Lecture 7 neuromuscular plasticity and disuse-training.pdf
Document Details
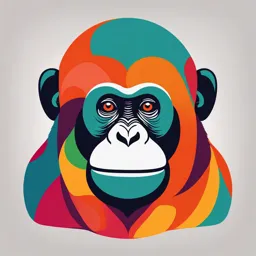
Uploaded by Curtis
York University
Tags
Full Transcript
Neuromuscular plasticity: disuse and exercise Michael Paris School of Kinesiology and Health Science York University, Toronto, ON Learning objectives Describe the impairments in skeletal muscle tissue that occur with disuse, with reference to specific fibre type and contractility measures Explain th...
Neuromuscular plasticity: disuse and exercise Michael Paris School of Kinesiology and Health Science York University, Toronto, ON Learning objectives Describe the impairments in skeletal muscle tissue that occur with disuse, with reference to specific fibre type and contractility measures Explain the neural and skeletal muscle adaptations to exercise loading and their time-course Convey the neural sites for loading-induced increases in strength, with reference to typespecificity of training Models of disuse Accelerated loss of skeletal muscle mass (and function) with disuse and illness Contrast with age-related loss of ~0.5 to 1% / year! Atrophy occurs in all fibre-types Muscle fibre atrophy is consistent across all fibre-types in humans Likely dependent upon the model of disuse AND muscle group! Example of vastus lateralis, but what about non-lower limb muscles? Complete spinal cord injury – major fibre type transitions No visible slow fibres All fast fibres Near complete fibre-type transition from fast to slow phenotype Extreme form of disuse – less clear in other forms of disuse Aging vs disuse vs disease Fatigability is worsened with disuse Muscle structure and function during disuse and disease Brief summary of disuse on muscle structure and function Property Involvement Muscle atrophy Greatest in lower limbs, antigravity muscles Least in upper limbs (e.g., hand muscles) Fibre type atrophy Occurs in both type I and II fibres Twitch force Unclear – may remain normal or decrease Tetanic force reduced Voluntary force VERY reduced Fatigability increased Exacerbated in disease states? Does the disease CAUSE the impairment? Or does it limit activity/exercise, leading to disuse? Combination? How do we attenuate these declines in muscle structure and function Timeline of central and peripheral adaptations to strength training Early phase of adaptations (2-4 weeks) Late phase of adaptations (months, years) How do muscles grow in response to loading? Is each fibre is bigger? More muscle fibres? Myofibre hypertrophy Myofibre hyperplasia myofibre loading loading Fascicle But what is actually ‘growing’? Answer - Myofibrils! However, it is unresolved if myofibrils increase in size or number (note – myofibril is not a myofibre) Muscle growth occurs when muscle protein synthesis > muscle protein breakdown Muscle protein in constant flux Synthesis and breakdown of myofibrils A) no exercise, regular protein consumption Synthesis=breakdown B) exercise + protein Stimulate synthesis above breakdown Net accretion of contractile tissue Fibre-type specific hypertrophy 40 1 RM (%) 95 40 1 RM (%) What about the influence of load/intensity of training? Most work has focused on ‘heavy’ (>80% 1 RM) resistance exercise. What about low loads? 95 Low-load vs high-load REMEMBER SUB-MAXIMAL FATIGUE! Progressive MU recruitment and rate coding until range of motion failure – all MUs were fatigued Loading induced hypertrophy Muscle growth in adults from to loading is due to muscle fibre hypertrophy, not hyperplasia Unclear how myofibrils within muscle fibre are growing to cause muscle fibre hypertrophy Growth occurs when synthesis>breakdown – need loading + protein Fibre-type specific adaptations? Evidence points to Type II hypertrophy but likely training-dependent Low-load vs. high-load training to failure Fully activated (and fatigued??) all motor unit Similar training responses? Perhaps for hypertrophy, but certainly not strength (task specificity) Short-term improvements in muscle strength Task-specificity of training is important! More on this later…. What can account for this rapid increase in muscle strength? What is adaptating? Very incomplete understanding of neural adaptations to loading 3 key factors Agonist activation Coordination amongst synergist muscles Antagonist activation Agonist activation Isometric knee extensor training Early increase in isometric strength aligned well with increased EMG activation of the quadriceps muscles Most common observation, increased agonist EMG What is responsible for increased agonist EMG? Motor unit firing rates increase with loading Greater voluntary activation (interpolated twitch) Decreased antagonist activation – an early adaptation?? Antagnoist activation will limit the force expression of the agonist muscle, but also is necessary for joint stabilization Not clear what CNS optimizes, force or stability BUT, some evidence of decreased antagonist early in the training adaptations Decreased antagonist activation occurred in both the trained and non-trained legs…. Indicating a central adaptation Where are these adaptations occurring? Task-specificity of training Significant improvements in isotonic weight training But minimal improvements in isometric maximal voluntary force Training is highly specific to type of loading Explosive vs slow controlled? Δ~21% Δ~4% Δ~28% Δ~1% Δ~11% Δ~53% Δ~12.5% Δ~42% Rapid training – increase in motor unit firing rate during ‘explosive’ contraction Take home points Both central and peripheral adaptations are responsible for improvement in muscle strength timeline of adaptations different Neural sites of adaptation not clear Difficult to study! Many neural adaptations are specific to training paradigm Often train using sub-maximal efforts but test using maximal efforts – incongruency