Nuclear Medicine Physics Module PDF
Document Details
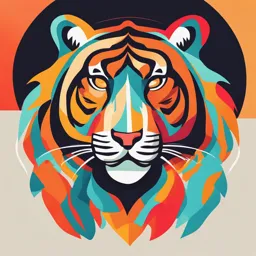
Uploaded by GloriousRhodochrosite
King Khalid University
Dr Mohammed Saeed Alqahtani
Tags
Related
- Nuclear Medicine Physics Module Lecture 1: Introduction PDF
- Nuclear Medicine Physics Module Lecture Notes PDF
- Nuclear Medicine Physics Module Lecture 1 PDF
- Nuclear Medicine Physics Module PDF
- Nuclear Medicine Physics and Techniques (IS-NUM 401) Fall 2024-2025 Lecture Notes PDF
- Types of Radiation and Radioactivity Lecture Notes PDF
Summary
This document presents lecture notes on nuclear medicine physics, focusing on the production of radionuclides and radioactive decay. It details various methods of production, and decay processes, including beta decay and positron emission. The information is useful for undergraduate studies in nuclear medicine.
Full Transcript
Nuclear Medicine Physics Module Dr Mohammed Saeed Alqahtani Lecture 2 Production of radionuclides There are more than 2700 known radionuclides. Radionuclides used in medical imaging are produced artificially, in the following ways: - - - 2 ↳ass Production of radionuclides A) If an additional neu...
Nuclear Medicine Physics Module Dr Mohammed Saeed Alqahtani Lecture 2 Production of radionuclides There are more than 2700 known radionuclides. Radionuclides used in medical imaging are produced artificially, in the following ways: - - - 2 ↳ass Production of radionuclides A) If an additional neutron is forced into a stable nucleus a neutron excess This process occurs in a nuclear reactor. For example, with Molybdenum: 98Mo [ +n 99Mo The atomic number of the nucleus remains unchanged, but its mass has been increased by one. -- 3 P - - Production of radionuclides B) If an additional proton is forced into a stable nucleus, knocking out a neutron a neutron deficit This process occurs in a cyclotron, which accelerates positively charged ions (protons, deuterons or alpha particles) on the target material. For example, -with oxygen: - 18O +p proto-atomic 18F +n TheO mass number has not been changed, but its atomic number has increased by one to become fluorine. O 4 Production of radionuclides Radionuclides produced in a cyclotron are short-lived (i.e. with half-lives ranging from less than a minute to a couple of hours). So, it is only possible to use them reasonably close to the cyclotron. Medical minicyclotrons have been designed specially for the production of short-lived radionuclides such as fluorine-18 (18F) at or near the hospital site. -- - - > - - 5 A cyclotron is like a powerful machine that accelerates charged particles, like protons or ions, to very high speeds. This Production of radionuclides If u machine is commonly used in nuclear medicine to produce radioactive isotopes for various applications, including imaging and cancer treatment. The accelerated particles collide with a target material, creating the desired radioactive isotopes that can be used in radiopharmaceuticals for diagnostic scans and therapy. : Cyclotron important 6 Asselratic charged particle like preson election / ⑳ = - / usin altemen, an magutiefeld feild Kenic e This ginengun Allo part ac gaineur n di Hil e A * Production of radionuclides C) Radioactive fission products may be extracted from the spent fuel rods of nuclear reactors - - 238U 99Mo + other fission by-products As the molybdenum =is different chemically from the other products, it can be separated and prepared in a very pure form. - - -- - - - 7 Production of radionuclides & D) Some radionuclides are daughter products obtained from generator that contain a longer-lived radioactive parent. - - - - - - Useful daughter products: Molybdenum-99 (99Mo) generator Technetium-99m (99mTc) Germanium-68 (68Ge) generator Gallium-68 (68Ga) - 8 Radioactive transformation (Decay) 9 · = B Radionuclides with a neutron excess (β- decay) * Radionuclides with a neutron excess may lose energy and become stable by a neutron changing into a proton plus an electron. * The electron is ejected from the nucleus with high energy and is referred to a negative beta particle 16 n p + βY high energy electron (Beta) 10 Radionuclides with a neutron excess (β- decay) => For example: Iodine-131 (131I) with atomic number 53, decays in this way to xenon-131 (131Xe), with atomic number 54. There has been no change of mass number, but the atomic number has increased by one. 11 Radionuclides with a neutron excess (β- decay) Isomeric transition in the case of some radionuclides, the gamma ray is not emitted until an appreciable time after the j emission of the beta particle. e For example: 99Mo decays by the emission of negative beta particle, the daughter nucleus technetium remains in the excited state for a variable length of time, which averages a matter of hours. - It is said to be metastable and is written as 99mTc a · C. 12 Radionuclides with a neutron excess (β- decay) & Parent nuclide emission (half-life) daughter nuclide S. 99mTc 3 decay to the ground state, technetium-99 (99Tc), and the decay happens with the emission of a gamma ray of energy 140 keV. Both 99mTc and 99Tc are said to be* isomer, nuclei having different energy states and halflives, but otherwise indistinguishable as regards mass number, atomic number and other properties. al TC - ↳ > - ② 99mTc half-life = 6 hours decay to 99Tc unstable 99Tc half-life = 211000 years decay to ruthenium-99 (99Ru) stable ---- 13 Radionuclides with a neutron excess (β- decay) ↑ ⑬ 14 Radionuclides with a neutron deficit: β+ decay or K-electron capture E β+ decay Radionuclides with a neutron deficit may lose energy and become stable by a proton within the nucleus changing into a neutron and a positive electron. The positive electron is ejected from the nucleus with high energy and is referred to a positive beta particle (i.e. positron) p n + β+ 15 Radionuclides with a neutron deficit: β+ decay or K-electron capture For example: Fluorine-18 (18F) with atomic number 9, transforms into oxygen-18 (18O), with atomic number 8. There has been no change of mass number, but the atomic number has decreased by one. 16 Radionuclides with a neutron deficit: β+ decay or K-electron capture K-electron capture the nucleus may increase its number of neutrons relative to the number of protons by capturing an extranuclear electron from the nearest K-shell. O 125 jists p + e- n - - = - - > - & neS - & The daughter nuclide will emit K-characteristic X-rays when an electron from an outer shell fills the created in the K-shell. proton n relat O · Kshe elector nearst by 17 Radionuclides with a neutron deficit: β+ decay or K-electron capture * For example: Iodine-123 (123I) decays wholly by electron capture and emits 160 keV gamma and 28 keV X-rays but no positive beta particles. - s - 18 Gamma rays The gamma rays emitted during radioactive decay of a given radionuclide have at most a few specific energies (forming a line spectrum) that are characteristic of the nuclide that emits them. Gamma rays have identical properties to X-ray. radi active decaye Gamma Ray emitted during spectrum) line have at most spotic energy (form o radionwide and to th carstast nuclid theat emitic 19 Gamma rays For example: Iodine-131 O (131I) emits mostly 364 keV gamma rays. 20 Positron emitters important BT 1. B Positive electrons (i.e. positrons) have a very brief existence. When a positive beta particle comes to the end of its range, it combines with a nearly negative electron. The opposite charges neutralise each other. 15 The combined masses of the two electrons are wholly converted into energy. - · s · - 1 ele 21 - Positron emitters According to Einstein's formula (E = m c2 ) for the equivalence between energy E and mass m, the mass of each electron is equivalent to 511 keV. When the positive and negative electrons annihilate each other, the energy is emitted as two photons of annihilation radiation (each of 511 keV) travelling in opposite directions. x Positron emitters are used in positron emission tomography (PET) imaging. -- S I - o 5jl - 22 Positron emitters f K-1 Atomic number 23 Radioactive decay - Radioactive disintegration is a stochastic (random) process. 0 It is impossible to predict which of the unstable nuclei in a sample will disintegrate in the next second. ~ , J BUT it is possible to be quite precise about the fraction that will disintegrate on account of the large numbers of nuclei the sample contains. S 24 Activity& & 8 A radioactive nucleus does not make its presence known until it decays and emits a beta or gamma rays, or both. - - ② The quantity of radioactivity is measured not by the ‘population’, the mass or number of radioactive atoms, BUT by the transformation rate, i.e. the number that represents disintegrate per second (also known as the decay rate). = 25 Activity what is the activity or · The activity of radioactive sample is the rate of disintegration, the