cellulose-based water filters are made from a renewable resource, cellulose, reducing the dependency on non-renewable resources. Good mechanical strength: Cellulose-based water filters have good mechanical strength, allowing them to maintain their structure and perform effectively over time. Chemical resistance: Cellulose-based water filters are resistant to most chemicals, including acids and bases, and can be used in a wide range of water treatment applications. Large surface area: Cellulose-based water filters have a large surface area, which enhances their filtration capabilities and reduces the frequency of filter replacement. Importance of cellulose based water filters Cellulose-based water filters are important for several reasons: Safe and clean water: Cellulose-based water filters effectively remove impurities and contaminants from water, making it safer and more potable for various applications, including household, industrial, and agricultural use. Sustainability: Cellulose-based water filters are made from a renewable resource, cellulose, and are biodegradable, reducing their impact on the environment and promoting sustainability in water treatment processes. Affordability: Cellulose-based water filters are often more affordable than traditional synthetic polymer filters, making them accessible to a wider range of consumers and communities, especially in developing countries. Versatility: Cellulose-based water filters can be used in various types of filtration systems and can be produced in different sizes and shapes to fit specific needs. Alternative to synthetic filters: Cellulose-based water filters provide an environmentally friendly alternative to traditional synthetic polymer filters, reducing the dependency on non- renewable resources and reducing waste. Advantages of cellulose based water filters Cellulose-based water filters have several advantages that make them an attractive option for water filtration: Environmentally friendly: Cellulose-based water filters are made from a renewable resource, cellulose, and are biodegradable, reducing their impact on the environment compared to synthetic polymer filters. Cost-effective: Cellulose-based water filters are often more affordable than traditional synthetic polymer filters, making them accessible to a wider range of consumers and communities. High porosity: Cellulose-based water filters have a high porosity structure, which allows them to efficiently remove impurities and contaminants from water. Versatile: Cellulose-based water filters can be used in various types of filtration systems and can be produced in different sizes and shapes to fit specific needs. Good mechanical strength: Cellulose-based water filters have good mechanical strength, allowing them to maintain their structure and perform effectively over time. Chemical resistance: Cellulose-based water filters are resistant to most chemicals, including acids and bases, and can be used in a wide range of water treatment applications. Large surface area: Cellulose-based water filters have a large surface area, which enhances their filtration capabilities and reduces the frequency of filter replacement. Limitations of cellulose based water filters Cellulose-based water filters have some limitations that need to be considered when choosing a water filtration solution: Low resistance to high temperature: Cellulose-based water filters have low resistance to high temperature and can lose their structural integrity when exposed to high temperatures. Low filtration efficiency for certain contaminants: Cellulose-based water filters may not be efficient in removing certain contaminants, such as heavy metals, from water. Limited lifespan: Cellulose-based water filters have a limited lifespan and may need to be replaced more frequently compared to synthetic polymer filters. Difficult to sterilize: Cellulose-based water filters may be difficult to sterilize effectively, increasing the risk of contamination. May clog easily: Cellulose-based water filters may clog easily when exposed to high levels of contaminants, reducing their filtration efficiency and requiring frequent replacement. May affect water taste: Cellulose-based water filters may affect the taste of water by absorbing or releasing certain chemicals or minerals, reducing the quality of the purified water. Construction of cellulose-based water filters Construction of cellulose-based water filters involves the following steps: 1. Cellulose Material Selection: The type of cellulose material used in the water filter will depend on the desired properties such as strength, porosity, and chemical resistance. Common cellulose materials include paper, cotton, and wood fibers. 2. Cellulose Preparation: The cellulose material is prepared by cutting it into small pieces, washing it to remove impurities, and drying it for use. 3. Cellulose Layer Formation: The cellulose material is formed into a layer by either stacking it or compacting it using heat and pressure. 4. Filter Medium Attachment: The cellulose layer is attached to a filter medium such as a mesh or a support structure to provide stability and increase the filter surface area. 5. Chemical Treatment: The cellulose layer may be chemically treated to modify its properties, such as increasing its hydrophilicity or adding antimicrobial agents. 6. Housing Assembly: The filter medium is assembled into a housing that provides a means to attach it to a water source and to collect the filtered water. 7. Filter Testing: The completed filter is tested to ensure that it meets the desired specifications, such as filtration efficiency and flow rate. Note: This is a general outline, the exact process may vary depending on the specific requirements of the water filter and the type of cellulose material used. Cellulose material used in house hold water filters The cellulose material commonly used in household water filters is cellulose acetate. Cellulose acetate is a synthetic form of cellulose that has properties such as good chemical resistance, high porosity, and high flow rate, making it suitable for use in household water filters. Additionally, cellulose acetate is also a low-cost material, making it accessible for use in household applications. Figure: Cellulose acetate material Other cellulose materials such as paper, cotton, and wood fibers may also be used, but cellulose acetate is the most commonly used due to its favorable properties for water filtration applications. 