lq2 treaphs.pdf
Document Details
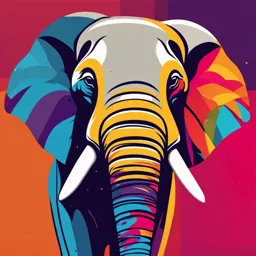
Uploaded by IngenuousGold6378
De La Salle University
Tags
Full Transcript
The Air Age On December 17, 1903, a bicycle repairer by the name of Orville Wright propelled himself through the air a distance of 120 ft. near Kitty Hawk, North Carolina. This was the first powered flight in a heavier-than-air aircraft known to humans. It was the equivalent of 0.23 passenger-...
The Air Age On December 17, 1903, a bicycle repairer by the name of Orville Wright propelled himself through the air a distance of 120 ft. near Kitty Hawk, North Carolina. This was the first powered flight in a heavier-than-air aircraft known to humans. It was the equivalent of 0.23 passenger-mile, the first such statistic that could be recorded in aviation history. In contrast to this humble beginning, the commercial airlines in the United States in 1990 carried more than 450 million passengers, almost 350 billion passenger-miles, which is nearly 10 times the number of passenger-miles logged by railroads and buses. The availability of air transport, however, has done more than provide a carrier service. It has affected our economic way of life, it has made changes in our social and cultural viewpoints, and it has had a hand in shaping the course of political history. The sociological changes brought about by air transportation are perhaps as important as those it has brought about in the economy. People have been brought closer together and so have reached a better understanding of interregional problems. Industry has found new ways to do business. The opportunity for more frequent exchanges of information has been facilitated, and air transport is enabling more people to enjoy the culture and traditions of distant lands. AIRPORT PLANNING Introduction The planning of an airport is a complex process that the analysis of one activity without regard to its effect on other activities will not provide acceptable solutions. In the past, airport master plans were developed on the basis of local aviation needs. In more recent times, these plans have been integrated into an airport system plan which assessed not only the needs at a specific airport site but also the overall needs of the system of airports which service an area, region, state, or country. Components of an Airport System for a Large Airport Enroute Airspace Airport System Terminal Airspace Airfield surface system Runway Air Side Holding Pad Exit Taxiway Taxiway system Apron-gate area Terminal Buildings Land Side Vehicular Circulation Parking Airport Ground Access System Aircraft flow Passenger flow TYPES OF AIRPORT PLANNING STUDIES The Airport System Plan An airport system plan is a representation of the aviation facilities required to meet the immediate and future needs of a metropolitan area, region, state, or country. The system plan presents the recommendations for the general location and characteristics of new airports and heliports and the nature of expansion for existing ones to meet forecasts of aggregate demand. It includes the timing and estimated costs of development, and it relates airport system planning to the policy and objectives of the relevant jurisdiction. Its overall purpose is to determine the extent, type, nature, location, and timing of airport development needed to establish a viable, balanced, and integrated system of airports. The objectives of the system plan include: 1. The orderly and timely development of a system of airports adequate to meet present and future aviation needs and to promote the desired pattern of regional growth relative to industrial, employment, social, environmental, and recreational goals. 2. The development of aviation to meet its role in a balanced and multimodal transportation system to foster the overall goals of the area as reflected in the transportation system plan and comprehensive development plan. 3. The protection and enhancement of the environment through the location and expansion of aviation facilities in a manner which avoids ecological and environmental impairment. 4. The provision of the framework within which specific airport programs may be developed consistent with the short and long-range airport system requirements. 5. The implementation of land use and airspace plans which optimize the use of these resources in an often constrained environment. 6. The development of long-range fiscal plans and the establishment of priorities for airport financing within the governmental budgeting process. 7. The establishment of the mechanism for the implementation of the system plan through the normal political framework, including the necessary coordination between governmental agencies, the involvement of both public and private aviation and non-aviation interests, and compatibility with the content, standards, and criteria of existing legislation. The Airport Master Plan An airport master plan is a concept of the ultimate development of a specific airport. The overall objective of the airport master plan is to provide guidelines for future development which will satisfy aviation demand and be compatible with the environment, community development, and other modes of transportation. More specifically, it is a guide for: 1. Developing the physical facilities of an airport 2. Developing land on and adjacent to the airport 3. Determining the environmental effects of airport construction and operations 4. Establishing access requirements 5. Establishing the technical, economic, and financial feasibility of proposed developments through a thorough investigation of alternative concepts 6. Establishing a schedule of priorities and phasing for the improvements proposed in the plan 7. Establishing an achievable financial plan to support the implementation schedule 8. Establishing a continuing planning process which will monitor conditions and adjust plan recommendations as circumstances warrant. The Airport Project Plan An airport project plan is a detailed plan of the specific development planned in the immediate future for an airport. The project plan focuses on specific elements of the airport master plan which are to be implemented in the short term. THE ELEMENTS OF AN AIRPORT PLANNING STUDY 1. Inventory 2. Forecasts a. Observe past and current trends of air travel demand. b. Inventory the variations in economic, social, and technological factors affecting air travel demand. c. Establish a relationship between travel demand and those factors found to be of significance in altering travel demand. d. Project the values of those factors affecting travel demand into the future. e. Use the model and the forecasts to obtain forecasts of future air travel demand. 3. Analysis of Capacity and Delay 4. Facility Requirements 5. Airport Site Selection a. Type of development of the surrounding area b. Atmospheric conditions c. Accessibility to ground transport d. Availability of land for expansion e. Presence of other airports and availability of airspace in the area f. Surrounding obstructions g. Economy of construction h. Availability of utilities i. Proximity to aeronautical demand 6. Land-use Planning 7. Environmental Impact Assessment 8. Economic and Financial Feasibility HOMEWORK: 1. Name and give the functions of two (2) international aviation organizations. 2. Compare the two levels of forecasts in aviation. 3. Differentiate economic feasibility from financial feasibility. AIRCRAFT CHARACTERISTICS RELATED TO AIRPORT DESIGN Aircraft weight Size Wingspan Passenger capacity Types of propulsion: 1. Piston engine applies to all propeller-driven aircraft powered by gasoline-fed reciprocating engines. 2. Turboprop refers to propeller-driven aircraft powered by turbine engines. 3. Turbojet refers to those aircrafts which are not dependent on propellers for thrust but which obtain the thrust directly from a turbine engine. When a fan is added in front or rear of a turbojet engine, it is referred to as a turbofan. A single row of blades is referred to as single-stage; two row of blades as multistage. Components of Aircraft Weight 1. Operating Empty Weight (OEW) is the basic weight of the aircraft including the crew and all the gear required for flight but not including the payload and fuel. 2. Zero-Fuel Weight (ZFW) is the weight above which all additional weight must be fuel, so that when the aircraft is in flight, the bending moments at the junction of the wing and fuselage do not become excessive. The term payload refers to the total revenue-producing load. This includes the weight of the passengers, baggage, mail, and cargo. The maximum structural payload is the maximum load which the aircraft is to certify to carry. Theoretically, the maximum structural payload is the difference between the ZFW and OEW. 3. Maximum Ramp Weight is the maximum weight authorized for ground maneuver including taxi and run-up fuel. 4. Maximum Structural Takeoff Weight is the maximum weight authorized at brake release for takeoff. It excludes taxi and run-up fuel and includes the OEW, trip and reserve fuel, and payload. 