Meteorology Lecture Notes PDF
Document Details
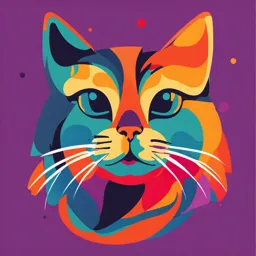
Uploaded by PermissibleSavanna9402
Tags
Summary
These lecture notes cover the fundamental concepts of meteorology, including the study of the atmosphere, weather patterns, and forecasting methods. The notes discuss different scales of meteorology, such as microscale and mesoscale, and explain weather phenomena like convection.
Full Transcript
Lesson 1 Scope of Meteorology Definition Meteorology is basically the study of the atmosphere. This field of science also investigates various atmospheric phenomena and the effects of the atmosphere on our weather. The gaseous layer surrounding our planet is called...
Lesson 1 Scope of Meteorology Definition Meteorology is basically the study of the atmosphere. This field of science also investigates various atmospheric phenomena and the effects of the atmosphere on our weather. The gaseous layer surrounding our planet is called the atmosphere. The earth’s atmosphere is approximately 100 to 125 km (65 to 75 mi) thick. The gravitational pull prevents further expansion of the atmosphere. Under the atmospheric science, there is a subdiscipline called Meteorology. This field encompasses all studies about the atmosphere. There are related sub disciplines under atmospheric sciences. One is called Climatology which is concentrated on how the world’s climates are altered by atmospheric changes. Another specialized subdiscipline is called Aeronomy which investigates the upper constituents of the atmosphere to analyze the unique physical and chemical processes that exist. On the other hand, Meteorology is fixated on the lower parts of the atmosphere, more specifically on the troposphere, where most weather activities happen. Meteorologists are specialists in weather forecasting. They use scientific principles to observe and explain the weather. Furthermore, through atmospheric research or operational weather forecasting, the potential weather conditions are predicted. Research meteorologists encompass multiple fields of study including atmospheric physics, climate modelling, air quality, remote sensing and climate change. The different interrelationship between the hydrosphere, atmosphere and the biosphere are also studied by these specialists. Forecasters use this research, alongside atmospheric records, to scientifically determine the atmosphere 's current condition and make forecasts of its future state. Atmospheric conditions both on and above Earth's surface are measured from a number of sources: weather stations, ships, buoys, aircraft, radar, weather balloons, and satellites. This data is being sent to centers across the world that provide computer analyses of global weather. These analyses are transmitted on to national and regional weather centers that feed these data into computers that model the atmosphere’s future condition. This information transfer shows how the study of the weather and the atmosphere take place in many, interconnected ways. Scales of Meteorology There are different scales of time and space at which weather happens. These are: microscale, mesoscale, synoptic scale, and global scale. Each meteorologist specifically focus on a particular scale. 1. Microscale Meteorology When we say microscale, the phenomena occur in a short range (from a few cm to few km). It also happens in a short life span (< a day). These weather conditions affect micro-geographic scales, temperatures and terrains on given areas. The processes that occur in soil, in surface waters, in near ground levels, even in vegetations are studied in microscale meteorology. The transfer of heat between the surfaces, even gas and liquid movement are measured. Hence, this scale often includes the study of chemistry. Air pollutants tracking is a good example of meteorology in a microscale. A practical microscale meteorology program between the USA and Mexico, called “MIRAGE - MEXICO” operates to study the physical and chemical changes of aerosols and gas pollutants within Mexico City. They use ground station observations, even aircrafts and satellites to monitor pollutants. 2. Mesoscale Meteorology In mesoscale meteorology, the range of observation is from a few km to approximately 1000 km. Two essential meteorological principles are involved: the mesoscale convective systems (MCS) and the mesoscale convective complex (MCC). Both aforementioned principles are affected by convection. Convection is a process that involves circulation. Convection occurs when the less-dense, warmer fluid rises, while the denser, colder fluid sinks. A fluid is any substance that flows. For meteorologists, the air is the most essential fluid they study. The movement and transfer of heat, moisture and energy is due to convection. These, in principle, are the fundamental elements that form the weather. In both the MCS and the MCC, a huge parcel of moisture and air is heated at midday (highest sun angle). As this heated air mass goes up into the cooler atmosphere, clouds form as it condenses, turning water vapor to precipitation. A single system of clouds can reach a huge size and generate heavy rainfall and even flooding. This is an MCC (mesoscale convective complex). In contrary, an MCS (mesoscale convective system) is a smaller cluster of thunderstorms lasting for a couple of hours. They are reactive to unique transfer of energy, moisture, and heat brought about by convection. The Deep Convective Clouds and Chemistry (DC3) is a program crafted to study thunderclouds and storms. This project determines how convection affects the making and movement of clouds, as well as the development of lightning. It is also useful in determining the impact of convection in flight patterns and aircraft. 