Tomographic Nuclear Imaging Lecture Notes PDF
Document Details
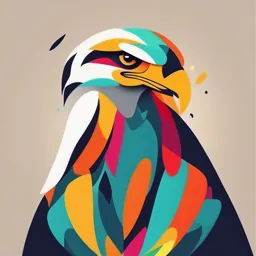
Uploaded by AppreciableDouglasFir
University of Nicosia Medical School
Dr. Anastasia Hadjiconstanti
Tags
Summary
These lecture notes provide an overview of tomographic nuclear imaging techniques, specifically focusing on Anger cameras, SPECT, and PET. The document explains the fundamentals of these imaging modalities, including components, operations, and applications in medical diagnostics.
Full Transcript
TOMOGRAPHIC NUCLEAR IMAGING Dr. Anastasia Hadjiconstanti Acknowledgements: Dr. Constantinos Zervides LECTURE LOB’S 44. DESCRIBE THE IMPORTANCE OF THE ANGER CAMERA IN NUCLEAR MEDICINE. 45. EXPLAIN THE PRINCIPLES OF SPECT AND LIST APPLICATIONS. 46. EXPLAIN THE PRINCIPLES OF PET AND LIST APPLICATIONS....
TOMOGRAPHIC NUCLEAR IMAGING Dr. Anastasia Hadjiconstanti Acknowledgements: Dr. Constantinos Zervides LECTURE LOB’S 44. DESCRIBE THE IMPORTANCE OF THE ANGER CAMERA IN NUCLEAR MEDICINE. 45. EXPLAIN THE PRINCIPLES OF SPECT AND LIST APPLICATIONS. 46. EXPLAIN THE PRINCIPLES OF PET AND LIST APPLICATIONS. 47. DESCRIBE THE MULTI-MODALITY IMAGING USED IN NUCLEAR MEDICINE. ANGER CAMERA I The daily workload in a nuclear medicine department consists of functional imaging of organs. This is accomplished using a large scintillation device. In the 1950s, Hal Oscar Anger developed the basic design of the modern nuclear medicine camera. ANGER CAMERA II ANGER CAMERA III The Anger camera was a significant improvement over its predecessor. The components of the imaging system are: collimator, camera head which encompasses: crystal, Photomultiplier tubes (PMTs) electronics computers for image acquisition and image processing. ANGER CAMERA: COLLIMATOR I A collimator restricts the rays from the source so that each point in the image corresponds to a unique point in the source. Collimators are composed of thousands of precisely aligned holes. They are usually depicted in cross section. Nuclides emit gamma ray photons in all directions. The collimator allows only those photons traveling directly along the long axis of each hole to reach the crystal. ANGER CAMERA: COLLIMATOR II Photons emitted in any other direction are absorbed by the septa between the holes. Without a collimator in front of the crystal, the image would be unclear. ANGER CAMERA: COLLIMATOR III There are several types of collimators, designed to channel photons of different energies. By an appropriate choice of collimator, it is possible to magnify or reduce images. It is also possible to select between imaging quality and imaging speed. ANGER CAMERA: CAMERA HEAD I The camera head contains: the crystal, PMTs, and associated electronics. The head housing envelopes and shields these internal components. Typically, it includes a thin layer of lead. A gantry supports the heavy camera head. ANGER CAMERA: CAMERA HEAD II The crystal for an imaging camera is a large slab of thallium-“doped” NaI crystal. The thickness of a crystal affects its resolution as well as its sensitivity. Thicker crystals have higher sensitivity but lower resolution. This is because gamma rays may be scattered and absorbed farther from the point at which they entered the crystal. ANGER CAMERA: CAMERA HEAD III Sixty or more PMTs may be attached to the back surface of the crystal using light-conductive jelly. Each of these functions very much like the single PMT. The amount of light received by a photomultiplier tube is related to the proximity of the tube to the site of interaction of the gamma ray in the crystal. The PMT closest to the site of interaction receives the greatest number of photons and generates the greatest output pulse. The tube farthest from the nuclide source receives the fewest light photons and generates the smallest pulse. ANGER CAMERA: CAMERA HEAD IV An image can be formed purely from the points corresponding to the PMT with the highest output at each photon interaction. This means that the number of resolvable points is limited to the total number