5. Maximum Structural Landing Weight is the structural capability of the aircraft in landing. A portion of the weight of an aircraft at the start of takeoff is composed of fuel. The fuel requirements can be segregated into two components: the amount of fuel needed to make the trip and the reserves required by regulations. The fuel for the trip depends on the distance to be traveled, speed, meteorological conditions, altitude at which the aircraft is flown, and payload. The fuel reserve is dependent on the distance to the alternate airport. Thus the aircraft weight is composed of the operating empty weight and the three variables of payload, trip fuel, and fuel reserve. On landing, the weight of an aircraft is the sum of the OEW, payload, and fuel reserve, assuming that the aircraft lands at its destination and is not diverted to an alternate airport. This landing weight cannot exceed the maximum structural landing weight of the aircraft. The takeoff weight is the sum of the landing weight and the trip fuel. This weight cannot exceed the maximum structural takeoff weight of the aircraft. Payload and Range “How far can an aircraft fly?” Payload Range Payload versus range depends on a number of factors such as meteorological conditions en route, flight altitude, speed, fuel, wind, and amount of reserve fuel. Point A, the range at maximum payload, designates the farthest distance R a that an aircraft can fly with a maximum structural payload. To fly a distance of R a and carry a payload of Pa, the aircraft has to take off at its maximum structural takeoff weight; however, its fuel tanks are not completely filled. Point B, the range at maximum fuel, represents the farthest distance Rb that an aircraft can fly if its fuel tanks are completely filled at the start of the journey. The corresponding payload that can be carried is P b. To travel the distance Rb, the aircraft must takeoff at its maximum structural takeoff weight. Therefore to extend the distance of travel from Ra to Rb, the payload has to be reduced in favor of adding more fuel. Point C represents the maximum distance that an aircraft can fly without any payload. This is sometimes referred to as the ferry range, and it is used, if necessary, for delivery of aircraft. To travel this distance Rc, the maximum amount of fuel is necessary, but since there is no payload, the takeoff weight is less than maximum. In some cases, the maximum structural landing weight may dictate how long an aircraft can fly with a maximum structural payload. If this is the case, line DE represents the tradeoff between payload and range which must occur, since the payload is limited by the maximum structural landing weight. The shape of the payload-versus=range curve would then follow line DEBC instead of ABC. D A Pa Payload E Pb B C Rd Ra Re Rb Rc Range Example. The weight characteristics of a commercial aircraft are given as follows: Aircraft Weight Characteristics Maximum structural takeoff weight 220,000 Maximum structural landing weight 198,000 Zero-fuel weight 182.513 Operating empty weight 125,513 Maximum structural payload 57,000 Fuel capacity 75,400 We assume that the regulations governing the use of this aircraft require only a 1.25-hr reserve in route service. The aircraft has an average route speed of 540 mi/hr and an average fuel burn of 22.8 lb/mi. Range at Maximum Payload MSTOW = OEW + Max. Structural Payload + Allowable Fuel 220,000 = 125,513 + 57,000 + Allowable Fuel Allowable Fuel = 37,487 lb Reserve fuel = 1.25 x 540 x 22.8 = 15,390 lb Allowable Fuel = route fuel + reserve fuel 37,487 = route fuel + 15,390 Route Fuel = 22,097 lb Range = 22,097 ÷ 22.8 = 969 mi LW = MSTOW – route fuel LW = 220,000 – 22,097 = 197,903 lb. Range at Maximum Fuel Fuel Capacity = 75,400 lb Max. Route Fuel = 75,400 – 15390 = 60,010 lb Range at Max. Fuel = 60,010 ÷ 22.8 = 2632 mi MSTOW = OEW + Max. Fuel + Allowable Payload 220,000 = 125,513 + 75,400 + Allowable Payload Allowable Payload = 19,087 lb Ferry Range is computed by determining the range the aircraft can fly by using all the fuel available fuel including the reserve fuel. Ferry Range = 75,400 ÷ 22.8 = 3307 mi. Turning Radii For determining aircraft positions on the apron adjacent to the terminal building and establishing the paths of aircraft at other locations in the airport, it is important to understand the geometry of movement of an aircraft. Turning radii are a function of the nose gear steering angle; the larger the angle, the smaller the radii. From the center of rotation, the distances to the various parts of the aircraft, such as the wing tips, nose, or tail, create a number of radii. The largest radius is the most critical. The maximum angles vary from 60° to 80°. Minimum turning radii are not used in practice very often because the maneuver produces excessive tire wear and in some instances results in scuffing of the pavement. Accordingly smaller angles on the order of 50° are more proper. Static Weight on the Main Gear and the Nose Gear For the design of pavements, it is normally assumed that 5% of the weight is supported on the nose gear and the remainder on the main gears. Thus if there are two main gears, each gear supports 47.5% of the total weight. For example, if the takeoff weight of an aircraft is 300,000 lb, each main gear is assumed to support 142,500 lb. If the main gear has four tires, it is assumed that each tire supports an equal fraction of the weight on the gear, in this case, 35,625 lb. Wing-tip Vortices Whenever the wings lift an aircraft, vortices form near the ends of the wings. The vortices are made up of two counter rotating cylindrical air masses about a wingspan apart, extending aft along the flight path. The velocity of the wind within these cylinders can be hazardous to other aircraft encountering them in flight. The tangential velocities in a vortex are directly proportional to the weight of the aircraft and inversely proportional to the speed. The more intense vortices are therefore generated when the aircraft is flying slowly near an airport. The winds created by the vortices are often referred to as wake turbulence or wake vortex. The rate at which vortices settle toward the ground is dependent to some extent on the weight of an aircraft. The heavier the aircraft, the faster the vortex will settle. 0 0 Time, min Calm 1 1 4 3 2 2 3 4 Semispan Time, min 0 0 Wind 1 1 3 2 2, 3, 4 Effect of Aircraft Performance on Runway Length The factors which have a bearing on runway length may be grouped into three general categories: 1. Performance requirements imposed by the government on aircraft manufacturers and operators. 2. The environs at the airport. 3. Those items which establish the operating takeoff and landing gross weights for each aircraft type. Performance Requirements Imposed by Government Transport aircraft are licensed and operated under the code of regulations known as the Federal Aviation Regulations (FAR). The regulations pertaining to turbine aircraft consider three general cases in establishing the length of a runway necessary for safe operations: 1. A normal takeoff in which all engines are available and sufficient runway is required to accommodate variations in liftoff techniques and the distinctive performance characteristics of these aircrafts. Runway (FS) – refers to the full-strength pavement. The normal case, all-engine takeoff defines a takeoff distance (TOD) which, for a specific weight of aircraft, must be 115% of the actual distance that the aircraft uses to reach a height of 35 ft (D35). Not all of this distance has to be of full-strength pavement; however, pilot techniques would never vary so greatly as to need all the distance before lifting off the ground. What is necessary is that all this distance be free from obstructions to protect against an overshooting takeoff. Consequently, the regulations permit the use of a clearway (CL) for part of this distance. A clearway is a rectangular area beyond the runway not less than 500 ft wide and not longer than 1000 ft, centrally located about the extended centerline of the runway and under the control of the airport authorities. The clearway is expressed in terms of a clearway plane, extending from the end of the runway with an upward slope not exceeding 1.25% above which no object nor any portion of the terrain protrudes, except that threshold lights may protrude above the plane if their height above the end of the runway is not greater than 26 in. and if they are located to each side of the runway. Up to one-half the difference between the 115% of the distance to reach the point of liftoff, the liftoff distance (LOD) and the takeoff distance may be clearway. The remainder of the takeoff distance must be full-strength pavement and is identified as the takeoff run (TOR). 2. Takeoff involving an engine failure in which sufficient runway is required to allow aircraft to continue the takeoff despite the loss of power or else to brake to a stop. For the engine-failure case, the regulation specifies that the takeoff distance required is the actual distance to reach a height of 35 ft (D35) with no percentage applied, as in the all-engine takeoff case. This recognizes the rarity of engine failure. The regulations again permit the use of a clearway, in this case up to one-half the difference between the liftoff distance and the takeoff distance, with the remainder being full-strength pavement. The regulations for piston-engine aircraft normally require full-strength pavement for the entire takeoff distance. The engine-failure case requires that sufficient distance also be available to stop the airplane rather than to continue the takeoff. This distance is referred to as the accelerate-stop distance (DAS). For piston- engine aircraft, only full-strength pavement was normally used for this purpose. The regulations for turbine-powered aircraft, however, recognize that an aborted takeoff is relatively rare and permit use of lesser-strength pavement, known as stopway (SW), for that part of the accelerate-stop distance beyond the takeoff run. The stopway is an area beyond the runway, not less wide than the width of the runway, centrally located about the extended centerline of the runway, and designated by the airport authorities for use in decelerating the aircraft during an aborted takeoff. To be considered as such, the stopway must be capable of supporting the airplane during an aborted takeoff without inducing structural damage to the aircraft. The required field length (FL) is generally made up of three components: the full-strength pavement (FS), the partial-strength pavement or stopway (SW), and the clearway (CL). 