3. Synoptic Scale Meteorology In synoptic-scale, the phenomena encompass several hundred to thousands of kilometers. As seen on local weather forecasts, high- and low- pressure systems have a synoptic scale. Pressure is another significant meteorological principle. It is at the core of large-scale weather systems. The study of pressure is diverse, in such, it explains the formation of hurricanes, cold outbreaks and other related systems. When the atmospheric (atm) pressure at the surface of the Earth is below the surrounding environment, then low pressure systems occur. From areas of higher pressure, wind and moisture search for low pressure systems. This drive, together with friction and the so-called “Coriolis effect”, will lead the system to rotate in a counter- clockwise direction in the Northern Hemisphere and also a clockwise direction in the Southern Hemisphere, hence, creating a cyclone. These cyclone phenomena have an inclination for upward vertical movement. This leads moist air from adjacent areas to rise, expand and condense to form water vapor, which soon forms clouds. The common weather events are due to this action of air and moisture. A good example of low-pressure systems (cyclones) are hurricanes that develop in tropical waters in the Western Hemisphere. Great amount of moisture from the sea is drawn by the system. This causes convection to be undertaken, that eventually causes wind speeds to intensify and pressure to decline. If these winds attain speeds of over 199 km/hr, the cyclone is considered as a hurricane. The most destructive natural calamities in the Western Hemisphere are hurricanes. There are hurricane centers that regularly provide weather reports and forecasts on all tropical weather systems. There are hurricane specialists in the eastern tropical Pacific and western tropical Atlantic that issue forecasts and warnings to any tropical storm during hurricane season. Forecasts from the National Hurricane Centers are necessary to mitigate potential threats. Many times during the day, the atmospheric (atm) pressure at the surface of the Ea rth is higher than its adjacent surrounding, this forms high-pressure systems. In this type of pressure, a downward vertical motion is predisposed, and dry air plus clear skies are observed. When high-pressure systems develop over the Arctic, then eventually move over the Northern Hemisphere, the extreme cold temperatures are experienced. Arctic air is immensely cold due to its development over ice and snow-covered soil. This cool air is very dense causing a push against the surface of the Earth with extreme pressure. This eventually prevents any heat or moisture from staying within the system. Many semi-permanent areas of high pressure have been identified by meteorologists. For example, there is an archipelago in the midi-Atlantic Ocean called Azores. Around this area, is a relatively stable region of high pressure, called the Azores high. Arid temperatures in the Mediterranean basin is brought about by the Azores high, as well as summer heat waves in Western Europe. 4. Global Scale Meteorology The transport of heat, wind, and moisture from the tropics to the poles are regarded as global scale phenomena. An essential pattern is global atmospheric circulation, the large-scale motion of air that helps dissipate thermal energy (heat) across the Earth’s surface. The fairly constant movement of winds across the globe is called global atmospheric circulation. When air masses move from areas of high pressure to areas of low pressure, winds are developed. Global atmospheric circulation is chiefly caused by Hadley cells. These Hadley cells are equatorial and tropical convection patterns. Convection moves warm air high in the atmosphere. On the other hand, cool air (dense) pushes lower in a constant loop. These loops are called Hadley cells. Meteorologists forecast the flow of trade winds is determined by Hadley cells. Businesses, particularly those product exporters study the strength of trade winds because these winds assist the faster travel of ships. In the midlatitudes, the winds that blow from the west are called westerlies. Nearer the Equator, trade winds move from the north of the Equator (northeast) and the south of the Equator (southeast). The long-term climate patterns that disturb global atmospheric circulation are studied by meteorologists. For example, meteorologists found out the pattern of El Niño. El Niño phenomenon encompasses trade winds and ocean currents across the Pacific Ocean. Roughly every five years, this phenomenon happens, creating disturbance in the global atmospheric circulation. It also affects local economies and weather from Peru to Australia. The Southern Oscillation, or the changes in air pressure in the Pacific Ocean is associated with El Nino. Over the eastern Pacific, near the coast of the Americas, air pressure drops. In contrast, over the western Pacific, near the coasts of Indonesia and Australia, air pressure rises. Weakened