Lecture 7.1: Introduction to Nuclear Medicine PDF
Document Details
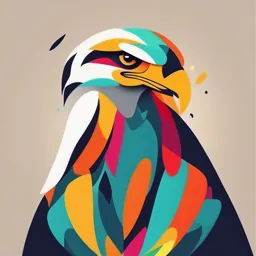
Uploaded by AppreciableDouglasFir
University of Nicosia Medical School
Dr. Anastasia Hadjiconstanti
Tags
Summary
These lecture notes provide an introduction to nuclear medicine, covering topics such as radioactive decay modes, radiopharmaceuticals, and associated imaging techniques. The material includes detailed explanations and examples.
Full Transcript
INTRODUCTION TO NUCLEAR MEDICINE Dr. Anastasia Hadjiconstanti Acknowledgements: Dr. Constantinos Zervides LECTURE LOB’S 42. DESCRIBE THE MODES OF RADIOACTIVE DECAY. 43. EXPLAIN THE DESIRABLE ATTRIBUTES OF A RADIOPHARMACEUTICAL. INTRODUCTION I Nuclear medicine consists of a range of diagnostic and th...
INTRODUCTION TO NUCLEAR MEDICINE Dr. Anastasia Hadjiconstanti Acknowledgements: Dr. Constantinos Zervides LECTURE LOB’S 42. DESCRIBE THE MODES OF RADIOACTIVE DECAY. 43. EXPLAIN THE DESIRABLE ATTRIBUTES OF A RADIOPHARMACEUTICAL. INTRODUCTION I Nuclear medicine consists of a range of diagnostic and therapeutic procedures that use radioisotopes. For diagnostic work it is usually necessary for the radioisotope to emit gamma rays. This is because these can penetrate tissue and be detected outside the body. This allows for their distribution in the body to be determined. If the diagnostic test involves measuring samples taken from a patient, β particle emitters can be used. For therapeutic work it is usually more desirable for the radioisotope to emit β particles. INTRODUCTION II They are preferred because: they have a short range in tissue and can deliver a high radiation dose to the location of the radioisotope. For example, radioiodine used for thyroid gland treatments. INTRODUCTION III Henri Becquerel discovered natural radioactivity in 1896. He opened a whole new world for physics and introduced nuclear physics and particle physics. The early investigators explained the macroscopic kinetics of radioactive decay. The work of Marie Sklodowska-Curie and Pierre Curie, Ernest Rutherford and Frederick Soddy, paved the way. It took several decades until the various radioactive decay modes were fully understood. INTRODUCTION IV Radioactive nuclides, either occur in nature or they are man made. They are unstable and try to reach more stable nuclear configurations. This is done through various processes of spontaneous radioactive decay. Radioactive decay processes are divided into six main categories: Alpha (α) decay. Spontaneous (SF). fission Beta (β) decay. Gamma (γ) decay and internal conversion (IC). Proton emission (PE) decay. Neutron emission (NE) decay. INTRODUCTION V Nuclear transformations are usually accompanied by emission of energetic particles. The particles released in the various decay modes are as follows: α particles in α decay, Atomic orbital electrons in IC, Electrons in β− decay, Neutrons in spontaneous fission and in neutron emission decay, Positrons in β+ decay, Neutrinos in β+ decay, Heavier nuclei in spontaneous fission, Antineutrinos in β− decay, γ-rays in γ decay, Protons in proton emission decay. INTRODUCTION VI INTRODUCTION VII In each nuclear transformation several physical quantities must be conserved. The most important of these quantities are: Total energy Atomic number Momentum Atomic mass nucleons). number (number of Charge In low atomic number (Z) elements, nuclear stability is achieved when the number of neutrons (N) is approximately equal to the number of protons (Z). As the atomic number Z increases, N/Z increases from 1 to about 1.5. INTRODUCTION VIII If a nucleus has N/Z too high for nuclear stability, it has an excess number of neutrons and is called neutron rich. It decays through conversion of a neutron into a proton and emits an electron and antineutrino. This process is referred to as β− decay. If the N/Z ratio is extremely high, a direct emission of a neutron is possible. If a nucleus has N/Z that is too low for nuclear stability, it has an excess number of protons and is called proton rich. It decays through conversion