3. Landing in which sufficient runway is required to allow for normal variation in landing technique, overshoots, poor approaches, and the like. The regulations state that the landing distance (LD) needed for each aircraft using the airport must be sufficient to permit the aircraft to come to a full stop, the stop distance (SD), within 60 percent of this distance, assuming that the pilot makes an approach at the proper speed and crosses the threshold of the runway at a height of 50 ft. The above regulations for turbine-powered aircraft may be summarized for each case in equation form to find the required field length. It is apparent that both the takeoff distance and the accelerate-stop distance will depend on the speed the aircraft has achieved when an engine fails. The speed at which engine failure is assumed to occur is selected by the aircraft manufacturer and is referred to as the critical engine-failure speed, V1. If an engine actually fails prior to this selected speed, the pilot brakes to a stop. If the engine fails at a speed greater than this speed, the pilot has no choice bu to continue the takeoff. The Environs at the Airport Certain conditions at the airport can influence runway length. The more important of these conditions are temperature, surface wind, runway gradient, altitude of the airport, and condition of the runway surface. The effect of these conditions on runway length can only be approximated. Temperature The higher the temperature, the longer the runway required because high temperatures reflect lower air densities, resulting in lower output of thrust. The increase in runway length is not linear with temperature. The rate of increase at high temperatures is greater than at lower temperatures. For estimating purposes, the approximate variations of runway length with temperature which apply to turbine-powered transport between 59° to 90° F can be used. In this temperature range, the average increase in runway length varies from about 0.42 to 0.65 percent per degree Fahrenheit above the runway requirements at 59° F. The increase is 1 percent of runway length for every 1°C by which the airport reference temperature exceeds the temperature in the standard atmosphere for aerodrome elevation. Surface Wind The greater the headwind, the shorter the runway length; and also the greater tailwind, the longer the runway. For computing takeoff weight, regulations allow operators to use only ½ of the reported headwind. IF there is a tailwind, 1 ½ times the reported tailwind is used for the computation of takeoff weight, up to a maximum allowable tailwind of 10 knots. The effect of wind varies, depending in part on temperature and aircraft weight. For approximation purposes, a 5-know tailwind increases this length by about 7%. For airport planning purposes, it is desirable to use no wind, particularly if flight winds occur at the airport site. A 5 knot headwind reduces the takeoff length by about 3 percent, whereas a 5 knot tailwind increases this length by about 7 percent. Runway Gradient An uphill gradient requires more runway than a level or downhill gradient, the specific amount depending on the elevation of the airport and temperature. Studies indicate that the relationship between uniform gradient and increase or decrease in runway length is nearly linear. For turbine-powered aircraft, this amounts to 7-10% for each percent of uniform gradient. Airport design criteria limit the gradient to a maximum of 1 ½%. Since most runway profiles are not uniform, aircraft operators are allowed to substitute an average uniform gradient, which is a straight line joining the ends of the runways, as long as no intervening point along the actual path profile lies more than 5 ft. above or below the average line. FAA uses an effective gradient which is defined as the difference in elevation between the highest and lowest points on the actual runway profile divided by the length of the runway. For positive gradient 10 percent increase in runway length for each percent of grade increase. Altitude All other things being equal, the higher the altitude of the airport, the longer the runway required. This increase is not linear, but varies with weight and temperature. At higher altitudes, the rate of increase is higher than at lower altitudes. For planning purposes, an increase from sea level of 7% per 1000 ft. or 300m of altitude will suffice for most airport sites, except those that experience very hot temperatures or are located at high altitudes. Condition of Runway Surface Slush or standing water on the runway has an undesirable effect on aircraft operation. Slush is equivalent to wet snow. It has a slippery texture which makes braking extremely poor. Being a fluid, it is displaced by tires rolling through it, causing a significant retarding force, especially on takeoff. The retarding forces can get so large that the aircraft can no longer accelerate to takeoff speed. In the process, slush is sprayed on the aircraft, which further increases the resisting forces on the vehicle and can cause damage to some parts. From NASA and FAA experiments on the effect of standing water, jet operations are limited to no more than 0.5 inch of slush or water. It is therefore important to provide adequate drainage on the surface of the runway for the removal of water and a means of rapidly removing slush. When tires ride on the surface of the water or slush, the phenomenon is known as hydroplaning. When tires hydroplane, the coefficient of friction is on the order of wet ice and the steering ability is completely lost. According to tests made by NASA, the approximate speed at which hydroplaning develops may be determined by the formula: Vp = 10 P 0.5 where Vp = speed where hydroplaning develops, mi/hr P = tire inflation pressure, psi Sequence of runway length correction Taking off : 1) Elevation 2) Temperature 3) Slope 4) Surface wind 5) Surface condition Landing : Elevation Example: The following are data for an aircraft. Calculate the runway length. Runway required for landing at sea level in standard atmospheric conditions 2,100 m. Runway length for takeoff at MSL 2,500 m. Airport altitude 150 m. Airport reference temperature 24°C Temperature in the standard atmosphere for 150 m. 14.025°C Runway slope 0.5 % Solution Correction for elevation (2500 x 0.07 x 150/300) + 2500 = 2,587 m Correction for elevation and temperature [2587 x (24 – 14.025) x 0.01] + 2587 = 2,845 m Correction for elev., temp. and slope (2845 x 0.05 x 0.10) + 2845 = 2,985 m Correction for landing, elevation (2100 x 0.07 x150/300) + 2100 = 2,175 m ∴Actual runway length = 2985 m Ans. Airport Airside Capacity and Delay The effectiveness of a transportation system is commonly measured in terms of its ability to efficiently process the transported unit. Since the system performance is dependent upon the individual components of that system, it is usually necessary to evaluate these components to determine overall system capabilities. In cases where use of the system requires the sequential utilization of a group of processors, the overall efficiency of the system is usually limited by the characteristics of the least efficient component. Capacity and Delay Capacity refers to the processing capability of a service facility over some period. For a service facility to realize its maximum or ultimate capacity, there must be a continuous demand for service. However, in aviation, it is virtually impossible to have a continuous demand throughout the operating period of the system. Thus, the designer is faced with the problem of providing sufficient capacity to accommodate fluctuating demand with an acceptable level of service. Typically, the design specifications at an airport require that sufficient capacity be provided that a relatively high percentage of the demand will be subjected to some minimal amount of delay. To provide sufficient capacity to service a varying demand without delay will normally require facilities that are difficult to justify economically. Therefore, in design a level of delay acceptable from the perspectives of both the user and the operator is usually established, and system components of sufficient capacity are chosen to ensure that these delay criteria are met. Capacity estimates are useful for the initial screening of alternatives and for selecting those alternatives which should be subject to further analysis. When demand approaches capacity, delays to aircraft build up very rapidly. Because of economic factors, estimating the magnitude of delays often is more important for the justification and establishment of requirements for airfield improvements than the determination of capacity. The primary objective of capacity and delay studies is to determine the effective and efficient means to increase capacity and to reduce delay at airports. Percentage Distribution of Causes of Flight Delays Greater than 15min, 1985 and 1990 Cause 1985 1900 Weather 68 53 Terminal volume 12 36 Center volume 11 2 Runway-taxiway closures 6 4 National airspace system equipment 2 2 Other 1 3 Total Delayed Operations 334,000 404,000 Source: FAA Definitions of Capacity In airport planning, airfield capacity has been defined in two ways. One definition has been used extensively in the past in the USA: capacity is the number of aircraft operations during a specified time corresponding to a tolerable level of average delay. This is referred to as practical capacity. Another definition is that capacity is the maximum number of aircraft operations that an airfield can accommodate during a specified time when there is a continuous demand for service. The continuous demand for service means that there are always aircraft ready to take off or land. This definition has been referred to as the ultimate capacity, saturation capacity, and maximum throughput rate. Average Aircraft Delay Acceptable Level Average Practical Ultimate Demand Capacity Capacity The airfield its components The airfield is the system of components upon which aircraft operate. There are many factors that