trade winds are experienced. Extreme rainfall is experienced in the Eastern Pacific nations. A corresponding reduction of fish stocks experienced due to warm ocean currents. This is so because the fish stocks depend on nutrient-rich upwelling of cold water to thrive. Eventually, Western Pacific nations experience tragic agricultural production due to drought. In order to help farmers, fishers and coastal residents, the meteorological processes of El Nino need to be understood to eventually prepare for the climate pattern. THINK! As a student, how can you use your knowledge of the difference meteorological scales? Make sure that your answers are practically sound. LEARNING ACTIVITY Download a Google Scholar Research Article related to any of the four meteorological scales; and Critique the paper taking into account the societal significance and the challenges to be addressed by future studies. Lesson 2 History of Meteorology The progress of meteorology is profoundly connected to developments in science, math, and technology. Around 340 BCE, the Greek philosopher Aristotle wrote the first major study of the atmosphere. Since Aristotle did not make any scientific observations, many of his ideas about the atmosphere are incoherent. When the use of the scientific method expanded, the study of meteorology greatly improved in the 17th and 18th centuries. An Italian physicist named Evangelista Torricelli found out that changes in air pressure were related to changes in the weather. In 1643, the barometer was invented by Torricelli. This will accurately measure the ai r pressure. The barometer is still a chief material in forecasting and understanding weather systems. In 1714, a German physicist named Daniel Fahrenheit, invented the mercury thermometer. These materials made it possible to concisely measure these two essential atmospheric variables. In the mid-1800s, the transfer of weather data became faster due to the invention of the telegraph by Samuel Morse, an American inventor. The first modern weather maps were first produced using this new telegraph technology by sharing information between and among meteorological offices. During this time more complex information such as isobars (lines of equal air pressure) and isotherms (lines of equal temperature) were combined and displayed on these maps. Using these large-scale weather maps, a broader geographic picture of weather was examined. Also, they were able to make more accurate forecasts. In the 1920s, the building blocks of modern weather forecasting were developed when a group of Norwegian meteorologists forged the notions of air masses and fronts. By understanding the fundamental laws of physics, meteorologists found out that huge warm and cold air masses move and meet in specific patterns that generate many weather systems. Other historic events that brought great advances to meteorology are the military operations during World War I and World War II. The operations’ success was highly reliant on weather over huge regions in the globe. To do this, the military operations invested much on research, trainings and new technologies to enhance their knowledge of the weather. Radar was one of the most important new technologies generated during these military operations. Radar was developed to detect the presence, direction, and speed of ships and aircrafts. When the wars ended, radar has still been used and enhanced to detect the presence, direction and speed of wind patterns and precipitation. The technological advancements in the 1950s and 1960s made it easier and faster for meteorologists to study and predict massive scale weather systems. More complex technological systems were developed in the 1950s when computers created the 1 st models of atmospheric conditions. This was done by generating complex equations after running hundreds of data points. The series of high- and low- pressure systems encircling the globe is observed and large-scale weather systems were predicted through these models. In 1962, the first accurate weather forecast from space was provided by the first meteorological satellite, TIROS I. More sophisticated satellites were created due to the success of TIROS I. Because of these satellites’ ability to collect and transfer data with very high accuracy and speed made crucial tools to meteorologists. Advanced satellites with their corresponding data-processing computers are the main tools used in meteorology today. Meteorology Today In the study of meteorology today, there are different tools that examine, describe, model and predict weather systems. The applications of these technologies are at different meteorological scales, enhancing forecast efficiency and accuracy. In terms of forecasting, radar is an essential remote sensing technology. A radar dish is an activated sensor that shoots out radio waves that bounce back particles from the atmosphere and return it to the dish. The pulses are processed by a computer and the horizontal dimension of clouds and precipitation, likewise, the speed and direction in which these clouds are moving would be determined. The dual-polarization radar is a new technology that transfers both vertical and horizontal wave pulses. This dual-polarization radar is better able to approximate precipitation due to the additional pulse. Additionally, it differentiates more accurately the various