1.1.2 PHA as Bioplastic Polyhydroxyalkanoates (PHAs) are a class of biodegradable and biocompatible polyesters produced by microorganisms, such as bacteria and fungi. They are a type of bioplastic. They are made from renewable resources, such as sugar and cornstarch, and are considered to be an environmentally friendly alternative to traditional petroleum-based plastics. Figure: General representation and examples of PHAs Properties of PHA PHAs have several properties that make them ideal for use as bioplastics, including: Biodegradability: PHAs are biodegradable and can break down into water and carbon dioxide, reducing their impact on the environment. Biocompatibility: PHAs are biocompatible and can be used in medical devices, such as sutures and implants, without causing adverse reactions in the body. Mechanical properties: PHAs have similar mechanical properties to traditional petroleum- based plastics, making them suitable for various applications. Processing: PHAs can be processed using conventional plastic processing techniques, such as injection molding, blow molding, and extrusion. Engineering applications of PHA bioplastic Packaging: PHA is used in various forms of packaging such as food containers, beverage cups, and clamshell containers. Medical Devices: PHA is biocompatible and can be used in the manufacture of medical devices such as sutures, implants, and drug delivery systems. Textiles: PHA is used in the production of biodegradable textiles, as well as for the production of biodegradable composites for use in construction and furniture. Agricultural Mulch Films: PHA is used in the production of biodegradable mulch films for agriculture to reduce soil erosion and conserve moisture. Consumer Goods: PHA is used in the production of various consumer goods, such as toys, phone cases, and water bottles. Automotive Parts: PHA is used for the production of biodegradable automotive parts such as air ducts and headlamp covers. Electronic Devices: PHA is used for the production of biodegradable components in electronic devices such as smartphones and laptops. Aerospace: PHA is used for the production of biodegradable parts in aerospace applications, such as insulation and cable management. Sporting Goods: PHA is used for the production of biodegradable sporting goods such as golf tees and fishing lures. Construction: PHA is used for the production of biodegradable insulation and soundproofing materials. 1.1.3 PLA as Bioplastic Polylactic Acid (PLA) is a biodegradable and bio-based plastic made from corn starch, sugarcane, or other natural resources. Figure: Molecular formula of PLA It is commonly used as a sustainable alternative to traditional petroleum-based plastics in various applications such as packaging, disposable tableware, and 3D printing. However, it's important to note that while PLA is biodegradable in industrial composting facilities, it may not break down in the environment as quickly as advertised and may still have negative impacts on wildlife and ecosystems if not properly disposed of. Properties of PLA as bioplastic Biodegradable: PLA can be broken down by microorganisms in industrial composting facilities, reducing waste in landfills. Renewable: PLA is derived from renewable resources such as corn starch or sugarcane, reducing dependence on finite petroleum resources. Clear/Transparent: PLA has a clear and transparent appearance, making it suitable for packaging applications. Heat-resistant: PLA has a relatively low melting temperature and is not recommended for high heat applications, but it can maintain its shape and stability up to 60°C. Biocompatible: PLA is non-toxic and biocompatible, making it suitable for food packaging and medical devices. Stiffness and Strength: PLA has good stiffness and strength, but not as strong as traditional petroleum-based plastics. Printability: PLA is commonly used in 3D printing due to its good printability and ease of use. Engineering applications of PLA bioplastic Automotive parts: PLA is used for the production of biodegradable automotive parts such as air ducts and headlamp covers. Electronic Devices: PLA is used for the production of biodegradable components in electronic devices such as smartphones and laptops. Aerospace: PLA is used for the production of biodegradable parts in aerospace applications, such as insulation and cable management. Sporting Goods: PLA is used for the production of biodegradable sporting goods such as golf tees and fishing lures. Construction: PLA is used for the production of biodegradable insulation and soundproofing materials. Agricultural Equipment: PLA is used for the production of biodegradable parts in agricultural equipment such as seed trays and greenhouse film. Medical Equipment: PLA is used for the production of biodegradable components in medical equipment such as diagnostic equipment and hospital beds. 1.2 Nucleic Acids: Nucleic acids are biopolymers that play a crucial role in the storage and transfer of genetic information in all living organisms. There are two types of nucleic acids: Deoxyribonucleic acid (DNA): DNA is the genetic material that carries the instructions for the development, functioning, and reproduction of all living organisms. DNA is a double- stranded helix structure composed of nucleotides, which consist of a sugar (deoxyribose), a phosphate group, and a nitrogenous base (adenine, guanine, cytosine, or thymine). Ribonucleic acid (RNA): RNA is involved in the expression of the genetic information stored in DNA by carrying the message from the DNA to the ribosome, where it is used to build proteins. RNA is a single-stranded molecule composed of nucleotides, which consistof a sugar (ribose), a phosphate group, and a nitrogenous base (adenine, guanine, cytosine, or uracil). Figure: Schematic representation of DNA and RNA Both DNA and RNA play essential roles in the functioning of cells and organisms, and their structures and interactions with other molecules are the basis for many biological processes such as replication, transcription, and translation. 1.2.1 DNA Vaccine A DNA vaccine is a type of vaccine that uses a piece of viral or bacterial DNA to stimulate an immune response against the pathogen. The vaccine works by introducing the pathogen's DNA into the body, where it is taken up by cells and used to produce viral or bacterial proteins. These proteins are then displayed on the surface of the cells, which triggers an immune response and the production of antibodies against the pathogen. DNA vaccines are being actively researched and developed for a range of diseases, including cancer, rabies, influenza, and human immunodeficiency virus (HIV). While the technology is still in its early stages, it has the potential to revolutionize the field of vaccine development and provide new treatment options for a range of diseases. 