influence the capacity of an airfield. Capacity depends on the configuration of the airfield, environment in which the aircrafts operate, availability and sophistication of navigation aids, and air traffic control facilities and procedures. The most significant factor which affects runway capacity is the spacing between successive aircrafts. This spacing is dependent upon the appropriate air traffic rules, which are to large extent functions of weather conditions and aircraft size and mix. Mathematical formulation of delay The delay for runways used exclusively for arrivals was computed from the following equation: Wa a a 2 1 / a 2 21 a / a Where: Wa = mean delay to arriving aircraft λa = mean arrival rate of aircraft μa = mean service rate for arrival, or reciprocal of mean service time σa = standard deviation of mean service time of arriving aircraft The mean service time may be the runway occupancy time or the time separation in the air immediately adjacent to the runway threshold, whichever value is the larger. The model for departures is identical to that for arrivals except for a change in subscripts. The following equation is therefore used for the departure delay: Wd d d 2 1 / d 2 21 d / d Where: Wd = mean delay to departing aircraft λd = mean departure rate of aircraft μd = mean service rate for departures, or reciprocal of mean service time σd = standard deviation of mean service time of departing aircraft For mixed operations, arriving aircraft are normally given priority and the delay to these aircraft is given by the arrival equation. However, the average delay to departures in this situation can be found from the following equation: Wd d j 2 j 2 g f 2 f 2 21 d j 21 a f Air Traffic Control Definition of air traffic control The federal government prescribes two types of flight rules for air traffic. These are known as the visual flight rules (VFR) and instrument flight rules (IFR). In general, VFR are in effect when visual meteorological conditions prevail. In the other hand, IFR are in effect when instrument meteorological conditions prevail. In IFR conditions, safe separation between aircraft is the responsibility of air traffic control personnel, while in VFR conditions it is the responsibility of the pilot. Air traffic control monitors flights under VFR and intervenes only when apparent conflicts between aircrafts develop. This is known as passive control. Positive air control is exercised when IFR apply or when aircraft operating under VFR enter controlled airspace. Essentially these rules require the assignment of aircrafts to specific routes and altitudes and the maintenance of minimum separation between aircrafts. Visual Flight Rules. Aircrafts must maintain: o 3 mi visibility o Remain 500 ft below the clouds o 1000 ft above the clouds o 2000 ft horizontally from the clouds As the speed of the aircraft and the density of the traffic in the airspace increased, there was greater concern over the possibility of midair collisions. Accordingly, in certain parts of the airspace, IFR have been prescribed regardless of weather conditions. This is referred to as positive control airspace. Positive control airspace usually encompasses the airspace where high-speed jet aircrafts operate, and therefore it can include the airspace in the vicinity of a major airport, often designated as a terminal control area (TCA) or an airport radar service area (ARSA), as well as the airspace at and above 18,000 ft AMSL in which jets fly en route from one airport to another. The limits of positive control airspace can be extended by the FAA as necessary to ensure safe operations. The trend is toward more positive control. Terminal Control Areas A terminal control area consists of the controlled airspace extending upward from the surface or higher to specified altitudes, within which all aircrafts are subject to operating rules and equipment requirements specified in FAR part 91. Airport Radar Service Areas An airport radar service area (ARSA) is regulatory airspace around designated airports wherein ATC provides radar vectoring and sequencing on a continuous basis for all VFR and IFR aircraft. An ARSA consists of the airspace from the airport surface up to an elevation of 4000 ft above ground level from the airport surface within a radius of 5 nmi of the airport and from an elevation of 1200ft above the ground surface to an elevation of 4000 ft above the ground surface between 5 and 10 nmi radially from the airport. Pilots are required to establish radio contact with ATC before entering ARSA. ARSA services include: o Sequencing of all aircrafts to the primary airport o Standard IFR separations to IFR aircrafts o Traffic advisories and safety alerts between IFR and VFR aircrafts o Mandatory traffic advisories and safety alerts between VFR aircraft. Airspace Classifications Effective September 16, 1993, the FAA has reclassified its airspace designations as follows: Class A Airspace – Positive Control Areas. Airspace in which only IFR operations are permitted. Pilots must be instrument-rated and have ATC clearance to enter this airspace. A transponder with altitude reporting capability is required. This airspace is designated from 18,000 ft above mean seal level to flight level 600 (60,000-ft pressure altitude). Class B Airspace – Terminal Control Areas. Airspace in which both VFR and IFR operations are permitted but an ATC clearance is required to enter the airspace. Aircraft must be equipped with a transponder with altitude reporting capability and must have a two-way radio with appropriate frequencies and a very high-frequency omnirange radio receiver for IFR operations. All aircrafts are subject to ATC separation, and VFR aircraft must remain clear of clouds. Class C Airspace – Airport Radar Service Areas. Airspace in which entry requires prior two- way radio communications with ATC and a transponder with altitude reporting capability. ATC provides traffic advisories and conflict resolution between all aircrafts. Class D Airspace – Airport traffic areas and control zones at airports with control towers. Airspace established around airports with operating air traffic control towers. Pilots must establish and maintain radio contact with ATC prior to entering and while operating in this airspace. Class E Airspace – General controlled airspace and control zones at airports without control towers. The airspace above 14,500 ft above mean sea level. Class G Airspace – Uncontrolled airspace. The airspace not under the control of any ATC facility. Major Components and Functions of the National Airspace System The national airspace system (NAS) consists of a network of navigational aids and a number of air traffic control facilities. The specific purpose of the air traffic control service is twofold: to prevent collisions between aircraft and on the maneuvering area between aircraft and obstructions and to expedite and maintain an orderly flow of air traffic. To properly manage the traffic in the system, the jurisdiction of control is divided into three parts: en route, terminal, and oceanic. Terminal air traffic control may be divided into terminal radar-approach control (TRACON) and air traffic control tower (ACTC) operations at an airport. Each part has a specific function. Air route traffic control centers Air route traffic control centers (ARTCC) have the responsibility of controlling the movement of en route aircraft along the airways and jet routes and in other parts of the airspace. Each of the 20 air traffic control centers within the continental US has control of a definite geographical area which may be bigger than 100,000 mi 2. At the boundary point, which marks the limits of the control area of the center, control of aircraft may be transferred to an adjacent center or an approach control facility, or radar service may be terminated and aircraft using VFR are free to contact the next center. Air traffic control centers can also provide approach control service to non-towered airports and to non- terminal radar-approach control airports. The ARTCC is concerned primarily with the control of aircraft operating under instrument flight rules. Under IFR pilots are required to file a flight plan indicating the route and altitude they desire to fly. The ARTCC will then check to determine whether the flight plan can be approved so that a safe separation between aircraft can be ensured. Changes in flight plans en route are permitted if approved by each ARTCC along the route of the flight. o Victor airways extend from 1200 ft above the terrain to, but not including 18,000 ft AMSL. o Jet routes extend from 18,000 ft to 45,000 ft AMSL. Terminal approach control facility The terminal approach control facility monitors the air traffic in the airspace surrounding airports with moderate to high-density traffic. It has jurisdiction in the control and separation of air traffic from the boundary area of the air traffic control tower at an airport to a distance of up to 50 mi from the airport and to an altitude ranging up to 17,000 ft. This is commonly referred to as the terminal area. Where there are several airports in an urban area, one facility may control traffic to all the airports. In essence, the facility receives aircraft from the ARTCC and guides them to one of several airports. In providing this guidance, the facility performs the important function of metering and sequencing aircraft to provide uniform and orderly flow to airports. The terminal radar- approach control facility is referred to as TRACON. If the flow of aircraft is greater than what the facility can handle, traffic management and the air traffic system command center manipulate aircraft on the ground and en route to adjust the arrival flows to their destination airports. This may result in delays to departing aircraft or delays en route. In the past, such aircrafts were delayed by either reducing their speed en route or detaining