of a proton into a neutron and emits a positron and a neutrino (β+ decay). INTRODUCTION IX Alternatively: the nucleus may capture an orbital electron, transform a proton into a neutron and emit a neutrino (electron capture). A direct emission of a proton is also possible. This is less likely, unless the nuclear imbalance is very high. INTRODUCTION X INTRODUCTION XI ALPHA DECAY I Alpha (α) decay was the first mode of radioactive decay detected and investigated during the 1890s. It is characterized by a nuclear transformation. An unstable parent nucleus P attains a more stable nuclear configuration (daughter D) through ejection of an α-particle. This α-particle is a helium 4 nucleus that has a very stable configuration. In α decay the number of protons and neutrons is conserved by producing a He nucleus (αparticle) and lowering the parent’s A and Z by 4 and 2, respectively. 𝐴 𝑍𝑃 → 𝐴−4 𝑍−2𝐷 + 42𝐻𝑒 ALPHA DECAY II When an α-particle is emitted by the radioactive parent (Z, A) nucleus: the atomic number Z of the parent decreases by 2 and it sheds two orbital electrons from its outermost shell. The energetic α-particle slows down through the absorber. This allows it to capture two electrons from its surroundings to become a neutral He atom. ALPHA DECAY III α particles have a range: in air of about 1 cm to 10 cm and in tissue of about 10−3 cm and 10−2 cm. The most important example of radioactive decay in α decay is the decay of radium-226 (halflife of 1602 years) into radon-222 (half-life of 3.8 days). 226 88𝑅𝑎 → 222 86𝑅𝑛 +𝛼 BETA DECAY I The term β decay includes modes of radioactive decay in which: the atomic number Z of the parent nuclide changes by one unit (±1), while the mass number A remains constant. The number of nucleons and the total charge are both conserved in the β decay processes. The daughter D can be referred to as an isobar of the parent P. BETA DECAY II Three processes fall into the category of β decay: 1. Beta minus (β−) decay with the following characteristics: 𝒏 → 𝒑 + 𝒆− + 𝝂𝒆 , 𝑨𝒁𝑷 → 𝑨 𝒁+𝟏𝑫 + 𝒆− + 𝝂𝒆. 2. Beta plus (β+) decay with the following characteristics: 𝒑 → 𝒏 + 𝒆+ + 𝝂𝒆 , 𝑨𝒁𝑷 → 𝑨 𝒁−𝟏𝑫 + 𝒆+ + 𝝂𝒆. 3. Electron capture with the following characteristics: 𝒑 + 𝒆− → 𝒏 + 𝝂𝒆 , 𝑨𝒁𝑷 + 𝒆− → 𝑨 𝒁−𝟏𝑫 + 𝝂𝒆. BETA DECAY III In many cases, β decay of a parent nucleus does not lead directly to the ground state of the daughter nucleus. It leads to an unstable or even metastable excited state of the daughter. The excited state de-excites through emission of γ-rays or through emission of internal conversion electrons. β decay can only take place when the binding energy of the daughter nucleus exceeds the binding energy of the parent nucleus. BETA MINUS DECAY I Several radionuclides decaying by β− decay are used in medicine for: external beam radiotherapy and brachytherapy. The parent nuclide decays by β− decay into an excited daughter nuclide. This nuclide instantaneously or through a metastable decay process decays into its ground state. By doing so it emits the excitation energy in the form of γ-ray photons. These photons are then used for radiotherapy. BETA MINUS DECAY II Of the over 3000 natural or artificial radionuclides known, only a few are suitable for use in radiotherapy. Of these practically only cobalt 60 is used. The unstable cobalt 60 radionuclide has a half-life of 5.26 years. It decays into an excited nickel 60 nuclide that decays instantaneously into its ground state and emits two γ-rays. 60 27𝐶𝑜 → 60 28𝑁𝑖 + 𝑒 − + 𝜈ഥ𝑒 + 𝑄𝛽− (2.82 𝑀𝑒𝑉) BETA PLUS DECAY I The β+ decay is characterized by the production of positrons. Radionuclides undergoing β+ decay are often called positron emitters. They are used in medicine for functional imaging with positron emission tomography (PET). An example for a simple β+ decay is the decay of nitrogen-13 into carbon-13 with a half-life of 10 min. 13 7N → 13 6C + e+ + νe + Q β+ (1.2 MeV) Nitrogen-13 is a proton-rich radionuclide produced in a cyclotron. BETA PLUS DECAY II Nitrogen-13 labeled ammonia is injected intravenously and is mainly used in: cardiac imaging for diagnosis