amount of disintegrating per second. The SI unit is the Becquerel (Bq) = 1 disintegration per second. In medical gamma imaging, most radionuclide administrations are measured in megabecquerels (1 MBq = 106 Bq). The activity of radionuclide generators in gigabecquerels (1 GBq = 109 Bq). A unit found in old textbooks is the curie (Ci) 1 mCi = 37MBq = 26 Activity S T When the gamma rays enter a detector, they may be registered individually as counts. The count rate [number of counts per second (cps)] measured by a given instrument is less than the activity, because the greater proportion of the rays usually miss the detector and some pass through it undetected. However, there is a proportionality: 5 Count rate α activity α number of radioactive atoms in the sample - N - 27 Activity Es The fundamental law of radioactive decay states that the activity of a radioactive sample decreases by equal fractions (percentage) in equal interval time. => & jo S - I This is referred to as the exponential law. - However long the time, the activity of a radioactive sample never falls to zero. 28 Physical half-life what is the different between physical & half-life Biological half-lif ? The half-life (t1/2) of a radionuclide is the time taken for its activity to decay to half of its original value. - - 10 For example: 5 * Two successive half-lives reduce the activity of a S 22 radionuclide by a factor of 2 X 2 = 4. * Ten half-lives reduce the activity by a factor of 210 ≈ 1000 -- = ⑳ 2029 Physical half-life -- S Radioactive decay reduces the number of radioactive nuclei over time. - - In one half-life (t1/2), the number decreases to half of its original value. Half of what remains decay in the next halflife, and half of those in the next, and so on. > - This is an exponential decay, as seen in the graph of the number of nuclei present as a function of time. 30 < Physical half-life This half-life is more properly called the physical half-life. X It is a fixed characteristic of the radionuclide, cannot be predicted for a given radionuclide in any way. The physical half-life is unaffected by any other factors, such as heat, pressure, electricity or chemical reactions. It can range from fractions of a second (useless in imaging) to millennia in the case of 99Tc (also useless in imaging). - - - - - 31 Physical half-life - Physical half-lives of some radionuclide used in medical imaging: Half-life Radionuclide Half-life Radionuclide 13 s Krypton-81m 67 h < 1 min Rubidium-82 67 h < 10 min Nitrogen-13 73 h Thallium-201 20 min Carbon-11 78 h Gallium-67 68 min Gallium-68 5 days Xenon-133 110 min Fluorine-18 8 days Iodine-131 6h Technetium-99m 211 000 years Technetium-99 13 h Iodine-123 Molybdenum-99 Indium-111 32 Effective half-life When a radionuclide is used in medical gamma imaging, it usually forms part of a salt or organic compound, the S metabolic properties&of which in - it concentrates - ensure that & & the tissues or organ of interest. - *& - ↑ - jeg A pharmaceutical that has been labelled with a radionuclide is referred to as a radiopharmaceutical. I & j1 ~ ~ M J -s S 33 Effective half-life If the pharmaceutical alone is administered, it is gradually eliminated from the tissue, organ and whole body by the usual metabolic processes of turnover and excretion. jig j-193 & Such a process can be regarded as having a biological half-life · (tbiol). 1 , - If the radionuclide is stored in a bottle, its activity decays with its physical half-life (tphys). - > - - 34 Effective half-life or If the radiopharmaceutical is administered to a patient, the radioactivity in specific tissue, an organ or the whole body decreases because of the simultaneous effects of radioactive decay and metabolic turnover and excretion.. 's The radiopharmaceutical can be regarded as having an , effective half-life (teff). 35 Effective half-life The effective half-life is shorter than either the biological or => physical half-lives. - - teff < tbiol , tphys 1/teff = 1/tbiol + 1/tphys The effective half-life depends on the radiopharmaceutical used and the organ involved, and it can vary from person to person, depending on their disease state. - - - - - 36 Radiopharmaceuticals C 37 Radiopharmaceuticals 9355s 5 Desirable properties of a radionuclide for imaging are as meta follow: the Technasiu qq Best why ? 1. E A physical half-life of aL few hours,I similar to the time from preparation to injection. If the half-life is too short, much more activity must be prepared than the actually injected. 