1.2.2 DNA Vaccine for Rabies A DNA vaccine for rabies is a type of vaccine that uses a piece of rabies virus DNA to stimulate an immune response against the virus. The vaccine works by introducing the rabies virus DNA into the body, where it is taken up by cells and used to produce viral proteins. These viral proteins are then displayed on the surface of the cells, which triggers an immune response and the production of antibodies against the rabies virus. Importance of DNA vaccine for rabies DNA vaccines offer several advantages over traditional vaccines, including their abilityto stimulate a strong and long-lasting immune response with fewer doses required, as well as their ease of manufacture and storage. These advantages make DNA vaccines particularly useful for preventing the spread of infectious diseases like rabies. In the case of rabies, DNA vaccines have several key advantages: Efficacy: DNA vaccines have been shown to be highly effective in preventing rabies infection in both animal and human trials. In one study, a DNA vaccine was found to be as effective as a traditional vaccine in protecting dogs against rabies. Long-lasting protection: DNA vaccines can stimulate a strong and long-lasting immune response, which means that they can provide protection against rabies for extended periods of time. Ease of administration: DNA vaccines are easy to administer, as they can be given via injection or even delivered orally, which can be particularly useful in areas where access to medical facilities is limited. Cost-effective: DNA vaccines are relatively inexpensive to produce compared to traditional vaccines, which can make them more accessible in areas where resources are limited. Reduced risk of side effects: DNA vaccines do not contain live virus particles, which means that they are generally safer and have a lower risk of side effects compared to traditional vaccines. 1.2.3 RNA Vaccines RNA vaccines are a type of vaccine that use genetic material from a pathogen, in the form of RNA, to stimulate an immune response against the disease. The vaccine works by introducing the pathogen's RNA into the body, where it is taken up by cells and used to produce viral or bacterial proteins. These proteins are then displayed on the surface of the cells, which triggers an immune response and the production of antibodies against the pathogen. RNA vaccines have several advantages over traditional vaccines, including faster production time and the ability to target multiple antigens. RNA vaccines can be manufactured quickly, making them a good option for emergency situations where large numbers of people need to be vaccinated quickly. RNA vaccines are also thought to be safer than traditional vaccines, as they do not contain any live virus or bacteria that could cause disease. RNA vaccines are currently being developed and tested for a range of diseases, including COVID 19, influenza, and cancer. 1.2.4 RNA Vaccines for Covid19 RNA vaccines for COVID 19 are a type of vaccine that use genetic material from the SARS- CoV-2 virus, in the form of RNA, to stimulate an immune response against the virus. The vaccine works by introducing the virus's RNA into the body, where it is taken up by cells and used to produce viral proteins. These proteins are then displayed on the surface of the cells, which triggers an immune response and the production of antibodies against the virus. The first RNA vaccine for COVID 19, the Pfizer-BioNTech vaccine, was authorized for emergency use in December 2020 and has been administered to millions of people worldwide. Another RNA vaccine, the Moderna vaccine, was also authorized for emergency use in December 2020. Importance of RNA vaccine for Covid 19 RNA vaccines have emerged as a promising tool for preventing the spread of COVID-19, offering several key advantages over traditional vaccine approaches. Here are some of the main reasons why RNA vaccines are important in the fight against COVID-19: High efficacy: RNA vaccines have been shown to be highly effective at preventing COVID- 19 infections. The Pfizer-BioNTech and Moderna mRNA vaccines, for example, have reported efficacy rates of around 95% in clinical trials. Rapid development: RNA vaccines can be rapidly developed and manufactured, making them particularly useful in the context of a pandemic. The Pfizer-BioNTech vaccine, for instance, was developed in under a year, and went from the initial discovery of the viral genome to emergency use authorization in less than 11 months. Easy to modify: RNA vaccines can be easily modified to target new strains or variants of the virus. This means that if a new variant emerges that is resistant to the existing vaccines, it is possible to quickly modify the vaccine to provide protection against the new strain. Safe: RNA vaccines are generally considered safe, as they do not contain any live virus particles. They work by instructing cells to produce a harmless piece of the virus (in this case, the spike protein), which triggers an immune response that provides protection against the virus. Potential for broader use: RNA vaccines have the potential to be used in the prevention of other infectious diseases, such as influenza, HIV, and Zika, as well as in the treatment of cancer. 1.2.5 Forensics – DNA Fingerprinting DNA fingerprinting, also known as DNA profiling or genetic fingerprinting, is a technique used in forensic science to identify an individual based on their unique DNA profile.The process involves analyzing specific regions of an individual's DNA, called markers, whichcan vary from person to person. Working of DNA fingerprinting for forensic applications Here is how it works: Sample collection: DNA is extracted from a biological sample, such as blood, semen, or hair. The sample is then purified and processed to isolate the DNA. DNA amplification: The extracted DNA is then amplified using a technique called polymerase chain reaction (PCR). PCR produces many copies of a specific DNA region, which allows for more accurate analysis. DNA analysis: The amplified DNA is then analyzed using a technique called gel electrophoresis. The DNA fragments are separated based on size and charge, and a DNA profile is generated. DNA comparison: The DNA profile obtained from the biological sample is then compared to the DNA profiles of other individuals, such as suspects or victims, to determine if there is a match. DNA comparison is typically done manually by forensic analysts, as it involves analyzing complex DNA profiles and comparing them to control samples to determine if there isa match. However, artificial intelligence (AI) is beginning to play a more prominent role in DNA analysis, particularly in the development of automated DNA profiling systems. The DNA profile consists of a series of bands on a gel, which represent specific DNA fragments. The bands are compared to those from a control sample, such as blood or saliva from a suspect or victim, to see if there is a match. If there is a match, it is considered strong evidence that the biological sample came from that individual. Forensic DNA fingerprinting has become a critical tool in criminal investigations, allowing investigators to link individuals to crime scenes and to exonerate innocent individuals who may have been wrongly accused. It has also been used to identify victims of natural disasters and mass casualties, and to resolve paternity disputes. 1.3 Proteins: Proteins are large, complex molecules made up of chains of smaller building blocks called amino acids. They play a vital role in the structure, function, and regulation of cells, tissues, and organs. Functions of Proteins Proteins perform a wide range of functions in the body, including 1) Catalyzing chemical reactions Amylase: An enzyme that breaks down starch into simple sugars like glucose and maltose. It is found in saliva and pancreatic juice. Lipase: An enzyme that breaks down fats into fatty acids and glycerol. It is found in the pancreas and small intestine. Catalase: An enzyme that converts hydrogen peroxide into water and oxygen. It is found in most cells of the body. Trypsin: An enzyme that breaks down proteins into smaller peptides. It is produced in the pancreas and released into the small intestine. ATP synthase: An enzyme that catalyzes the synthesis of ATP (adenosine triphosphate) from ADP (adenosine diphosphate) and phosphate. It is found in the mitochondria of cells. 