them at specified radio fixes within the area of the destination facility. The latter method is referred to as stacking. In a stack, aircrafts navigate around a fix in a racetrack pattern, a holding pattern, and are separated vertically by 1000 ft intervals. Air traffic control tower The airport traffic control tower is the facility which supervises, directs, and monitors the arrival and departure traffic at the airport and in the immediate airspace within about 5 mi from the airport. The tower is responsible for issuing clearances to all departing aircraft; providing pilots with information on wind, temperature, barometric pressure, and operating conditions at the airport; and controlling all aircraft on the ground except in the maneuvering area immediately adjacent to the aircraft parking positions called the ramp area. Flight service stations The flight service stations (FSS) are located along the airways and at airports. FSS are not air traffic control facilities. Their principal function is to accept and close flight plans and to brief pilots, before flight and in flight, on weather, navigational aids, airports, and navaids that are out of commission, and changes in procedures and new facilities. A secondary function is to relay traffic control messages between aircraft and the appropriate control facility on the ground. Air Traffic Separation Rules Air traffic rules governing the minimum separation of aircraft in the vertical, horizontal or longitudinal, and lateral directions are established in each country by the appropriate government authority. Homework: 1. Research about the different separation rules such as the: o vertical separation in the airspace (VFR and IFR) o longitudinal separation in the airspace o Lateral separation in the airspace 2. What is GPS? Explain how the system works. Navigational Aids Aids to aerial navigation can be broadly classified into two groups: external aids and internal aids. External aids are those that are located on the ground and internal aids are those that are installed in the cockpit. Some aids are primarily for flying over the oceans, other aids are only applicable to flight over land masses, and still other aids can be used over either land or water. Some aids are used only during the en route portion of the flight, while other aids are necessary in terminal areas or near airports. External overland en route aids o Very high-frequency omnirange radio These stations are located on the ground and send out radio signals in all directions. Each signal can be considered as a course or a route, referred to as a radial, which can be followed by an aircraft. The VOR transmitter is a small square building topped with what appears to be a white derby hat. The range of VOR stations varies but is usually less than 200 nmi. Aircrafts equipped with a VOR receiver in the cockpit have a dial for tuning in the desired VOR frequency. A pilot can select the VOR radial or route to follow to the VOR station. o Distance-measuring equipment Distance-measuring equipment (DME) has been installed at nearly all VOR stations in the US. Those so equipped are called VORTAC facilities. The DME shows to the pilot the slant distance between the aircraft and a particular VOR station. o Air route surveillance radar These radars have a range of about 250 nmi. Its principal function is to provide air traffic controllers with a visual display of the position of each aircraft so they can monitor the spacing and intervene when necessary. However, radar can be and is used by air traffic controllers to guide aircraft whenever necessary. External overland terminal aids The principal aids in the terminal area are used for landing aircraft. o Instrument landing system The system consists of two radio transmitters located at the airport. One radio beam is called the localizer, and the other is the glide slope. The localizer indicates whether the aircraft is to the left or right of the correct alignment for approach to the runway. The glide slope indicates the correct angle of descent to the runway. Glide slopes measure on the order of 2° to 3° minimum to 7.5° maximum. To further help pilots on their ILS approach, two low-power fan markers, called ILS markers, are usually installed so that pilots will know just how far along the approach to the runway they have progressed. The first is called the outer marker (LOM) and is located about 3.5 to 5 mi from the end of the runway. The other is called the middle marker (MM) and is located about 3000 ft. from the end of the runway. When visibility is quite poor, an additional marker called the inner marker (IM) is located 1000 ft. from the end of the runway. This inner marker is places so as to alert pilots that they must have visual reference with the ground at that point, and if they do not, abandon the approach. When the plane passes over a marker, a light goes on in the cockpit and a high-pitched tone sounds. o Microwave landing system The ILS has a number of problems which have made development of more sophisticated landing systems necessary. The ILS is based on signals reflecting from the surface of the ground. Thus the area adjacent to the antennas must be relatively smooth and must be kept clear of any obstructions such as buildings and taxiing aircraft; otherwise the beams are distorted. The ILS provides only one path is space, which all aircrafts must follow if they are using the system. Some aircraft, particularly the STOL type can use a steeper approach angle. Instead of providing only one glide slope as the ILS does, the MLS provides for any desired routes as long as they are within an area that is 20° to 60° on each side of the runway centerline. The MLS is far less susceptible to interference from surrounding objects than the ILS. With the MLS, a pilot can choose any desired route to the runway at any glide slope within the vertical coverage of the system. o Airport surveillance radar To provide the TRACON and control tower operators with an overall picture of what is going on within the airspace surrounding the terminal, airport surveillance radar (ASR) has been installed in major airports. The ASR rotates 360°, and its primary range is from 30 to 60 miles. It shows the aircraft in their relative horizontal positions on the radarscope as blips. o Approach lighting systems The most critical point of approach to landing comes when the aircraft breaks through the overcast and the pilot must change from instrument to visual conditions. Lights are installed on the approach to the runways and on the runways themselves. These are generally termed approach lighting systems (ALS). o Airport surface detection This system gives the air traffic controller in the control tower a pictorial display of the runways, taxiways, and terminal area, with radar indicating the positions of aircraft and other vehicles moving on the surface of the airport. o Visual-approach slope indicators These systems, the VASI and PAPI provide, through a system of lights, the proper approach slope to the runway much the same as the glide slope of an ILS system. VASI systems are intended for day or night use during good conditions, and they cannot be used under poor visibility conditions. A refined version of the VASI is the precision approach slope indicator system. The system gives a more definitive indication of approach slope to the pilot and uses only a single set of electronic devices at one point down the runway. o Runway end identifier lights Runway end identifier lights are installed to give the pilot positive visual identification of the approach end of the runway when there are no approach lights. PLANNING AND DESIGN OF AIRSIDE FACILITIES Terminal Airspace Airfield surface system Runway AIRSIDE Holding Pad Exit Taxiway Taxiway system Apron-gate area The airport configuration is the number and orientation of runways and the location of the terminal area relative to the runways. The number of runways depends on the volume of traffic. The orientation depends on the direction of the prevailing wind patterns in the area, the size and shape of the area available for airport development, and land-use or airspace restrictions in the vicinity of the airport. The terminal building should be located so as to provide easy and timely access to runways. Runways Runways and connecting taxiways should be arranged so as to: 1. provide adequate separations between aircraft in the air traffic pattern 2. cause the least interference and delay in landing, taxiing, and takeoff operations 3. provide the shortest taxi distance possible from the terminal area to the ends of the runways 4. provide adequate taxiways so landing aircraft can exit the runways as quickly as possible and follow the shortest possible routes to the terminal area Geometric Specification of Runway System 1. The runway structural pavement supports the aircrafts’ structural load, maneuverability, and control stability. 2. The shoulder adjacent to the edges of the structural pavement resists jet blast erosion and accommodates maintenance and emergency equipment. 3. The runway strip is the area which includes the structural pavement, shoulders, blast pad and stopway, if provided. This area should be capable of supporting emergency and maintenance equipment as well as providing support for aircraft in case they veer off the pavement. 4. The blast pad is the area designed to prevent pavement erosion of the surfaces adjacent to the ends of runways due to jet blast or propeller wash. 5. The runway end safety area is an area intended to reduce accidents of aircraft undershooting or overrunning the runway. 6. The stopway is an additional length of pavement which extends beyond the end of the runway. The stopway pavement must have adequate strength to support occasional aircraft loading. 