2. Decay to a stable daughter or at least one with a very long half-life (e.g. 99Tc has half-life of about 211000 years). 38 Radiopharmaceuticals Desirable properties of a radionuclide for imaging are as follow: 3. Emission of gamma rays (which produce the image), but not alpha or beta particles nor very low energy photons, which have a short range in tissue and deposit unnecessary dose in the patient. ↳ why 2-particle & Beta particle are not used in imaging ? 4. Emission of gamma rays of energy 50-300 keV and ideally about 150 keV – high enough to exit the patient but low enough to be Spatial reslution (SR) easy collimated and easily detected. --- - · counts ↳ --. Full width maxie Distance 39 " are m a x i n a t wi d t h < Radiopharmaceuticals , Fill e Desirable properties of a radionuclide for imaging are as follow: 5. Ideally, emission of monoenergetic gamma rays, so that scattered radiation can be eliminated by energy ing e discrimination with a pulse height analyser (PHA). Enerving Air 6. Easily and firmly attached to the pharmaceutical at room O temperature, but has no affect on its metabolism. 40 Radiopharmaceuticals Desirable properties of a radionuclide for imaging are as follow: 7. Readily available at the hospital site. 8. A high specific activity, i.e. high activity per unit volume. 41 Radiopharmaceuticals -- Desirable properties of a radiopharmaceutical are as follow: 1. localise largely and quickly in the target (i.e. O the tissue of interest) 2. be eliminated from the body with a effective half-life similar to the duration of the examination, to reduce the dose to the patient 3. have a low toxicity 42 Radiopharmaceuticals - J J & Desirable properties of a radiopharmaceutical are as follow: 4. form a stable product both in vitro and in vivo 5. be readily available and inexpensive per patient dose The decay during transport and storage of a short-lived radionuclide is reduced if it can be supplied with its longerlived parent in a generator. 43 Technetium generator Technetium-99m (99mTc) is used in 90% of radionuclide imaging, as it fulfils most of the mentioned criteria. With its gamma energy of 141 keV, it is easily collimated and easily absorbed in a fairly thin crystal, thus giving good spatial resolution. With its short half-life (6 h) and pure gamma emission, a reasonably large activity can be administered, reducing noise in the image. 44 Technetium generator important How To generator works ? Technetium-99m (99mTc) is supplied from a generator shielded with lead. The generator contains an exchange column of alumina beads on which have been absorbed a compound of the parent 99Mo (which can be produced in a nuclear reactor and has a 67 h half-life). 45 Technetium generator Schematic of Technetium-99m generator 46 Technetium generator When the generator is delivered, the activity of the daughter 99mTc has built up to its maximum, equal to that of the parent 99Mo. The daughter is decaying as quickly as it is being formed by the decay of its parent. The daughter and the parent decay together with the halflife of the parent (67 h). S 18 47 portant Technetium generator im I Decay of activity of molybdenum-99 and growth and regrowth of activity of technetium-99m in a generator that is eluted daily. x o + el + 48 Technetium generator The technetium-99m is washed off the column (eluted) as sodium pertechnetate with sterile saline solution. This flows under pressure from a reservoir through the column into a rubber-capped sterile container. The precise design of the generator and pressure system depends on the manufacturer. Elution takes a few minutes and leaves behind the molybdenum, which is firmly attached to the column. 49 Technetium generator o - As you have seen in the previous figure, the 99mTc in the column increases with the same half-life of 6 h. After 24 h, the activity has grown again to a new maximum (equilibrium) value. Elution can be made daily, although it will be seen that the strength of successive eluents diminishes in line with the decay of 99Mo. After a week, the generator is usually replaced and the old one is returned for recycling. - -- - 50 Uses of technetium-99m - >1/5 ↑ T P Sodium pertechnetate- 99mTc is used for imaging the tissues: the thyroid, the gastric mucosa (localisation of Meckel’s diverticulum) and the salivary glands. Sis - Blocked from the thyroid by administration of potassium perchlorate, it can be used for cerebral blood flow and testicular imaging and mixed with bran porridge for gastricemptying studies. 