2) Transporting molecules Hemoglobin: Hemoglobin is a protein found in red blood cells that transports oxygen from the lungs to the tissues in the body. Albumin: Albumin is a protein found in blood plasma that helps transport various substances such as hormones, fatty acids, and drugs throughout the body. Transferrin: Transferrin is a protein that transports iron in the blood from the site of absorption in the gut to the bone marrow, liver, and other tissues that require it. Apolipoproteins: Apolipoproteins are a family of proteins that transport lipids (fats) in the bloodstream. Examples include ApoA, ApoB, and ApoE. Ferritin: Ferritin is a protein that stores iron in a non-toxic form in the liver, spleen, and bone marrow. Glut transporters: Glut transporters are a family of proteins that transport glucose and other sugars across cell membranes. Examples include GLUT1 and GLUT4. 3) Providing mechanical support Collagen: Collagen is the main structural protein in the body and provides support to tissues such as skin, tendons, cartilage, bone, and teeth. Elastin: Elastin is a protein that provides elasticity and stretchability to tissues such as skin, lungs, arteries, and ligaments. Keratin: Keratin is a protein that forms the structural basis of hair, nails, and the outer layer of skin. Actin and Myosin: Actin and myosin are proteins that are involved in muscle contraction and provide the mechanical force required for movement. Tubulin: Tubulin is a protein that forms the structural basis of microtubules, which provide support to cells and are involved in various cellular processes such as cell division and intracellular transport. Laminin: Laminin is a protein that forms part of the extracellular matrix and provides structural support to cells in tissues such as skin, muscles, and organs. and 4) Regulating cell behavior Receptor proteins: Receptor proteins are proteins that are located on the surface of cells and bind to specific signaling molecules such as hormones, growth factors, and neurotransmitters. When these molecules bind to the receptor, they trigger a cellular response, such as a change in gene expression or the activation of an intracellular signaling pathway. Enzymes: Enzymes are proteins that catalyze specific chemical reactions in the body. Many enzymes are involved in regulating cellular behavior, such as kinases and phosphatases that regulate protein phosphorylation and dephosphorylation, respectively. Cytoskeleton proteins: Cytoskeleton proteins, such as actin and tubulin, play a critical role in regulating cell shape, movement, and division. Transcription factors: Transcription factors are proteins that bind to DNA and regulate gene expression. They play a critical role in regulating cellular differentiation, proliferation, and apoptosis. Adhesion proteins: Adhesion proteins are proteins that are involved in cell-to-cell and cell- to- matrix adhesion. They play a critical role in regulating cell behavior such as cell migration, tissue development, and wound healing. Ion channels: Ion channels are proteins that allow ions to move across the cell membrane. They play a critical role in regulating cellular excitability and communication. Proteins are also involved in immune responses, hormone regulation, and muscle contraction. The structure of a protein determines its function, and the sequence of amino acids in a protein determines its structure. There are 20 different types of amino acids, and the specific sequence of amino acids in a protein determines its unique structure and function. Proteins are synthesized by cells from the genetic information encoded in DNA. The process of protein synthesis begins when the genetic code for a particular protein is transcribedinto a molecule of messenger RNA (mRNA). The mRNA is then transported out of the nucleus and into the cytoplasm, where it is translated into a protein by ribosomes. Proteins play a critical role in many biological processes, and their dysfunction is involved in the development of many diseases, including cancer, heart disease, and neurological disorders. Understanding the structure and function of proteins is therefore a major focus of biomedical research, with the goal of developing new treatments and therapies for these diseases. 1.3.1 Proteins as Food Proteins are essential nutrients that provide the body with amino acids, which are the building blocks of the body's tissues. Proteins are found in many different foods, including meat, poultry, fish, dairy products, beans, lentils, tofu, and eggs. Proteins are a vital component of a healthy diet, as they help to build and repair tissues, support immune function, and regulate various metabolic processes. The body also uses proteins as a source of energy when carbohydrates and fats are not available. The quality of proteins in food is determined by the types of amino acids they contain, as well as the amount of each type of amino acid. To ensure adequate protein intake, it is important to consume a variety of protein-rich foods and to include both complete and incomplete protein sources in the diet. It is also important to consume sufficient amounts of other nutrients, such as carbohydrates, fats, and vitamins and minerals, to support overall health and well-being. Whey protein as food Whey protein is a type of protein derived from the liquid that separates from milk during the cheese-making process. It is a complete protein, meaning it contains all of the essential amino acids the body needs to build and repair tissues. Whey protein is widely used as a dietary supplement, particularly by athletes, bodybuilders, and people looking to increase their protein intake. It is commonly added to smoothies, shakes, and other beverages, and is also available in powder form that can be mixed into other foods or beverages. Compared to other types of protein, whey protein is rapidly absorbed by the body and is high in branched-chain amino acids, which are essential for muscle growth and repair. It is also a good source of essential nutrients, including calcium, potassium, and vitamins B2 and B12. However, it is important to note that not all whey protein products are equal in terms of quality, purity, and nutrient content. Some whey protein supplements may contain added sugars, artificial sweeteners, or other ingredients that can be harmful to health. It is therefore importantto choose a reputable brand and to carefully read the ingredient list before purchasing. Use of whey protein as food Whey protein is a type of protein derived from cow's milk and is commonly used as a food supplement. There are several uses of whey protein as food, including: Sports nutrition: Whey protein is often used by athletes and