types of precipitation: hail, sleet, snow and rain. This new radar technology will also seriously improve winter-weather and flash-flood forecasts. To understand the global scale weather phenomena, satellites are absolutely necessary and are very important. The NASA or the National Aeronautics and Space Administration and the NOAA or the National Oceanic and Atmospheric Administration in the USA operate three GOES (Geostationary Operational Environmental Satellites. These satellites provide weather observations for more than 50% of the surface of the Earth. A recent improvement in 2010, GOES-15 includes a solar x-ray imager that observes and monitors the sun’s X-rays the early detection of solar flares and other solar phenomena. This is important because solar flares affect commercial and military satellite communications all over the globe. A highly accurate imager produces visible and infrared images of severe storm developments, cloud cover, oceans, and the Earth’s surface. Infrared imagery detects the motion and transmission of heat, enhancing our knowledge of the global energy balance and processes like severe weather, convection and even global warming. Figure 1. Hadley cells are our guide for our understanding of global-scale meteorology. (Illustration courtesy NASA) THINK! Discuss the major turning points that gave rise to the present-day study of Meteorology. LEARNING ACTIVITY In a tabular form, explain the main events in the various turning points that elucidates the history of Meteorology Lesson 3 The Earth’s Atmosphere (Inner Layers) The Earth’s atmosphere is composed of homogeneously mixed gases that surrounds the planet. The atmosphere gives us something to breathe. It also helps in the existence of life on Earth because (1) it protects us from most UV (ultraviolet) radiation that comes from the sun. (2) It also warms the surface of the Earth by approximately 33 oC or 59oF through the so-called greenhouse effect. Finally, it prohibits extreme changes in the temperature during daytime and nighttime. Gases in Earth's Atmosphere Nitrogen and oxygen are the most common gases in the Earth’s atmosphere. Our dry air constitutes about 78% nitrogen (N2) and around 21% oxygen (O2). Argon, carbon dioxide and several other gases are also found in the atmosphere in much lower amounts (each makes up lower than 1% of the atmosphere’s gas mixture). Water vapor is also an inclusive part of the atmosphere. It is present in varied amounts (1% on average). There are also tiny particles – both solid and liquid – that “floats” in the atmosphere. These particles are called “aerosols” and they include dust, pollen and spores, smoke, salt from sea spray, volcanic ash and others. Layers of Earth's Atmosphere As we move upward from the Earth’s surface, the atmosphere grows thinner. This means it becomes less dense and lower in pressure. It also slowly gives way to the vacuum in outer space. The atmosphere’s “topmost” part is not precisely known. Air becomes very thin at altitudes between 62 to 75 miles (100 to 120 km) going up, and this can be considered as the boundary between the atmosphere and space. But, there are still very thin measurable traces of atmospheric gases beyond this atmosphere – outer space boundary. The atmosphere has several distinct layers or regions. Each of these layers has its own unique temperatures, pressures and conditions. We live and we are closest in the troposphere. This is the lowest layer in the Earth’s atmosphere. Most clouds are formed here. Most weather events occur in this layer. Since most clouds are found here, aircrafts fly in the next higher layer, the stratosphere, which contains the jet streams and also the ozone layer. Above the stratosphere are the mesosphere, thermosphere and exosphere. Figure 1. The layered structure of the planet's atmosphere is observed in this sunset view from the International Space Station. (Credit: Image Science & Analysis Laboratory, NASA Johnson Space Center) The Troposphere The lowest layer of the Earth’s atmosphere is called the troposphere. Majority of the mass (around 75 to 80%) of the Earth’s atmosphere is found in the troposphere. Most kids of clouds are formed in this layer, and nearly all weather happens here. This layer is also considered the most wet layer in the atmosphere. With the exception of this layer, all other layers above contain very little moisture. The Earth’s surface is found at the bottom of the troposphere. This layer extends upward to around 10 kilometers (around 6.2 miles or approximately 33,000 feet) above sea level. The height of the top of the troposphere is inversely proportional to the latitude. This means that the top of the troposphere is lowest over the poles and highest at the equator. And by season, the troposphere is lower in winter and higher in summer. The troposphere can be as high as 20 kilometers (12 miles or around 65,000 feet) close to the equator. It can also be as low as 7 kilometers (4 miles or 23,000 feet) over the north/south poles during winter. Near the ground level, at the bottom of the troposphere, air is warmest. As one rises through the troposphere, air gets colder. This is the reason why the peaks of tall mountains can still be covered with snow even in summertime. Density and air pressure decrease with altitude. This is the reason why the cabins of high-flying aircrafts are