of coronary artery disease and myocardial infarction, liver imaging and brain imaging. BETA PLUS DECAY III The β+ decay of fluorine-18 into oxygen-18 with a half-life of 110 min is an important practical example of the β+ decay. Fluorodeoxyglucose (FDG) labeled with radionuclide fluorine-18 is a sugar compound that can be injected intravenously into a patient for use in PET functional imaging. Based on demonstrated areas of increased glucose metabolism the FDG PET scan: Can detect malignant disease. Can distinguish benign from malignant disease. Can be used for staging of malignant disease. Can be used for monitoring response to therapy of malignant disease. GAMMA DECAY I α decay as well as β decay may produce a daughter nucleus in an excited state without expending the full amount of the decay energy available. The daughter nucleus will reach its ground state through one of the following two processes: 1. Emit the excitation energy in the form of a γ photon in a decay process referred to as γ decay. 2. Transfer the excitation energy to one of its associated atomic orbital electrons in a process called internal conversion (IC). GAMMA DECAY II GAMMA DECAY III In most radioactive α or β decays the daughter nucleus de-excitation occurs instantaneously. Thus, we refer to the emitted γ-rays as if they were produced by the parent nucleus. For example, for the cobalt-60 β−decay into nickel-60, the γ-rays following the β−decay originate from nuclear deexcitations of nickel-60. For convenience, we refer to these γ-rays as the cobalt-60 γ-rays. GAMMA DECAY IV In certain α or β decays, the excited daughter nucleus does not immediately decay to its ground state. It de-excites with a time delay of the order of several minutes or several hours. The excited state of the daughter is then referred to as a metastable state and the process of de-excitation is called an isomeric transition. The nucleus in a metastable state is identified with a letter “m” next to the atomic mass number designation: barium-137m or 137m 56Ba with a half-life of 2.552 min. GAMMA DECAY V The term isomer is used for designation of nuclei that have the same atomic number Z and same atomic mass number A but differ in energy states. γ decay stands for nuclear de-excitation by emission of a γ-ray photon or by internal conversion. γ decay only implies emission of γ photons. The energy of γ-rays emitted is determined by the energy level structure of the radionuclides. GAMMA DECAY VI The γ decay process may be represented as follows: 𝑨 ∗ 𝑨 𝐗 → 𝒁 𝒁𝐗 + 𝛄 + 𝑸𝜸 Nuclear de-excitation in which the de-excitation energy is transferred from the parent nucleus almost in full to an orbital electron of the same atom is called internal conversion (IC). The process is represented as follows: 𝑨 ∗ 𝑨 + 𝒁𝐗 → 𝒁𝐗 +𝒆− + 𝑸𝑰𝑪 → 𝑨𝒁𝑿 SPONTANEOUS FISSION I Nuclei with very large atomic mass numbers A may disintegrate by splitting into two nearly equal fission fragments. They also simultaneously emit 2–4 neutrons. This decay process is called spontaneous fission (SF). It is accompanied by the release of a significant amount of energy. Spontaneous fission follows the same process as neutron-induced nuclear fission. There only difference is that SF is not self-sustaining. SF can occur only in thorium, protactinium, uranium and transuranic elements (Z>92). SPONTANEOUS FISSION II Generally, the transuranic elements are man-made and synthesized in nuclear reactions. Spontaneous fission is a competing process to α decay. The higher the atomic mass number above uranium-238, the more prominent the spontaneous fission and the shorter the half-life. SF is a limiting factor in how high in atomic number Z and atomic mass number A one can go in producing new elements. PROTON EMISSION I Proton-rich nuclides normally approach stability through β+ decay or α decay. In the extreme case of a very large proton excess, a nucleus may move toward stability through emission of one or two protons. Proton emission (PE) is thus a competing process to β+ and α decay. Proton emission decay is much less common than are β+ and α decay and is not observed in naturally occurring radionuclides. In PE decay the atomic number Z decreases by 1 and so does the atomic mass number A. 