51 Uses of technetium-99m 99mTc can easily be labelled to a wide variety of usual compounds, for example : methylene diphosphonate (MDP): for bone imaging 3061 hexamethyl propylene amine oxime (HMPAO): for cerebral > imaging dimercaptosuccinic acid (DMSA) and mercapto* acetyltriglycine (MAG3): for renal studies iminodiacetic acid (HIDA): for biliary studies (dH -- - - - - - - - - >B - - - 52 Uses of technetium-99m - human serum albumin (HSA): imaging of liver, spleen and > red bone marrow diethylene triamine pentacetic acid (DTPA) aerosol (5µm particles): for lung ventilation studies autologous red cells: for cardiac function 8 heat-damaged autologous red cells: for imaging the spleen sestamibi or tetrofosmin: for cardiac perfusion imaging - - - ---- - -= > -- - -- 53 other radionuclides and their uses - Iodine is trapped and metabolised by the thyroid, which was the first organ ever imaged,E and 131I was the first radionuclide used for imaging. 131I was cheap, highly reactive and an excellent label. 131I is> produced in a nuclear reactor and has a long half-life (8 days), but emits beta particles as well as energetic gamma rays (mainly364 keV). - - -- - - - - - - 54 other radionuclides and their uses ↑ 131I has largely been replaced for imaging by 123I, which is -more expensive, cyclotron produced, has a half-life of 13 h, -and decays by electron capture emitting 159 keV gamma rays. - - 123I is also more expensive than, but otherwise superior to, 125I with its long half-life (60 days) and low photon energy (35 keV) & Note that both 131I and 125I are used for therapy: - 131I is used for thyroid ablation - 125I is used as brachytherapy seeds --- -- - -- -- - --- - -- 55 other radionuclides and their uses - Xenon-133 (133Xe): is produced in a nuclear reactor, has a half-life of 5.2 days and emits beta particles and low energy ---gamma rays (81 keV) - - - - & It is an inert gas, although somewhat soluble in blood and fat, and is used, with rebreathing, in lung ventilation imaging. - - - - - - - -- - 56 other radionuclides and their uses - Krypton-81m (81mKr): another inert gas, is generatorproduced, has a half-life of7 13 s and emits190 keV gamma rays. The generator is eluted with compressed air, and the patient 81mKr mixture) in pulmonary ventilation inhaling the air (i.e. - - studies. The parent,=> Rubidium-81 (81Rb), has a short half-life (4.7 h), which presents transport difficulties and means that it must be used the day it is delivered. - - - - - - - - ↑ 57 other radionuclides and their uses Gallium-67 (67Ga): is cyclotron-produced, has a half-life of 67 h, and decays by electron capture, emitting gamma rays of S three main energies (93, 184, 296 and 388 keV). C - - - - & - - = - - - - = = E Gallium citrate is used to detect tumours and abscesses. - es 58 other radionuclides and their uses Indium-111 (111In): is cyclotron-produced, has a half-life of 67 h, and decays by electron capture, emitting 171.3 keV and & 245.4 keV gamma rays. -- - - - - - - -- - - -- - It is used to label white blood cells and platelets for locating abscesses and thrombosis. --- - - - 59 other radionuclides and their uses 111In, is Indium-113 (113mIn): is sometimes used instead ofO generator-produced, has a↑half-life of 100 min, and emits ---only gamma rays, but they have a high energy (390 keV). ---- - - - & 60 S. Positron emitters · Positron emitters are needed for PET (positron emission tomography) scans. The most common PET radionuclide is 18F, with a half-life of 110 min. 18F often used in the form of 18F 2-fluroro-2deoxyglucose (2FDG) for brain and heart metabolism as well as eplipsy and ) tumour detection.. - > - 61 Positron emitters Other radionuclides used for PET are carbon-11 (11C), nitrogen-13 (13N), oxygen-15 (15O) and rubidium-82 (82Rb), but these positron emitters have very short half-lives: 20 min, 10 min, 2 min and 75 s, respectively. -- - -- -- 82Rb, which is produced from a strontium-82 generator that lasts about 1 month, can be used for myocardial prefusion imaging. 62 Positron emitters Technetium-94m (94mTc), cyclotron-produced with a half-life of 50 min. It can be used to label many pharmaceuticals already available for 99mTc imaging. 94mTc sestamibi can be used in tumour imaging studies utilising PET. - - & 63 64