pressurized. Furthermore, the layer just above the troposphere is the stratosphere. There is a boundary between the troposphere and the stratosphere, this boundary is called the tropopause. Figure 2. This diagram shows some of the features of the troposphere. (Credit: Randy Russell, UCAR) The Stratosphere The stratosphere is another distinct layer in the planet’s atmosphere. As you go upward, it is the second layer of the Earth’s atmosphere. The troposphere is just beneath this layer. Above the stratosphere, the next higher layer is called the mesosphere. The stratosphere’s bottom is around 10 kilometers (6.2 mi or approximately 33,000 ft) above the ground at middle latitudes. On the other hand, the stratosphere’s top portion happens at an altitude of 50 kilometers (31 mi). The height of the stratosphere’s bottom differs with latitude and with the seasons. The stratosphere’s lower boundary can be as high as 20 kilometers (12 mi or 65,000 feet) close to the equator and as low as 7 kilometers (4 mi or 23,000 ft.) at the poles during winter. Tropopause is the term used to call the lower boundary of the stratosphere. Consequently, stratosphere is the term used to call the upper boundary of the stratosphere. A special type of oxygen molecule is present, with relative abundance, in the stratosphere. It is called “ozone” The ozone layer absorbs energy from incoming UV (ultraviolet) rays from the sun. As one moves upward through the stratosphere, temperature rises up. This is the exact opposite behaviour in the troposphere where we live, wherein temperatures decrease with an increase in altitude. Due to temperature stratification in this layer, there is little mixing and convection in the stratosphere. This means there is quite stability in the layers of air. In its practical sense, commercial aircrafts fly in the lower portion of the stratosphere to stay away from the turbulence that is usual in the troposphere below. Furthermore, the air in the stratosphere is very dry. It contains little water vapor. As a consequence, few clouds are formed in the stratosphere.Almost all clouds exist in the more humid, lower troposphere. Exceptions to this are the polar stratospheric clouds (PSCs). These clouds are found in the lower stratosphere near the poles during winter. PSCs are found at altitudes of 15 to 25 kilometers (9.3 to 15.5 mi) and they form only when temperatures at these given heights drop below -78 deg C. These clouds potentially aggravate the formation of ozone layer “holes” by stimulating particular chemical reactions that disrupts the ozone layer. They are also called nacreous clouds. The air at the top of the stratosphere is approximately 1000x thinner than it is sea level. Due to this trait, weather balloons and aircrafts attain their maximum operational altitudes in this layer. Since vertical movement of air or convection is lacking in the stratosphere, particulate materials that enter into this layer can stay in this layer for long periods of time. This is the reason why CFCs or chlorofluorocarbons are called “ozone-destroying chemicals.” When these materials enter the stratosphere, they interact and degrade the ozone chemical bonding. In addition, events such as major volcanic eruptions and large meteor impacts can throw aerosol particles up in the stratosphere where they stay for months or years. These materials can even alter the Earth’s climate. There are also other gases emitted by rocket launches that stay and may become problematic into the stratosphere. The stratosphere is also influenced by various kinds of waves and tides in the atmosphere. Various waves and tides contain energy from the troposphere that goes up to the stratosphere. Others can even move further from the stratosphere to the mesosphere. These tides and waves affect the air flow in the stratosphere and can even cause regional heating of this atmospheric layer. A unique kind of electrical discharge, a little similar to lighting exists in the stratosphere. These are called “blue jets” and they are seen above thunderstorms. They extend from the lowest part of the stratosphere up to altitudes of 40 or 50 kilometers (25 to 31 mi). THINK! Illustrate what the world would be like without the ozone layer. Explain why it would look like such. Figure 3. This diagram shows some of the features of the stratosphere. (Credit: Randy Russell, UCAR) LEARNING ACTIVITY In a tabular form, compare and contrast the two inner layers of the atmosphere Lesson 4 The Earth’s Atmosphere (Outer Layers) The Mesosphere Another layer in the Earth’s atmosphere is the mesosphere. This layer is right above the stratosphere. It is also underneath the thermosphere. Above our plant, it extends from 50 to 85 kilometers (31 to 53 mi). Throughout the mesosphere layer, the temperature diminishes with height. In the Earth’s atmosphere, the coldest temperature is about -90 deg C (-130 deg F) and these are felt near the top of the mesosphere. The mesopause is the boundary between the mesosphere and the thermosphere above it. On the other hand, at the bottom of the mesosphere is called the stratopause. This is the boundary between the mesosphere and the stratosphere underneath it. Little information is known about the mesosphere because this layer is hard to study compared to other layers of the atmosphere. Aircrafts and other weather balloons could not travel