of PMTs (≈128 per camera). A positioning algorithm improves the resolution by combining signals from adjacent tubes. ANGER CAMERA: CAMERA HEAD V ANGER CAMERA: CAMERA HEAD VI The electrical pulse generated by each PMT is first digitized by an analog-to-digital converter (ADC). These digital values are then transmitted to the positioning algorithm. This is a part of the computer-processing equipment in the camera head. The computer or positioning algorithm knows the location of each PMT on the surface of the crystal. It then estimates the site of the gamma ray interaction in the crystal by weighing in the digital value of the amount of light each PMT receives. ANGER CAMERA: PULSE-HEIGHT ANALYSER I Following each gamma photon interaction in the crystal, the sum of the digital outputs from all the PMTs is proportional to the energy of the gamma photon striking the crystal. This summed output is called the Z-pulse. The pulse-height analyzer accepts only those Z-pulses that correspond to the gamma energy of interest. Each accepted Z-pulse and its location are stored in the computer. ANGER CAMERA: PULSE-HEIGHT ANALYSER II ANGER CAMERA: COMPUTERS I Nuclear medicine computers are used for the: acquisition, storage, and processing of data. The image data are stored in digital form. For each Z-pulse that is accepted by the pulse-height analyzer, a count is added to the storage location corresponding to its x, y location determined by the positioning circuit. The data storage can be visualized as a matrix. Each position in the matrix corresponds to a pixel, which has a unique address composed of the row and column of its location. Data are digitized by assigning a matrix position to every accepted photon. ANGER CAMERA: COMPUTERS II ANGER CAMERA: COMPUTERS III The greater the number of pixels: the smaller is each pixel for a given field of view, and the better preserved is the resolution of the image. SPECT I Single-photon emission computed tomography (SPECT) cameras acquire multiple planar views of the radioactivity in an organ. The data are then processed mathematically to create cross-sectional views of the organ. SPECT utilizes the single photons emitted by gamma-emitting radionuclides such as: 99mTc 111In 123I (technetium), (indium), and (iodine). SPECT II The simplest camera design for SPECT imaging is like that of an Anger camera with two additional features: 1. the SPECT camera is constructed so that the head can rotate about the patient to acquire multiple views. 2. It is equipped with a computer that integrates the multiple images to produce the crosssectional views of the organ. SPECT III SPECT IV SPECT V SPECT VI SPECT is most used to help diagnose or monitor: brain disorders, dementia, seizures, clogged blood vessel, epilepsy, head injuries, heart problems, clogged coronary arteries, reduced pumping efficiency, bone disorders, areas of bone healing, cancer progression. SPECT VII SPECT VIII PET I Positron emission tomography (PET) differs from SPECT in one fundamental way: PET radionuclides are positron emitters. After the decay event, the positron travels a very short distance before colliding with an electron. The annihilation event creates two gamma photons with an energy of 511 keV each. The gamma photons are traveling in exactly opposite directions. PET scanners use complete detector rings. Individual detectors are PMTs with a conversion layer and a collimator, much like the individual elements of an Anger camera. PET II PET III PET IV PET IMAGING CONCEPTS PET V Unlike SPECT, the almost-simultaneous detection of two γ -photons can be expected, and single events can be rejected as noise. This method is referred to as coincidence detection. Furthermore, the line that connects the two PMTs that recorded the event serves as the direction of projection. As a result, PET has a dramatically better signal-to-noise ratio than SPECT. Coincidence detection requires ultrafast electronics. Any detector that records an event opens a gate for very short time window (5 to 15 ns). PET VI