𝐴 𝑍𝑃 → 𝐴−1 𝑍−1𝐷 +𝑝 PROTON EMISSION II When a proton is ejected from a radionuclide P, the parent nucleus P sheds an orbital electron from its outermost shell to become a neutral daughter atom. The energetic proton slows down in moving through the absorber medium and captures an electron from its surroundings to become a neutral hydrogen atom. Since the number of neutrons does not change in proton emission decay, the parent P and daughter D are isotones. PROTON EMISSION III For lighter, very proton-rich nuclides with an odd number of protons Z, proton emission decay is likely. For lighter, very proton-rich nuclides (A ≈ 50) with an even number of protons Z, a simultaneous two-proton emission may occur. An example of proton emission decay is the decay of lithium-5 into helium-4 with a half-life of 10−21 s. 5 3𝐿𝑖 → 42𝐻𝑒 + 𝑝 NEUTRON EMISSION I Neutron emission from a neutron-rich nucleus is a competing process to β− decay. It is much less common then the β− decay and is not observed in naturally occurring radionuclides. In contrast to spontaneous fission, in neutron emission decay the atomic number Z remains the same. However, the atomic mass number A decreases by 1. Both the parent nucleus P and the daughter nucleus D are thus isotopes of the same nuclear species. 𝐴 𝑍𝑋 → 𝐴−1 𝑍𝑋 +𝑛 NEUTRON EMISSION DECAY II An example of neutron emission decay is the decay of helium-5 into helium-4 with a half-life of 8×10−22 s. 5 2𝐻𝑒 → 42𝐻𝑒 + 𝑛 RADIOPHARMACEUTICALS I Functional information can be obtained by combining tomographic imaging with radiopharmaceuticals. The radioactive compound acts as the radiation source. Tomographic techniques radiopharmaceutical. are used to reconstruct the concentration Generally, two different types of radiolabels are used: gamma emitters for single photon emission computed tomography (SPECT) and positron emitters for positron emission tomography (PET). of the RADIOPHARMACEUTICALS II Examples for gamma emitters are: 81mKr (krypton) and 99mTc (technetium). Examples for positron emitters are: 18F (fluoride), 15O (oxygen), 13N (nitrogen), 11C (carbon), and 82Rb (rubidium). RADIOPHARMACEUTICALS III In some cases, the radioactive substance can be used directly. For example, 81mKr can be inhaled for immediate lung ventilation studies. More often, a specific physiologically relevant compound is labeled with a radioactive atom. One example is fluorodeoxyglucose (FDG), which is metabolized at sites of high glucose demand, i.e., brain or some tumors. The spatial distribution of FDG accurately reflects the glucose uptake in the body. By substituting the stable 9F for the positron-emitter 18F, local concentrations of glucose can be imaged with PET. RADIOPHARMACEUTICALS IV RADIOPHARMACEUTICALS V Fast radioactive decay is desirable in radiopharmaceuticals. This is because it reduces the total patient exposure. For example, 18F has a half-life of approximately 2 h. Therefore, only 1.6 % of the initially administered 18F is left after 12 h. In fact, the fraction of 18F remaining is even lower. This is because the radiopharmaceutical typically gets excreted through the urine. RADIOPHARMACEUTICALS V An ideal radiopharmaceutical needs to: have a short physical half-life, be eliminated from the body with an effective half-life approximately equal to the examination time, emit pure gamma rays with no subsequent change in the nucleus, emit mono-energetic gamma rays, have a high activity per unit mass (specific activity), be able to localise largely and quickly at the target site, RADIOPHARMACEUTICALS VI decay into a more stable daughter nucleus, easily and effectively be attached to the chemical compound at room temperature, be easily produced or found at the hospital site. The power-efficient Cyclone-30 isotope-producing cyclotron, 2.7 m in diameter and 2.8 m high. Image credit: IBA. EXERCISE FOR HOME – SBA 1 The emission of a gamma ray from a nucleus