high enough to arrive at the mesosphere. Even satellites that fly above this layer cannot straightly measure the characteristics of the mesosphere. To compensate for this, scientists are using sounding rocket instruments to sample this layer directly. However, these tests are short and infrequent. Due to this difficulty in obtaining measurements in this layer, through direct instruments, a lot is still unknown about the mesosphere. Most meteors dissolve in the mesosphere layer. When this happens, some materials are left floating in the mesosphere. This causes the layer to contain comparatively high concentration of metal atoms such as iron. There are interestingly strange clouds that form in the mesosphere close to the poles. These high altitude clouds are called “noctilucent clouds.” Sometimes, they are called “polar mesospheric clouds.” These unique clouds are developed far higher up compared to other types of clouds. They are unique because this layer, including the stratosphere below it is very dry in comparison to the moist troposphere where we are in, making the formation of clouds in this layer a genuine surprise. There are also bizarre electrical discharges similar to lightning that are generated in this layer. These are called “sprites” and “elves”. These discharges sometimes appear in this layer hundreds of kilometers above thunderclouds generated in the troposphere below. Middle atmosphere is often used to refer to the combined stratosphere and mesosphere. At the top of the mesosphere (the mesopause) and below, various atoms and molecules that make up gases are mixed together by irregular atmospheric motion in the atmosphere. However, as we go up the mesosphere into the thermosphere, and far beyond, collisions of gas particles become so infrequent. The gases even appear separated according to the kind of chemical elements they possess. Several types of atmospheric tides and waves affect the mesosphere. These tides and waves hold energy from the troposphere into the stratosphere and then upward to the mesosphere, driving the bulk of its global circulation. Figure 4. This diagram shows some of the features of the mesosphere. (Credit: Randy Russell, UCAR) The Thermosphere The thermosphere is another atmospheric layer of the Earth. It is straight above the mesosphere. It is also right below the exosphere. It reaches from approximately 90 kilometers (56 mi) to between 500 to 1000 kilometers (311 to 621 mi) above the Earth. Temperatures surge high in the lower thermosphere (below 200 to 300 kilometers altitude). This levels off and clutch fairly steady with increasing altitude above that given height. The temperature is greatly affected by solar radiation in the thermosphere. This layer is approximately around 200 deg C (360 deg F) hotter during daytime compared to night time. It is also approximately 500 deg C (900 deg F) hotter when the sun is highly active compared to other times. The temperatures in the upper thermosphere could probably range from around 500 deg C (932 deg F) to 2000 deg C (3632 deg F) and even higher. The thermosphere and the exosphere boundary is termed the “thermopause.” At the bottom of the thermosphere layer, is the boundary called mesopause which separates the thermosphere and mesosphere below it. The air density in the thermosphere is very low. Because of this, people usually think of this layer as the “outer space” though it is still considered to be part of the Earth’s atmosphere. As a matter of fact, outer space is often defined to have an altitude of 100 kilometers (62 mi), just little above the mesopause at the bottom part of the thermosphere. The International Space Station (ISS) and the space shuttle both orbit the planet within the thermosphere! Because of the turbulence in the atmosphere, various kinds of atoms and molecules are entirely mixed together below the thermosphere. To have a comparison, air in the lower atmosphere is chiefly composed of approximately 80% N2 (Nitrogen molecules) and around 20% O2 (Oxygen molecules. On the other hand, the gas particles rarely crash in the thermosphere and above. Rather, they are assumed to be separated based on the kind of element they possess. There are also highly active and energy-rich UV and X-ray photons coming from the sun. These active radiations crack molecules apart in the thermosphere. In the upper portion of the thermosphere, elements exist as atoms. We can see atopic oxygen (O), atomic nitrogen, (N) and also Helium (He) as the main components of its air. In the thermosphere, the majority of ultraviolet (UV) rays and even X-rays coming from the sun are absorbed. An activity called “puffing up” happens in the thermosphere when the sun becomes highly active and emits more high energy rays consequently making the thermosphere hotter then it expands. As a result, the thermopause’s (height on top of the thermosphere) height differs. The thermopause is located at an approximate altitude of 500 to 1000 kilometers, or higher. The thermosphere’s heating and expansion causes shifts in the density of extremely thin air found in this layer. As a consequence, it generates a drag force on satellites found at orbital altitudes. Since many satellites are found in the thermosphere, engineers should consider these drag force differences. Likewise, satellites should be boosted higher at times to offset the effects