7. A clearway is an unobstructed and unpaved area, also beyond the end of the runway which is controlled and maintained by the airport authority. Taxiways Taxiways provide access between runways and the terminal area and service hangars. They should be arranged so that aircraft which have just landed do not interfere with aircraft taxiing to take off. Taxiways should be located at various points along runways, so landing aircraft can leave the runways as quickly as possible to clear them for use by other aircrafts; these are commonly referred to as exit taxiways or turnoffs. Thus, during peak hours, the capacity of a runway is dependent to a large degree on how quickly a landing aircraft can be vacated from a runway. Runways Configurations 1. Single Runway It has been estimated that the hourly capacity of a single runway in VFR conditions is somewhere between 50-100 operations per hour, while in IFR conditions, this capacity is reduced to 50-70 operations per hour, depending on the composition of the aircraft mix and navigational aids available. 2. Parallel Runways The capacities of parallel runway systems depend on the number of runways and on the spacing between them. The spacing between parallel runways is classified as close, intermediate, and far, depending on the centerline separation between two parallel runways. Close parallel runways are spaced from a minimum of 700 ft. to less than 2500 ft. In IFR conditions, operation of one runway is dependent on the operation of the other runway. Intermediate parallel runways are spaced from 2500 to less than 4300 ft. In IFR conditions, an arrival on one runway is independent of a departure on the other runway. Far parallel runways are spaced at least 4300 ft. apart. In IFR conditions, the two runways can be operated independently for both arrivals and departures. 3. Intersecting Runways Intersecting runways are runways oriented in different directions crossing each other. They are necessary when relatively strong winds come from more than one direction, resulting in excessive crosswinds when only one runway is provided. The capacity of two intersecting runways depends a great deal on the location of the intersection. The farther the intersection is from the takeoff end of the runway and the landing threshold, the lower the capacity. The highest capacity is achieved when the intersection is close to the takeoff and landing threshold. 4. Open-V Runways Runways in different directions which do not intersect are referred to as open-V runways. Like intersecting runways, open-V runways revert to a single runway when winds are strong from one direction. When winds are light, both runways may be used simultaneously. The strategy which yields the highest capacity occurs when operations are away from the V, referred to as a diverging pattern. When operations are toward the V, referred to as a converging pattern, the capacity is reduced. From the standpoint of capacity and air traffic control, a single-direction runway configuration is most desirable. All other things being equal, this configuration will yield the highest capacity compared with other configurations. For air traffic control, the routing of aircraft in a single direction is less complex than routing in multiple directions. In comparing the divergent configurations, the open-V runway pattern is more desirable than an intersecting runway configuration. If intersecting runways cannot be avoided, every effort should be made to place the intersection of both runways as close as possible to their thresholds and to operate the aircraft away from rather than toward the intersection. Holding Bays Holding bays are often referred to as run-up or warm-up pads, are necessary at or very near the ends of runways for piston aircraft to make final checks prior to takeoff and for all types of aircraft to await takeoff clearance. A holding bay should be designed to accommodate two to four aircrafts and to allow sufficient space for one aircraft to bypass one another. Holding Aprons Holding aprons are relatively small aprons placed at a convenient location near the terminal area at the airport for the temporary storage of aircraft. In situations where the number of gates may be insufficient, aircrafts are routed to a holding apron and are held there until a gate becomes available. Relation of Terminal Area to Runways The key to a desirable airport layout is to provide the shortest taxiing distances from the terminal area to the takeoff ends of the runway and to shorten the taxiing distance for landing aircraft as much as is practicable. Analysis of Wind An analysis of wind is essential for planning runways. As a general rule, the principal traffic runway at an airport should be oriented as closely as practicable in the direction of the prevailing winds. When landing and taking off, aircrafts are able to maneuver on a runway as long as the wind component at right angles to the direction of travel, the crosswind component, is not excessive. The maximum allowable crosswind depends on the size of the aircraft, wing configuration, and the condition of the pavement surface. The FAA recommends that runways be oriented so that aircraft may be landed at least 95% of the time with allowable crosswind components not exceeding specified limits based upon the airport reference codes. When the wind coverage is less than 95%, a crosswind runway is recommended. The allowable crosswind is 10.5 kn (12mi/hr) for airport reference codes A-I and B-I; 13 kn (15 mi/hr) for airport reference codes A-II and B-II; 16 kn (18.5 mi/hr) for airport reference codes A-III, B-III, C-I, C-II, C-III, and C-IV; 20 kn (23 mi/hr) for airport reference codes A-IV through D-VI. In some cases, two runways are required to achieve the 95% wind coverage criteria. The International Civil Aeronautics Organization (ICAO) also specifies that runways should be oriented so that aircraft may be landed at least 95% of the time with crosswind components of 20kn (23 mi/hr) for runways of 1500 m or more, 13 kn (15 mi/hr) for runways between 1200 and 1500m, and 10kn (11.5 mi/hr) for runways less than 1200 m long. The Wind Rose The directions of the runway or runways at an airport can be determined through graphical vector analysis on a wind rose. A standard wind rose consists of a series of concentric circles cut by radial lines on polar-coordinate graph paper. The radial lines are drawn to the scale of the wind magnitude such that the area between each pair of successive lines is centered on the wind direction. A template representing the crosswind component limits is also drawn to the same radial scale. By overlaying the template on the wind rose and rotating the centerline of the template through the origin of the wind rose, one may determine the percentage of time that a runway in the direction of the centerline of the template can be used such that the crosswind components do not exceed the allowable values. With the center of the wind rose as a pivot point, the template is rotated until the sum of the percentages included between the outer lines is a maximum. It should be noted that if a wind vector from a segment lies outside either outer line on the template for the given direction of the runway, that wind vector must have a crosswind component which exceeds the allowable crosswind component plotted on the template. When one of the outer lines on the template divides a segment of wind direction, the fractional part is estimated visually to the nearest 0.1%. Airport Lighting, Marking, and Signing Facilities: 1. Approach lighting 2. Runway threshold lighting 3. Runway edge lighting 4. Runway centerline and touchdown zone lights 5. Runway approach slope indicators 6. Taxiway edge and centerline lighting 7. Runway and taxiway marking systems 8. Taxiway guidance sign systems Functions: 1. Ground to air visual information required during landing 2. The visual requirements for takeoff and landing 3. The visual guidance for taxiing Requirements for Visual Aids Since the earliest days of flying, pilots have used ground references for navigation when approaching an airport, just as officers on ships at sea have used landmarks on shore when approaching a harbor. Pilots need visual aids in good weather as well as bad and during the day as well as the night. The key elements in the for the perspective view of the runway are the threshold, the centerline, the edges, and multiple parallel lines to increase the perspective The Aircraft Landing Operation An aircraft approaching a runway in a landing operation can be visualized as a sequence of operations involving a transient body suspended in a three-dimensional grid that is approaching a fixed two-dimensional grid. While in the air, the aircraft can be considered as a point mass in a three-dimensional orthogonal coordinate system, in which it has translation along three coordinate directions and rotation about the three axes. If the three coordinate axes are aligned horizontal, vertical, and parallel to the end of the runway, the directions of motion can be described as lateral, vertical, and forward. The rotations are normally called, pitch, yaw, and roll for the horizontal, vertical, and parallel axes, respectively. During a landing operation, pilots must control and coordinate all six degrees of freedom of the aircraft so as to bring it into coincidence with the desired approach or reference path to the touchdown point on the runway. To do this, pilots need translation information regarding the aircraft’s alignment, height, and distance; rotation information regarding pitch, yaw, and roll; and information concerning the rate of descent and the rate of closure with the desired path. How much the pilot sees on the ground Several parameters influence how much a pilot can see on the ground. One is the cockpit cutoff angle, α. This is the angle between the longitudinal axis of the fuselage and an inclined plane below which the view of the pilot is blocked by some parts of the aircraft. Normally, the larger the angle α, the