fitness enthusiasts to help build and repair muscle tissue, support recovery after intense exercise, and increase overall muscle mass. Weight management: Whey protein can be used to help manage weight by increasing satiety and reducing appetite. It can also help with weight loss by preserving muscle mass while reducing body fat. Health promotion: Whey protein is rich in essential amino acids and has been shown to have various health benefits, including improved immune function, lower blood pressure, and reduced risk of cardiovascular disease. Meal replacement: Whey protein can be used as a meal replacement, either as a drink or in a variety of food products. It provides a quick and convenient source of protein, making it a popular option for people with busy schedules or limited access to fresh foods. Whey protein is available in a variety of forms, including powders, bars, and drinks. It is often added to smoothies, baked goods, and other food products to increase the protein content. When using whey protein as food, it is important to choose a high-quality product that is free of artificial sweeteners, flavors, and other additives. It is also important to talk to a healthcare professional before starting to use whey protein, especially when one have any medical conditions or allergies. Meat analogs of protein Meat analogs, also known as meat substitutes or meat alternatives, are plant-based foods designed to mimic the taste, texture, and appearance of meat. They are made from a variety of ingredients, including soy protein, wheat protein, pea protein, and other plant-based ingredients, and are often fortified with vitamins and minerals to provide a similar nutritional profile to meat. Meat analogs are a popular alternative to meat for many people, including vegetarians, vegans, and those who are looking to reduce their meat consumption for health or ethical reasons. They can be a good source of protein and can help to meet the body's protein needs. There are many different types of meat analogs available, including burgers, sausages, meatballs, deli slices, and more. Some are designed to mimic specific types of meat, such as chicken, beef, or pork, while others are marketed as a more generic "meat-like" product. When choosing meat analogs, it is important to look for products that are high in protein and low in added sugars, fats, and other ingredients that can be harmful to health. It is also important to consider the texture and taste, as some meat analogs can be more appealing than others. Examples of meat analogs of protein as food Tofu: Made from soybeans, tofu is a versatile meat analog that can be used in a variety of dishes, including stir-fries, salads, and smoothies. Tempeh: Another soy-based product, tempeh is made from fermented soybeans and has a nutty flavor and firm texture. It can be sliced and used in sandwiches or salads, or crumbled and used as a meat substitute in tacos or spaghetti sauces. Seitan: Also known as wheat meat or wheat protein, seitan is made from wheat gluten and has a chewy, meat-like texture. It can be used as a substitute for beef or pork in a variety of dishes. Veggie burgers: Made from a variety of plant-based ingredients, including soy protein, grains, and vegetables, veggie burgers are a popular meat analog that can be grilled or baked and served on a bun. Meatless meatballs: Made from plant-based ingredients such as soy protein, grains, and vegetables, meatless meatballs are a tasty and protein-rich alternative to traditional meatballs. Plant-based sausages: Made from soy protein, pea protein, or other plant-based ingredients, plant-based sausages are a convenient and protein-rich alternative to traditional sausages. a) b) c) d) e) f) Figure: Images of a) Tofu, b) Tempeh, c) Seitan, d) Veggie burgers, e) Meatless meatballs, f) Plant-based sausages These are just a few examples of meat analogs of protein as food. There are many other products available that can provide a similar taste, texture, and nutritional profile meat, making it easier for people to reduce or eliminate their meat consumption for health or ethical reasons. 1.3.2 Plant Based Proteins Plant-based proteins are proteins derived from plant sources, such as legumes, grains, nuts, and seeds. They are becoming increasingly popular as a alternative to animal-based proteins, especially for those following a vegetarian or vegan diet. Here are some benefits of plant-based proteins: Sustainable: Plant-based protein sources are more environmentally sustainable than animal- based sources, as they require fewer resources to produce and generate fewer greenhouse gas emissions. Nutrient-rich: Many plant-based protein sources are also rich in other essential nutrients, such as fiber, vitamins, and minerals. Versatile: Plant-based proteins can be used in a variety of ways, including as a protein supplement, in smoothies, or as an ingredient in various recipes. Hypoallergenic: Plant-based proteins are often better tolerated than animal-based proteins, making them a good option for people with food allergies or sensitivities. Cost-effective: Plant-based protein sources are often more affordable than animal-based sources, making them a more accessible option for many people. Examples of plant-based proteins include soy protein, pea protein, lentil protein, chickpea protein, and hemp protein. It is important to choose a high-quality product that is free of artificial additives and preservatives, and to consult a healthcare professional before starting to use any new protein supplement. Uses of plant based proteins Plant-based proteins are commonly used in a variety of ways, including: Dietary supplements: Plant-based proteins are often sold as powders, bars, and other supplements, making them a convenient way to add protein to a diet. Food products: Plant-based proteins are used as ingredients in a variety of food products, including plant-based meat analogs, protein bars, and smoothies. Health and wellness: Plant-based proteins are often marketed as a healthier alternative to animal-based proteins, due to their lower saturated fat and cholesterol content. Vegetarian and vegan diets: Plant-based proteins are a popular source of protein for people following a vegetarian or vegan diet, as they do not contain animal products. Fitness and sports nutrition: Plant-based proteins are also used by athletes and fitness enthusiasts to support muscle recovery and growth. It is important to choose a high-quality plant-based protein product, and to consult a healthcare professional before starting to use any new protein supplement. It is also important to remember that plant-based proteins may not contain all of the essential amino acids found in animal- based proteins, so it may be necessary to consume a variety of plant-based protein sources to ensure adequate protein intake. 