results in A) an increase in the atomic number by one. B) an increase in the atomic mass by four. C) a decrease in the atomic number by two. D) none of the above. EXERCISE FOR HOME – SBA 1 SOLUTION The emission of a gamma ray from a nucleus results in A) an increase in the atomic number by one. B) an increase in the atomic mass by four. C) a decrease in the atomic number by two. D) none of the above. EXERCISE FOR HOME – SBA 2 Which of the following isotopes is more likely to be radioactive? A) magnesium-24 B) calcium-40 C) astatine-210 D) ruthenium-101 EXERCISE FOR HOME – SBA 1 SOLUTION Which of the following isotopes is more likely to be radioactive? A) magnesium-24 B) calcium-40 C) astatine-210 D) ruthenium-101 SUMMARY I Nuclear physics has come a long way since Ernest Rutherford discovered that most of the atomic mass is concentrated in the atomic nucleus. The atomic nucleus consists of nucleons: positively charged protons and neutral neutrons. Each nuclear species is characterized with a unique combination of the number of protons and number of neutrons N. Their sum gives the number of nucleons. SUMMARY II Nucleons are bound together to form the nucleus by a strong nuclear force. To bind the nucleons into a stable nucleus a delicate equilibrium between the number of protons and the number of neutrons must exist. For light (low A) nuclear species, a stable nucleus is formed from an equal number of protons and neutrons (Z = N). Above the nucleon number A ≈ 40, more neutrons than protons must constitute the nucleus to form a stable configuration. If the optimal equilibrium between protons and neutrons does not exist, the nucleus is unstable (radioactive). SUMMARY III It decays with a specific decay constant into a more stable configuration that may also be unstable and decays further. This forms a decay chain that eventually ends with a stable nuclide. As discussed, nine main processes are available to unstable nuclei (radionuclides) to advance toward a more stable nuclear configuration. For a given radionuclide generally only one type or at most two types of decay process will occur. Nuclides with an excess number of neutrons are referred to as neutron-rich. Those with an excess of protons are referred to as proton-rich. SUMMARY IV For a slight imbalance, radionuclides will decay by β decay. This is characterized by: transformation of a proton into a neutron in β+ decay and a transformation of a neutron into a proton in β− decay. For a large imbalance, the radionuclides will decay by emission of nucleons. SUMMARY V α-particles in α decay, protons in proton emission decay, and neutrons in neutron emission decay. For very large atomic mass number nuclides(A > 230) spontaneous fission, which competes with α decay, is also possible. SUMMARY VII An ideal radiopharmaceutical needs to: have a short physical half-life, have a high activity per unit mass, be eliminated from the body with an effective half-life approximately equal to the examination time, be able to localise largely and quickly at the target site, decay into a more stable daughter nucleus, emit pure gamma rays with no subsequent change in the nucleus, easily and effectively be attached to the chemical compound at room temperature, emit mono-energetic gamma rays, be easily produced or found at the hospital site. REFERENCES Authors E.B. Podgorsak R.K.Hobbie and B.J.Roth Title Radiation Physics for Medical Physicists Intermediate Physics for Medicine and Biology Edition Publisher Year 3rd Edition Springer 2016 9783319253824 5th Edition Springer 2015 9783319126814 2nd Edition The British Institute of Radiology 2014 9780905749549 Medical Imaging Technology 1st Edition Springer 2013 9781461470724 Medical Imaging: Essentials for Physicians 1st Edition Wiley-Blackwell 2013 9780470505700 Medical Imaging and C. Martin, P. Dendy Radiation Protection for and R. Corbertt Medical Students and Clinical Staff M.A. Haidekker A.B. Wolbarst, P. Capasso and A.R. Wyant Suzanne Amador Kane Introduction to Physics in Modern Medicine 2nd Edition CRC Press ISBN 2009 9781584889434