more the pilot can see of the ground. Also important is the pitch angle, β, of the fuselage axis during the approach to the runway. Few aircrafts approach a runway with the fuselage angle horizontal; they are pitched either up or down. The larger the angle β, in a pitch-up attitude, the larger the angle α must be to have adequate over-the-nose vision. In the following figure, VR is the visual range or the maximum distance a pilot can see at some height above the runway h. The horizontal segment of the ground that a pilot can see is H. H = VR cos θ – h cot (α – β) and sin α = h / VR Note that for a fixed value of VR, the ground segment H increases as the height h of the eyes of a pilot above the ground decreases. Typical values of α range from 11° to 16°, and typical values of β are ±0.5°. It has also been found through experience that 3 seconds is approximately the minimum reaction time for a pilot to cause the aircraft to react after sighting a visual aid. If a minimum of 3 seconds is necessary for perception, pilot action, aircraft response, and checking the response, and if the approach speed of the aircraft is 150mi/hr, then the minimum horizontal segment on the ground should not be less than 660 ft. Visual Approach Slope Indicator As an aid in defining the desired glide path in relatively good weather conditions, an optical reference device located on the ground adjacent to the sides of a runway has been developed. There are a number of different VASI configurations depending on the desired visual range, type of aircraft, and whether large wide-bodied aircrafts will be using the runway. Each group of lights traverse to the direction of the runway is called a bar irrespective of the fact that it may be on one side or on both sides of the runway. A bar is made up of one, two, or three light units, referred to as boxes. The bar nearest to the runway threshold is referred to as the downwind bar, and the bar farthest from the runway threshold is referred to as the upwind bar. If pilots are on the proper glide path, the downwind bar appears white and the upwind bar appears red; if pilots are too low, both bars appear red; and if pilots are too high, both bars appear white. Precision Approach Path Indicator This system gives more precise indications to the pilot of the approach path of the aircraft and utilizes only one bar as opposed to the minimum of two bars required by the VASI system. The system consists of a unit with four lights on either side of the approach runway. By utilizing the color scheme, the pilot is able to ascertain five approach angles relative to the proper glide slope, compared to three with the VASI system. One problem with the VASI system has been the lack of an immediate transition from one color indication to another, resulting in shades of colors. The PAPI system solves this by providing an instant transition from one color indication to another as a reaction to the descent path of the aircraft. An advantage of the system is that it is a one-bar system as opposed to the two-bar VASI system. This results in greater operating and maintenance cost economies and eliminates the need for the pilot to look at two bars to obtain glide slope indications. Threshold Lighting During the final approach for landing, pilots must make a decision to complete the landing or “execute a missed approach.” The identification of the threshold is a major factor in pilot decisions to land or not to land. For this reason, the region near the threshold is given special lighting consideration. The threshold is identified at large airports by a complete line of green lights extending across the entire width of the runway, and at small airports by four green lights on each side of the threshold. Threshold lights in the direction of landing are green, but in the opposite direction these lights are red, to indicate the end of the runway. Line of green lights Runway Lighting After crossing the threshold, pilots must complete a touchdown and rollout on the runway. The runway visual aids for this phase of landing should be designed to give pilots information on alignment, lateral displacement, roll, and distance. The lights should be arranged to form a visual pattern that pilots can easily interpret. Runway edge lights These light fixtures are usually elevated. Each unit has a specially designed lens which projects two main light beams down the runway. Elevated runway lights are mounted of frangible fittings and project no more than 30in above the surface on which they are installed. They are located along the edge of the runway not more than 10ft from the edge of the full-strength pavement surface. The longitudinal spacing is not more than 200 ft. Runway edge lights are white except that in the last 2000 ft of an instrument runway in the direction of aircraft operations, these lights are yellow to indicate a caution zone. Runway centerline and touchdown zone lights As an aircraft proceeds over the approach lights, pilots are looking at relatively bright light sources on the extended runway centerline. The touchdown zone lights are white, consist of a three-bulb bar on either side of the runway centerline, and extend 3000 ft from the runway threshold or one-half the runway length if the runway is less than 6000 ft long. They are spaced at intervals of 100 ft, with the first light bar 100 ft from the runway threshold, and are located 36 ft on either side of the runway centerline. The centerline lights are spaced at intervals of 50 ft. They are normally offset a maximum of 2 ft from the centerline, to avoid the centerline paint line and to prevent the nose gear of the aircraft from riding over the light fixtures. These lights are also white, except for the last 3000 ft of the runway in the direction of aircraft operations, where they are color-coded. For the last 1000 ft, centerline lights are red, and for the next 2000 ft they are alternated red and white. Runway end identifier lights The system consists of a pair of synchronized white flashing lights located on each side of the runway threshold and is intended for use when there is adequate visibility. Taxiway Lighting Either after a landing or on the way to takeoff, pilots must maneuver the aircraft on the ground on a system of taxiways to and from the terminal and hangar areas. At a large airport, the taxiway system can be very complex; consequently, adequate lighting aids should be provided for taxiing at night and during the day when visibility is very poor. Two types of lights are used for the designation of taxiways. One type delineates the edges of taxiways and the other type delineates the centerline of the taxiway. Taxiway edge lights are blue whereas taxiway centerline lights are green. Taxiway edge lights are usually located at intervals of not more than 200 ft on either side of the taxiway. The exact spacing is influenced by the physical layout of the taxiways. Closer spacing is required on curves. Light fixtures are located not more than 10 ft from the edge of full-strength pavement surfaces. The lights cannot extend more than 30 in above the pavement surface. Research and experience have demonstrated that guidance from centerline lights is superior to that from edge lights, particularly in low-visibility conditions. Runway and Taxiway Marking To aid pilots in guiding the aircraft on the runways and taxiways, pavements are marked with lines and numbers. These markings are of benefit primarily during the day and at dusk; at night, lights are used to guide pilots in landing and maneuvering at the airport. White is used for all markings on runways, and yellow is used on taxiways and aprons. Runways The FAA has grouped runways for marking purposes into three classes: visual runways, non-precision- instrument runways, and precision-instrument runways. The visual runway is a runway intended solely for the operation of aircraft using visual-approach procedures. The non-precision-instrument runway has an existing precision-instrument approach procedure utilizing air navigation facilities with only horizontal guidance (VOR) for which a straight-in non-precision-instrument approach procedure has been approved. A precision-instrument runway has an existing precision-instrument approach procedure utilizing either an ILS or an MLS. The end of each runway is marked with a number which indicates the magnetic azimuth (clockwise from magnetic north) of the runway in the direction of operations. The marking is given to the nearest 10° with the last digit omitted. (L, R, C) The amount of runway marking required on a runway depends on whether the runway is used for VFR or IFR conditions. The required markings for a visual runway are the runway designation, centerline, threshold, fixed distance markings, and holding positions for taxiway-runway intersections. The required markings for a non- precision-instrument runway are the runway designation, centerline, threshold, fixed distance markings, and holding positions for taxiway-runway intersections and ILS critical areas. The required markings for a precision-instrument runway are the runway designation, centerline, threshold, fixed distance markings, touchdown zone, side stripes, and holding positions for taxiway-runway intersections and ILS critical areas. Runway designation markings consist of numbers and letters indicating the direction of the runway. These markings are white, 60 ft tall, and vary from 5 ft wide for the numeral 1 to 23 ft wide for the numeral 7. Runway threshold markings are white; consist of two groups of four longitudinal stripes 150 ft long, 12 ft wide, spaced 3 ft apart; and are located symmetrically located about the runway centerline. The center spacing between each group of four stripes is 16 ft. Runway centerline markings are white, are located on the centerline of the runway, and consist of a line of uniformly spaced strips and gaps. The stripes are 120 ft long and the