1.4 Lipids: Lipids are a group of organic compounds that include fats, oils, waxes, and some hormones. Figure: Schematic representation of lipid molecule, bilayer formation, and miscelle formation. Figure: Molecular structure of phospholipid (cell membrane) and triglyceride (fat) Role of Lipids Energy storage: Lipids are a major source of stored energy in the body, and they can be broken down to release energy when it is needed. Insulation: Lipids help to insulate the body, helping to regulate temperature and protect against heat loss. Cell membrane structure: Lipids are a major component of cell membranes, helping to maintain their fluidity and stability. Hormone synthesis: Some lipids, such as cholesterol, are precursors to hormones, and are necessary for their production. Transport: Lipids are soluble in fat, but not in water. This makes them ideal for carrying fat- soluble vitamins and other lipophilic compounds through the bloodstream. There are several types of lipids, including saturated and unsaturated fats, phospholipids, and steroids. It is important to have a balanced diet that includes a moderate amount of healthy lipids, such as monounsaturated and polyunsaturated fats, while limiting the intake of saturated and trans fats. This can help to maintain overall health and reduce the risk of chronic diseases such as heart disease and stroke. Engineering Applications of Lipids Cosmetics: Lipids are commonly used in cosmetics, such as moisturizers, to improve skin hydration and texture. Food industry: Lipids are used as ingredients in food products, such as margarine and frying oils, to improve texture, flavor, and shelf life. Medical devices: Lipids are used in medical devices, such as lipid-based drug delivery systems, to improve the delivery and efficacy of drugs. Biofuels: Lipids, such as vegetable oils and animal fats, can be converted into biofuels, such as biodiesel and bioethanol, to provide a renewable source of energy. Surface modification: Lipids can be used to modify the surface properties of materials, such as metals and polymers, to improve their performance and biocompatibility. Surfactants: Lipids are used as surfactants, which are compounds that reduce surface tension and improve the mixing of oil and water-based substances. It is important to note that the properties and applications of lipids can vary depending on the specific type of lipid and the processing method used. Further research is needed to fully understand and harness the potential of lipids in engineering applications. 1.4.1 Lipids as Biodiesel Lipids can be converted into biodiesel, which is a renewable source of energy. Biodieselis typically produced by transesterifying vegetable oils or animal fats with an alcohol, such as methanol, to form methyl esters. The resulting biodiesel can be used as a drop-in replacement for traditional diesel fuel in internal combustion engines. Advantages Renewability: Lipids are a renewable resource, and they can be produced from a variety of sources, such as vegetable oils, animal fats, and microalgae. Reduced emissions: Biodiesel produces fewer emissions compared to traditional diesel fuel, reducing the impact on the environment and public health. Improved performance: Biodiesel can improve engine performance, increasing fuel efficiency and reducing engine wear and tear. Biodegradability: Biodiesel is biodegradable, which reduces the risk of environmental contamination in the event of a spill. However, there are also some limitations to the use of lipids as biodiesel, such as higher production costs compared to traditional diesel fuel and the need for more efficient and cost- effective processing methods. Nevertheless, the use of lipids as biodiesel has the potential to play an important role in the transition towards a more sustainable energy system. The Process of Obtaining Biodiesel from Lipids Raw material preparation: The lipids, such as vegetable oils or animal fats, are collected and purified to remove impurities. Transesterification: The purified lipids are mixed with an alcohol, such as methanol, and a catalyst, such as sodium hydroxide, to produce fatty acid methyl esters (FAME), which are the main components of biodiesel. This process is known as transesterification. Separation: The reaction mixture is then separated into two layers: the upper layer contains the FAME (biodiesel) and the lower layer contains the glycerol (byproduct). Washing and drying: The biodiesel is washed with water to remove any residual alcohol and soap that was formed during the transesterification reaction. The biodiesel is then dried to remove any remaining moisture. Purification: The biodiesel is further purified to remove any impurities and improve its quality. Final product: The purified biodiesel is then stored and distributed for use as a fuel. It is important to note that the exact process can vary depending on the specific type of lipid and the desired quality of the final product. Further research is needed to improve the efficiency and cost-effectiveness of the biodiesel production process. 1.4.2 Lipids as Cleaning Agents/Detergents Personal care products: Lipids, such as fatty acids and glycerides, are commonly used as emulsifiers and surfactants in personal care products, such as shampoos, soaps, and lotions. Industrial cleaning: Lipids can be used as cleaning agents in various industrial applications, such as metal cleaning, degreasing, and stain removal. Laundry detergents: Lipids, such as fatty acids and glycerides, are used as ingredients in laundry detergents to improve their cleaning and sudsing performance. Cleaning agents for hard surfaces: Lipids can be used as cleaning agents for hard surfaces, such as floors, countertops, and walls, to remove dirt and grime. Lipids have several properties that make them suitable as cleaning agents, including their ability to emulsify and dissolve grease and oils. Additionally, lipids can form micelles, which are tiny spherical structures that can surround and trap dirt particles, making it easier to remove them. However, it is important to note that not all lipids are equally effective as cleaning agents and that the specific properties of each lipid can impact its performance. Further research is needed to optimize the use of lipids as cleaning agents and to identify new and more effective lipids for this purpose. Examples of lipids used as cleaning agent Soap: Soap is a traditional cleaning agent made from the reaction of an alkali with a fat or oil. Soaps are made from various types of lipids, including animal fats and vegetable oils. Fatty acids: Fatty acids, such as stearic acid, can be used as cleaning agents in personal care products, such as bar soaps and shampoos. Glycerol: Glycerol is a byproduct of soap production and can be used as a cleaning agent in a variety of applications, including household cleaners and industrial cleaning solutions. Fatty alcohols: Fatty alcohols, such as lauryl alcohol, can be used as cleaning agents in personal care products and industrial cleaning solutions. These are a few examples of lipids that are used as cleaning agents. There are many other lipids with different properties that can be used for cleaning, depending on the specific requirements of each application. Advantages of lipids as cleaning agents/detergents Biodegradability: Lipids are derived from natural