If a second detector records an event during this window, a coincidence event is recorded. Otherwise, the initial event is rejected. Noise is recorded when: two independent events occur within the detection window, or when a photon is scattered and changes its direction. As a result, the sensitivity of a PET scanner is several orders of magnitude higher than that of a SPECT scanner. PET VII EVENTS IN PET SCANNERS PET VIII EVENTS IN PET SCANNERS PET IX EVENTS IN PET SCANNERS PET X EVENTS IN PET SCANNERS PET XI PET is used to inspect blood flow, oxygen intake and metabolism of organs and tissues. PET scans are most used to detect: cancer, brain disorders, central nervous system problems. heart problems, PET scan shows problems at the cellular level and gives a better inside into diseases like: coronary artery disease, memory disorders, brain tumors, seizures. PET XII PET XIII PET Scan https://youtu.be/oySvkmezdo0 Radionuclides used in Nuclear Medicine MULTI MODALITY IMAGING I Both SPECT and PET provide functional information. It is desirable to know the location of radiopharmaceutical accumulation as exactly as possible. This will allow relating the activity to a specific organ or site. For this reason, a structural image is often acquired with CT or MRI. The reconstructed activity map can then be superimposed over the structural image. More commonly, a structural CT or MRI image is taken, followed by a separate SPECT or PET scan. MULTI MODALITY IMAGING II If patient positioning was not carefully reproduced between studies, nuclear medicine and CT/MRI images did not match. The resulting misregistration led to inaccuracies. To correct positioning errors, PET or SPECT camera images and CT/MRI images were fused manually or by computer software or both. Specialized dual modality scanners exist: SPECT/CT scanners, SPECT/MRI scanners, PET/CT scanners, PET/MRI scanners. They are not widely used because of their extremely high costs. MULTI MODALITY IMAGING III MULTI MODALITY IMAGING IV MULTI MODALITY IMAGING V SUMMARY I Nuclear imaging is related to X-ray and CT imaging in that it uses radiation. However, unlike X-ray based imaging modalities, radioactive compounds are injected into the body as radiation sources. These radioactive compounds are typically linked to pharmacologically active substances that accumulate at specific sites in the body. With either projection techniques or volumetric computerized image reconstruction, the spatial distribution of the radiopharmaceutical can be determined. In this fashion, metabolic processes can be imaged and used for a diagnosis. SUMMARY II Three-dimensional reconstructions are obtained in a fashion similar to CT, leading to a modality called SPECT. A parallel technology, PET, makes use of positron emitters that cause coincident pairs of gamma photons to be emitted. Its detection sensitivity and signal-to noise ratio are better than SPECT. Both SPECT and PET have a significantly lower resolution than CT with voxel sizes not much smaller than 1cm. Often, SPECT or PET images are superimposed with CT or MR images to provide a spatial reference. REFERENCES Authors E.B. Podgorsak R.K.Hobbie and B.J.Roth C. Martin, P. Dendy and R. Corbertt M.A. Haidekker A.B. Wolbarst, P. Capasso and A.R. Wyant R. A. Powsner, M. R. Palmer and E. R. Powsner R. A. Powsner and E. R. Powsner Title Radiation Physics for Medical Physicists Intermediate Physics for Medicine and Biology Medical Imaging and Radiation Protection for Medical Students and Clinical Staff Edition Publisher Year ISBN 3rd Edition Springer 2016 9783319253824 5th Edition Springer 2015 9783319126814 2nd Edition The British Institute of Radiology 2014 9780905749549 Medical Imaging Technology 1st Edition Springer 2013 9781461470724 Medical Imaging: Essentials for Physicians 1st Edition Wiley-Blackwell 2013 9780470505700 Essentials of Nuclear Medicine Physics and Instrumentation 3rd Edition Wiley-Blackwell 2013 9780470905500 Essential Nuclear Medicine Physics 2nd Edition Wiley-Blackwell 2008 9780470752869