of the drag force. Electrically-charged ions from atoms and molecules are created in the thermosphere when high-energy radiation breaks electrons apart from gas particles. This region of the thermosphere is called the ionosphere. This region comprises various regions of these ionized particles that converge and share the same space with the generally uncharged region of the thermosphere. Similar to oceans, the planet’s atmosphere has tides and waves within it. These tides and waves transfer energy around and within the atmosphere, reaching up to the thermosphere. The overall circulation including winds in this layer are hugely driven by these waves and tides. In some parts of the thermosphere, moving ions interact with uncharged (neutral) gases when they are dragged by collisions. These reactions produce powerful electrical currents. The thermosphere is most famous because of the presence of Aurora borealis (the Southern and Northern Lights) that occur in this layer. These spectacular lights are generated when charged particles (protons, electrons, and other ions) come into collision with atoms and molecules in the thermosphere particularly at high altitudes. This reaction excites them into higher energy states. The excess energy produced by these atoms and molecules are released as photons of light, which we observe as colourful displays in the night sky (near the polar region). Figure 5. Aurora borealis (Southern / Northern Lights) © Markus Kili The Exosphere The uppermost region of the Earth’s atmosphere is called the exosphere. This region then gradually dwindles into the vacuum of space. Air in this region is extremely thin and it is almost similar to the void, airless outer space. The thermosphere is right below the exosphere. The divider between the two is termed the thermopause. The bottom of this layer (exosphere) is also usually called exobase. The altitude of the lower portion of the layer fluctuates. Around the peak of the sunspot cycle, when the sun is active, UV radiation and X-rays from the sun “puff up” the heated thermosphere. This causes the altitude of the thermopause to be raised to heights around 1000 kilometers (620 mi) above the planet’s surface. On the other hand, during the low point of the sunspot, when the sun is less active, the thermopause recedes to approximately 500 kilometers (310 miles) of the planet’s surface due to less intensity of the solar radiation. Not all meteorological scientists consider the exosphere as part of the atmosphere. Some of these scientists regard the thermosphere as the topmost layer of the planet’s atmosphere, and consider that exosphere as a constituent of space. Nonetheless, there are other scientists who contemplate the exosphere to be part of the Earth’s atmosphere. Due to the fact that the exosphere slowly disappears into outer space, there is no clear partition of this layer. The outermost limit of the exosphere can be estimated at around 190,000 kilometers (120,000 mi), and could be placed as the uppermost edge of the Earth’s atmosphere. This is also about halfway to the Moon. At this point, the sun’s radiation pressure generates more force on H (hydrogen) atoms compared to the pull of the planet’s gravity. At heights of 100,000 kilometers (62,000 mi), satellites detected a faint glow of UV radiation scattered by H (hydrogen atoms). This region of ultraviolet (UV) glow is termed the geocorona. There are constant collisions of molecules and atoms of atmospheric gases below the exosphere. But due to the extremely thin nature of air in the exosphere, these collisions are relatively rare. There is a unique movement of gas atoms and molecules in the exosphere. They move in a so-called “ballistic trajectory” similar to when a cannonball is shot (arching flight of a thrown ball that gradually curves back towards the Earth). This is due to the gravitational pull. Majority of gas particles in this layer travel along curved directions without ever bumping another atom or molecule. These particles finally arc back to the lower atmosphere due to gravitational pull. In contrast, some particles don’t return to Earth, instead, some of the highly moving gases fly off into space. A small fraction of the Earth’s atmosphere escapes into space in this way each year. Technically speaking, we can consider the exosphere as part of the planet’s atmosphere. However, in many ways, it can be regarded as a portion of outer space. The ISS (International Space Station) and many other satellites circuit within the exosphere or below. For instance, the average altitude of the ISS is approximately 330 kilometers (205 mi), positioning it below the exosphere. Despite air being extremely thin in the thermosphere and exosphere, there could still be sufficient air to trigger a minute volume of drag force on satellites that course within these layers. This drag force causes a gradual slowing down of spacecrafts in their orbits. If not taken cared of, they will finally fall out of orbit and they will burn up as they re -enter the atmosphere. To resolve this, spacecraft boosts are placed to push them back upwards. A two kilometer (1.2 mi) “orbital decay” in altitude is lost by the ISS every month. Hence, it must occasionally be given a rocket engine-upward boost to keep it in its orbit. LEARNING ACTIVITY Explain the existence of Northern Lights / Aurora borealis. Not