sources, such as plants and animals, and are biodegradable, which makes them more environmentally friendly than many synthetic cleaning agents. Renewable resources: Lipids can be obtained from renewable resources, such as crops, and are not based on finite fossil fuels like some synthetic cleaning agents. Effectiveness: Lipids have excellent grease-cutting and emulsifying properties, making them effective cleaning agents. Mildness: Lipids are typically mild and gentle, making them suitable for use in personal care products, such as soaps and shampoos, and for cleaning delicate materials, such as silk and wool. Cost-effective: Lipids can be less expensive than synthetic cleaning agents, especially when obtained from low-cost feedstocks, such as vegetable oils. Customizability: Lipids can be modified and customized to improve their cleaning performance and to meet specific application needs. However, it is important to note that not all lipids are equally effective as cleaning agents and that the specific properties of each lipid can impact its performance. Further research is needed to optimize the use of lipids as cleaning agents and to identify new and more effective lipids for this purpose. Limitations of lipids as cleaning agents/detergents Limitations of using lipids as cleaning agents or detergents include: Stability: Some lipids can be susceptible to oxidation and degradation, which can reduce their effectiveness as cleaning agents over time. Compatibility: Some lipids may not be compatible with certain surfaces or materials, and may cause staining or damage. Cost: Although lipids can be less expensive than synthetic cleaning agents, the cost can vary depending on the source of the lipids and the processing methods used. Availability: The availability of lipids for use as cleaning agents may be limited by the availability of feedstocks, such as crops and animal fats, and the need for processing and refining. Performance: The cleaning performance of lipids can vary depending on the specific properties of each lipid and the type of soil or stain being removed. Some lipids may not perform as well as synthetic cleaning agents in certain applications. Regulation: The use of lipids as cleaning agents is regulated by government agencies, and specific requirements may vary from country to country. Overall, further research and development are needed to overcome these limitations andto optimize the use of lipids as cleaning agents and detergents. Working principle of lipids as cleaning agent The working principle of lipids as cleaning agents or detergents is based on their abilityto dissolve grease and oils. Lipids are composed of hydrophobic (water-fearing) and hydrophilic (water-loving) regions, which allows them to surround grease and oils, effectively breaking them down into smaller particles that can be more easily removed. This is why lipids are commonly used in cleaning products such as soaps, shampoos, laundry detergents, and dishwashing liquids. When a lipid-based cleaning agent is applied to a surface, the hydrophobic regions of the lipid molecule surround and dissolve grease and oils, while the hydrophilic regions interact with water, allowing the mixture to be rinsed away. The combination of the lipid and water also forms an emulsion, which helps to suspend and remove dirt and debris. In addition, some lipids have additional properties, such as foaming or lathering capabilities, that can enhance their cleaning performance. For example, fatty alcohols can be used as foaming agents in shampoos, while soap is known for its lathering properties. These additional properties can help to loosen and remove dirt and debris, making the cleaning process more effective. 1.5 Enzymes: Enzymes are proteins that act as catalysts in biological reactions. They speed up the rate of chemical reactions without being consumed in the process. Enzymes are specific to the type of reaction they catalyze, and they bind to specific substrates to facilitate the reaction. Enzymes play a crucial role in various metabolic pathways, digestion, and cellular respiration. Figure: Schematic representation of working of enzyme as catalyst Properties of Enzymes for Engineering Applications Enzymes have several properties that make them ideal for engineering applications, including: Specificity: Enzymes have a high level of specificity for the substrates they bind and the reactions they catalyze, making them highly efficient at performing specific tasks. Reactivity: Enzymes increase the rate of chemical reactions without being consumed in the process, allowing them to perform multiple cycles of the same reaction. Stability: Enzymes are generally stable at a wide range of temperatures and pH values, making them useful in a variety of industrial processes. Renewability: Enzymes are biodegradable and can be produced from renewable resources, making them an environmentally friendly alternative to traditional chemical catalysts. Cost-effectiveness: Enzymes can be produced in large quantities through fermentation, making them a cost-effective alternative to synthetic catalysts in many applications. These properties make enzymes ideal for use in various industrial and engineering applications, from bioremediation and biofuel production to food and beverage processing and textile production. Engineering Applications of Enzymes Bioremediation: Enzymes are used to break down pollutants in the environment, such as oils, pesticides, and toxic waste. Biofuel production: Enzymes are used to convert plant material into biofuels, such as ethanol and biodiesel. Food and beverage production: Enzymes are widely used in the food and beverage industry for processes such as baking, brewing, cheese making, and juice production. Textile production: Enzymes are used to remove stains, whiten fabrics, and improve the softness of textiles. Detergents: Enzymes are used in laundry detergents to break down protein, starch, and lipid stains. Pharmaceuticals: Enzymes are used in the production of various pharmaceutical products, such as antibiotics and vaccines. Research and biotechnology: Enzymes are used as tools in genetic engineering, protein engineering, and molecular biology. 