gaps are 80 ft long. The minimum width of stripes is 12 in for visual runway, 18 in for non-precision-instrument runways, and 36 ft for precision-instrument runways. Runway touchdown zone markings are white and consist of groups of one, two, and three rectangular bars symmetrically arranged in pairs about the runway centerline. These markings begin 500 ft from the runway threshold. The bars are 75 ft long, 6 ft wide, with 5 ft spaces between them, and are longitudinally spaced at distances of 500 ft along the runway. Runway side stripes, consisting of continuous white lines along each side of the runway, provide contrast with the surrounding terrain or delineate the edges of the full-strength pavement. To prevent erosion of the soil, many airports provide a paved blast pad 150 to 200 ft long adjacent to the runway end. Similarly, some airport runways have a stopway, which is designed only to support aircraft during rare aborted takeoffs and is not designed as a full-strength pavement. Since these paved areas are not designed to support aircraft and yet may have the appearance of being so designed, markings are needed to show this. The markings for blast pads and stopways are colored yellow. Taxiways The centerline of the taxiway is marked with a single, continuous 6-in yellow line. When runways or taxiways are permanently or temporarily closed to aircraft, yellow crosses are placed on these trafficways. To assist air traffic control and pilots in maintaining the safe movement of aircraft on the ground in poor- visibility conditions and dense traffic situations, a system of surface movement control devices is being implemented at many airports. The most common system is a system of stop bars placed across taxiways to supplement taxiway holding position and intersection markings. This type of system is very helpful in preventing runway incursions. The stop bars are controlled in the air traffic control tower. The stop bars are placed across the taxiway at the point where aircrafts are to stop. The stop bars typically consist of lights showing the color red in the intended direction of travel. In some cases, the operation of the stop bars is controlled by induction loop sensors embedded in the pavement. These sensors consist of three induction loops: a loop on the taxiway to detect the presence of an aircraft approaching the stop bar, a loop beyond the stop bar to indicate that an aircraft has passed the stop bar, and a loop on the runway pavement beyond the threshold to indicate the presence of an aircraft. The taxiway centerline lights remain lighted only up to the first loop. When clearance is given for the aircraft to proceed beyond the stop bar, the stop bar lights are switched off by the air traffic controller. This also illuminates the taxiway centerline lights beyond the stop bar. Once the aircraft passes over the second loop, the stop bar is automatically activated again. When the aircraft passes over the third loop, the portion of the taxiway centerline lights between the stop bar and the runway is automatically turned off. Runway Distance-Remaining Signs Runway distance-remaining signs, sometimes called “distance-to-go” signs have been installed at many airports. These signs are placed on the side of a runway and provide the pilot with information on how much runway is left during takeoff or landing operations. These signs are placed at 1000 ft intervals along the runway in a descending sequential order. Normally, these signs consist of white numerals on a black background. The FAA recommends that the signs be configured in one of three ways. The preferred method of configuration, and the most economical, is to place double-faced signs on only one side of the runway. The second method is to provide a set of single-faced signs on either side of the runway to indicate the distance remaining when the runway is used in both directions. The advantage of this configuration is that the distance remaining is more accurately reflected when the runway length is not an even multiple of 1000 ft. The third method used double-faced signs on both sides of the runway. The advantage of this method is that the runway distance is displayed on both sides of the runway in each direction, which is helpful when a sign on one side needs to be omitted because of a clearance conflict. These signs should be illuminated anytime the runway edge lights are illuminated. Taxiway Guidance Sign System The primary purpose of a taxiway guidance sign system is to aid pilots in taxiing aircraft at an airport. The taxiway guidance system consists of four basic types of signs: mandatory instruction signs, which indicate that aircraft should not proceed beyond a point without positive clearance; location signs, which indicated the location of an aircraft on the taxiway or runway system and the boundaries of critical airfield surfaces; direction signs, which identify the paths available to aircraft at intersections; and destination signs, which indicated the direction to a particular destination. The efficient and safe movement of aircraft on the surface of an airport requires that a well-designed and standardized taxiway guidance sign system be provided at the airport. The system must provide the pilot with the capacity to readily determine the designation of any taxiway on which the aircraft is located, readily identify routings to a desired destination on the airport property, indicate mandatory aircraft holding positions, and identify the boundaries for aircraft approach area, ILS critical areas, runway safety areas, and obstacle-free zones. Developing taxiway designations The fist step in designing a proper taxiway guidance sign system is the development of a simple and logical method for designating taxiways. The FAA recommends that the following guidelines be followed: 1. Keep it simple and logical. 2. Letters of the alphabet should only be used for designating taxiways. Optimally, the designation of taxiways should start at one end of the airport and continue to the opposite end of the airport in a sequential manner. When there are more taxiways than letters of the alphabet, double-letter designations may be used. Numbers alone should never be used for taxiway designations since they can be confused with runway designations. The letters I and O should not be used since they could be confused with a runway number designation. The letter X should not be used for a taxiway designation since it might be confused with a designation for a closed taxiway or runway. 3. For a major taxiway with numerous stub exits, the short segments of the taxiway may be designated by the taxiway letter and an exit number, where the number is sequential along the taxiway route. 4. All separate and distinct taxiway segments should be designated. No separate or distinct taxiway segment should have the same designation as another taxiway. 5. Taxiway designations should be changed when there is no significant change in the direction or continuity of the taxiway. If a change is found necessary, the change should be made only at a taxiway intersection and should be appropriately signed. 6. Taxiways should not be designated by direction of travel or location. Designations such inner, outer, or parallel should be avoided. Types of taxiway signs 1. Mandatory instruction signs – denote an entrance to a runway, critical area, or prohibited area. They are used for holding-position signs for runway-taxiway and runway-runway intersections, ILS critical areas, and runway approach areas, and to designate areas where entry by aircraft is prohibited. These signs have white inscriptions on a red background and are installed on the left side of the runway or taxiway. Generally, arrows are not permitted on mandatory instruction signs unless arrows are necessary at taxiway- runway-runway intersections to indicate directions to these runways. For runway designation use, these signs normally contain both designations of the runway; the designation on the left is for the runway to the left, and that on the right is for the runway to the right. 2. Location signs – used to identify the runway or taxiway on which an aircraft is located. These signs consist of a yellow inscription and border on a black background. Location signs are also used to identify the boundary of the runway safety area or obstacle-free zones or the ILS critical area for a pilot exiting a runway. In the latter cases, the signs consist of a black inscription and border on a yellow background, and the inscription on the sign is the same as relevant holding line marking. 3. Direction signs – used to indicate the directions of other taxiways leading out of an intersection. These signs are used as taxiway direction signs and runway exit signs. The signs have black inscriptions and borders on a yellow background and always contain arrows. The arrows are oriented in the approximate direction of the turn required. 4. Destination signs – have black inscriptions and black borders on a yellow background and always contain arrows. These signs indicate the general direction to a remote location at the airport, such as inbound destination and they are generally not required where taxiway direction signs are used. Outbound destination signs are used to identify directions to the takeoff runways. These routes normally begin at the entrance to a taxiway from the apron area. More than one runway number may be used, separated by a dot, if the route is common to more than one runway. Inbound destination signs are often used to indicate the general direction to major airport facilities. These signs should consist of a minimum of three letters to avoid confusion with taxiway guidance signs. 5. Information signs – indicate a noise abatement procedure to a pilot ready to take off on a specific runway. These signs should have black inscriptions on a yellow background, and they are not required to be lighted.