all scientists agree that the exosphere is really a part of the Earth’s atmosphere Why? MODULE SUMMARY Meteorology was introduced as a study of the Earth’s atmosphere and the movements at the atmosphere on short-term ranges. We have learned about the weather, a study that is focused on current and near-future predictions of atmospheric data. Various weather elements define the atmosphere. This includes temperature, type and amount of precipitation, direction and strength of wind, atmospheric pressure, and even cloud cover. The atmospheric circulation systems can be differentiated in three major spatiotemporal scales: (1) microscale, (2) mesoscale, and (3) macroscale (that includes planetary and synoptic scale motions). Meteorology has improved into a highly physics- based discipline with many specialization fields that necessitates highly trained professionals. In this module the Earth’s atmosphere was also discussed. This area consists of a mixture of gases that covers the Earth. Aside from its most significant use, that is to help us breathe, the atmosphere is the reason why life on the planet is feasible. It protects us from the most dangerous UV radiation from the solar rays. It also keep the surface of the Earth warm by approximately 33 deg C (59 deg F) through the presence of the greenhouse gases. It also helps minimize extreme disparities between nighttime and daytime temperatures. Congratulations! You have just studied Module I. now you are ready to evaluate how much you have benefited from your reading by answering the summative test. God bless!!! SUMMATIVE TEST If a meteorologist already has a good working knowledge on a macroscale meteorological investigation, does he/she still needs to learn the microscale? Why or why not? If a meteor falls into the Earth’s atmosphere, how big should it be to reach the Earth’s surface layer and cause global destruction? Explain and cite references to support your answer. Explain the chemical reaction behind the destruction of the ozone layer. What materials and substances can significantly alter this layer. What happens to us when we are exposed to the strong UV radiations from the sun. Explain the physiology at a cellular level. The Earth as an Ecosystem The Primitive Earth The Earth is composed of four different layers. Many geologists believe that as the Earth cooled the heavier, denser materials sank to the center and the lighter materials rose to the top. Because of this, the crust is made of the lightest materials (rock- basalts and granites) and the core consists of heavy metals (nickel and iron). The crust is the layer that you live on, and it is the most widely studied and understood. The mantle is much hotter and has the ability to flow. The Outer and Inner Cores are hotter still with pressures so great that you would be squeezed into a ball smaller than a marble if you were able to go to the center of the Earth The Crust The Earth's Crust is like the skin of an apple. It is very thin in comparison to the other three layers. The crust is only about 3-5 miles (8 kilometers) thick under the oceans(oceanic crust) and about 25 miles (32 kilometers) thick under the continents (continental crust). The temperatures of the crust vary from air temperature on top to about 1600 degrees Fahrenheit (870 degrees Celcius) in the deepest parts of the crust. The mantle is the layer located directly under the sima. It is the largest layer of the Earth, 1800 miles thick. The mantle is composed of very hot, dense rock. This layer of rock even flows like asphalt under a heavy weight. This flow is due to great temperature differences from the bottom to the top of the mantle. The movement of the mantle is the reason that the plates of the Earth move! The temperature of the mantle varies from 1600 degrees Fahrenheit at the top to about 4000 degrees Fahrenheit near the bottom! The core of the Earth is like a ball of very hot metals. (4000 degrees F. to 9000 degrees F.) The outer core is so hot that the metals in it are all in the liquid state. The outer core is located about 1800 miles beneath the crust and is about 1400 miles thick. The outer core is composed of the melted metals nickel and iron.The inner core of the Earth has temperatures and pressures so great that the metals are squeezed together and are not able to move about like a liquid, but are forced to vibrate in place as a solid. The inner core begins about 4000 miles beneath the crust and is about 800 miles thick. The temperatures may reach 9000 degrees F. and the pressures are 45,000,000 pounds per square inch. Reference: http://volcano.oregonstate.edu/ 1. LITHOSPHERE – this is the rocky material of the earth’s outer covering and is the vital source all mineral elements essential by living organisms. 2. HYDROSPHERE – This is the water on or near the earth’s surface, and it ranges into the lithosphere and the atmosphere. 3. ATMOSPHERE- The gaseous element of the biosphere which extends to some 3500 km above the surface of the earth, but all life is kept to the lowest 8 to 15 km (troposphere). LEARNING ACTIVITY Activity 1. Label the parts of the Earth using the illustration below Activity 2 Describe each layers using the table below Layers of the Earth Description(s) Draw each layer of the biosphere then enumerate some animals that are found in it. Lithosphere Hydrosphere Atmosphere Congratulations!