1.5.1 Glucose-Oxidase in Biosensors Biosensors Figure: Schematic representation of the working of different biosensors Biosensors are analytical devices that combine a biological recognition element with a transducer to detect and quantify target analytes. The biological recognition element can be an enzyme, antibody, nucleic acid, or other biological molecule that specifically interacts with the target analyte. The transducer converts the biological response into an electrical signal that can be quantified and interpreted. Biosensors have a wide range of applications in the fields of medicine, environmental monitoring, and food safety. For example, biosensors can be used to monitor blood glucose levels in patients with diabetes, detect contaminants in water and food, and monitor environmental pollutants. Biosensors have several advantages over traditional analytical methods, including rapid response time, high sensitivity, specificity, and portability. Additionally, they can be designed to be disposable and cost-effective, making them a useful tool in various industries and applications. Enzymes Used in Biosensors Enzymes are commonly used in biosensors as the biological recognition element. Here are some examples of enzymes used in biosensors: Glucose oxidase (GOx): Used in blood glucose monitoring for people with diabetes. The enzyme oxidizes glucose to gluconic acid and hydrogen peroxide, which is then detected by a transducer to quantify glucose levels in the blood. Lactate oxidase (LOx): Used in the determination of lactate levels in biological fluids, such as blood and urine. LOx oxidizes lactate to pyruvate, which is then detected by a transducer. Cholinesterase (ChE): Used in the detection of organophosphorus pesticides and nerve agents. ChE hydrolyzes acetylcholine, and the decrease in acetylcholine levels is detected by a transducer to quantify the presence of the toxic substances. Alkaline phosphatase (ALP): Used in the detection of inorganic phosphates, such as those found in wastewater and fertilizers. ALP catalyzes the hydrolysis of phosphates to produce a signal that can be quantified. Urease: Used in the detection of urea levels in biological fluids, such as urine. Urease catalyzes the hydrolysis of urea to produce ammonium and carbon dioxide, which can be quantified by a transducer. These are just a few examples of the many enzymes that can be used in biosensors to detect and quantify a wide range of target analytes. Glucose-Oxidase in Biosensors Glucose oxidase (GOx) is an enzyme commonly used in biosensors for the detection of glucose levels in biological fluids, such as blood and urine. The enzyme catalyzes the oxidation of glucose to gluconolactone and hydrogen peroxide (H2O2), which can be easily detected and quantified by a transducer. Figure: Schematic representation of GOx In glucose biosensors, GOx is typically immobilized on a substrate, such as a polymeric film, to ensure stability and specificity. The transducer in the biosensor can be an electrode, a fluorescence-based system, or other type of sensor, depending on the desired level of sensitivity and specificity. Advantages of Biosensors Sensitivity: Biosensors are highly sensitive and can detect target analytes at low concentrations, making them useful in applications that require precise quantification. Specificity: Biosensors can be designed to specifically recognize a target analyte, which minimizes interference from other substances in the sample. Rapid response time: Biosensors can provide results in real-time, making them useful in situations where quick results are required. Portability: Biosensors can be designed to be small and portable, making them useful in field applications and remote locations. Cost-effectiveness: Biosensors can be manufactured at a low cost, making them an attractive alternative to more expensive analytical methods in some applications. Limitations of Biosensors Stability: Biosensors can be affected by environmental conditions, such as temperature and pH, which can lead to degradation of the biological recognition element and loss of sensitivity. Interferences: Biosensors can be affected by other substances in the sample, which can interfere with the performance of the biosensor. Calibration: Biosensors may require frequent calibration to ensure accuracy, which can increase the time and cost associated with using the biosensor. Limited shelf-life: Biosensors have a limited shelf-life, and the biological recognition element may degrade over time, leading to decreased sensitivity and specificity. Complexity: Biosensors can be complex to manufacture and use, requiring specialized equipment and expertise to operate effectively. Despite these limitations, biosensors have proven to be a valuable tool in various industries and applications, and research is ongoing to improve their performance and reduce limitations. 1.5.2 Lignolytic Enzyme in Bio-Bleaching Bio-Bleaching Bio-bleaching is a process that uses biological agents, such as enzymes, to remove color and brighten fibers, paper, and textiles. It is a sustainable alternative to traditional chemical bleaching methods that use harsh chemicals, such as hydrogen peroxide and chlorine. Advantages of Bio-Bleaching Sustainability: Bio-bleaching uses biological agents, such as enzymes, which are renewable and biodegradable, reducing the environmental impact compared to traditional chemical bleaching methods. Improved product quality: Bio-bleaching can result in higher brightness and a more uniform color compared to traditional chemical bleaching, leading to improved product quality. Reduced energy consumption: Bio-bleaching typically requires lower energy input compared to chemical bleaching methods, reducing energy consumption and associated costs. Elimination of hazardous chemicals: Bio-bleaching eliminates the use of harsh chemicals, such as hydrogen peroxide and chlorine, which can be hazardous to workers and the environment. Lower production of harmful by-products: Bio-bleaching reduces the formation of harmful by- products, such as dioxins, that can be produced during traditional chemical bleaching methods. Limitations of Bio-bleaching High cost of enzyme production: The cost of producing enzymes used in bio-bleaching can be high, making the process more expensive compared to traditional chemical bleaching methods. Low efficiency compared to chemical bleaching: Bio-bleaching can be less efficient compared to traditional chemical bleaching methods, requiring longer processing times and higher enzyme doses. Need for further research: Bio-bleaching is still in the early stages of development, and further research is needed to optimize the process and improve efficiency. Lack of widespread implementation: The widespread implementation of bio-bleaching is limited by factors such as the high cost of enzyme production, low efficiency compared to chemical bleaching, and the need for further research to optimize the process. Lignolytic Enzyme in Bio-Bleaching Lignolytic enzymes, such as laccases, peroxidases, and manganese peroxidases, are used in bio-bleaching to remove color and brighten fibers, paper, and textiles. These enzymes catalyze the oxidation of colored impurities in the fibers, resulting in a brighter and more uniform color. Laccases are copper-containing oxidases that catalyze the oxidation of lignin, a complex polymer found in plant cell walls, as well as other compounds such as phenols and aryl alcohols. Peroxidases are enzymes that use hydrogen peroxide to oxidize organic compounds. Manganese peroxidases are enzymes that use hydrogen peroxide to oxidize lignin and other compounds. The lignolytic enzymes used in bio-bleaching are typically produced by fungi or bacteria, and are immobilized on a support, such as a ceramic bead or a cellulosic matrix, to ensure stability and prolonged activity. The immobilized enzymes are then added to the fibers, where they catalyze the oxidation of colored impurities, resulting in a brighter and more uniform color.