Lecture 7 BIO3147 (2024) PDF
Document Details
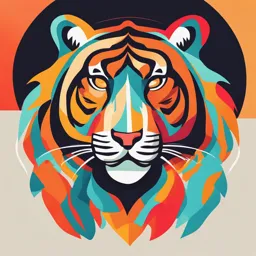
Uploaded by SafeMahoganyObsidian
null
2024
Tags
Summary
This lecture covers various aspects of cell communication and development. It details different cell signaling mechanisms and their roles in embryonic development.
Full Transcript
Figure 4.1 Local and long-range modes of cell-to-cell communication (A) Juxtracrine signaling: Local cell signaling is carried out via membrane receptors that bind to proteins in the extracellular matrix (ECM) or directly to receptors from a neighboring cell. (B) Paracrine signaling: Signaling acros...
Figure 4.1 Local and long-range modes of cell-to-cell communication (A) Juxtracrine signaling: Local cell signaling is carried out via membrane receptors that bind to proteins in the extracellular matrix (ECM) or directly to receptors from a neighboring cell. (B) Paracrine signaling: Signaling across multiple cell distances, whereby one cell secretes a signaling protein (ligand) into the environment and across the distance of many cells. Only those cells expressing this ligand’s corresponding receptor can respond, either rapidly through chemical reactions in the cytosol, or more slowly through the process of gene and protein expression. (C) Endocrine Signaling: Long range glandular/interorgan signaling of hormones (D) Autocrine Signaling: A cell signaling to itself. Figure 4.3 Reaggregation of cells from amphibian neurulae Presumptive epidermal cells from pigmented embryos and neural plate cells from unpigmented embryos were dissociated and mixed together. The cells reaggregated so that one type (the presumptive epidermis) covered the other. Figure 4.4 Sorting out and reconstruction of spatial relationships in aggregates of embryonic amphibian cells Figure 4.5 Hierarchy of cell sorting of decreasing surface tensions (A) Simple schematic demonstrating a logic statement for the properties of differential cell adhesion. (B) The equilibrium configuration reflects the strength of cell cohesion, with the cell types having the greater cell cohesion segregating inside the cells with less cohesion. Figure 4.6 Simplified scheme of cadherin linkage to the cytoskeleton via catenins Figure 4.7 E-cadherin is required for epiboly in zebrafish (A) Wild-type embryos (right), and embryos heterozygous (center) and homozygous (left) for the Ecadherin mutation. During normal gastrulation, cells merge into a thinner but more expansive epiblast layer that envelops the entire yolk (the red arrowhead points to the location of final yolk enclosure in the wildtype). E-cadherin mutants fail to complete epiboly, which is most severely impaired in the homozygous mutant (red lines denote the leading edge of epiblast). Figure 4.7 E-cadherin is required for epiboly in zebrafish (B) Schematic of radial intercalating cell movements in the zebrafish epiblast over time during gastrulation. EVL, enveloping layer; HB, hypoblast; YSL, yolk syncytial layer. Cells move toward the superficial enveloping layer in relationship to increasing expression of E-cadherin. Ecadherin is expressed at higher levels in the more superficial layers of the epiblast, including the enveloping layer, and it is this differential expression (and consequently differential adhesion) that powers the radial movement of deep cells to the periphery. Figure 4.8 Importance of the amount of cadherin for correct morphogenesis (12th edition) Aggregate surface tension correlates with the number of cadherin molecules on the cell membranes. Sorting can occur based on cadherin number even if the two cells express different cadherin proteins Figure 4.9 Extracellular matrices in the developing embryo (A) Fluorescent antibodies to fibronectin show fibronectin deposition as a green band in the Xenopus embryo during gastrulation. The fibronectin will orient the movements of the mesodermal cells. Figure 4.10 Simplified diagram of the fibronectin receptor complex RGD, arginine-glycine-aspartate Integrins function as transmembrane linkers (or “integrators”), mediating the interactions between the cytoskeleton and the extracellular matrix that are required for cells to grip the matrix. The integrins of the complex can form heterodimerized membrane-spanning receptor proteins that bind fibronectin on the outside of the cell while interacting with actin cytoskeletonassociated proteins such as α-Actinin, Vinculin, and Talin on the inside of the cell. Figure 4.11 Epithelial-mesenchymal transition, or EMT (A) Normal epithelial cells are attached to one another through adherens junctions containing cadherin, catenins, and actin rings. They are attached to the basal lamina (extracellular matrix) through integrins. Paracrine factors can repress the expression of genes that encode these cellular components, causing the cell to lose polarity, lose attachment to the basal lamina, and lose cohesion with other epithelial cells. Cytoskeletal remodeling occurs, as well as the secretion of proteases that degrade the basal lamina and other extracellular matrix components of the basement membrane, enabling the migration of the newly formed mesenchymal cell. Figure 4.11 Epithelial-mesenchymal transition, or EMT (B,C) EMT is seen in vertebrate embryos during the normal formation of neural crest from the dorsal region of the neural tube (B) and during the formation of the mesoderm by mesenchymal cells delaminating from the epiblast (C). Figure 4.12 Ectodermal competence and the ability to respond to the optic vesicle inducer in the frog Xenopus The optic vesicle is able to induce lens formation in the anterior portion of the ectoderm (1) but not in the presumptive trunk and abdomen (2). (3) If the optic vesicle is removed, the surface ectoderm forms either an abnormal lens or no lens at all. (4) Most other tissues are not able to substitute for the optic vesicle. Figure 4.13 Sequence of amphibian lens induction postulated by experiments on embryos of the frog Xenopus laevis Unidentified inducers (possibly from the foregut endoderm and cardiac mesoderm) cause the synthesis of the Otx2 transcription factor in the head ectoderm during the late gastrula stage. As the neural folds rise, inducers from the anterior neural plate (including the region that will form the retina) induce Pax6 expression in the anterior ectoderm that can form lens tissue. Expression of Pax6 protein may constitute the competence of the surface ectoderm to respond to the optic vesicle during the late neurula stage. The optic vesicle secretes BMP and FGF family paracrine factors (see signals in higher magnification of boxed area) that induce the synthesis of the Sox transcription factors and initiate observable lens formation. Figure 4.14 The Pax6 gene is similarly required for eye development in frogs, rats, and humans (12th edition) (A) Loss of Pax6 in rats results in the failure to form eyes as well as significant reductions in nasal structures. (B) An analysis of lens induction following recombination experiments of the optic vesicle and surface ectoderm between wild-type and Pax6 null rat embryos. Pax6 is required only in the surface ectoderm for proper lens induction. (C) Mutations in the Pax6 gene in Xenopus and humans result in similar reductions in the iris of the eye as compared with wild-type individuals. This phenotype is characteristic of aniridia. Figure 4.16 Specification of uniform cells into three cell types by a morphogen gradient (12th edition) Morphogen (red dots): signaling molecules (proteins or otherwise) that act over long distances (paracrine) to induce responses in cells based on the concentration of morphogen that the cells interact with. Cells exposed to morphogen concentrations above threshold 1 (red) activate certain genes. Cells exposed to intermediate concentrations (pink) activate a different set of genes and also inhibit the genes induced at the higher concentrations. Those cells encountering low concentrations of morphogen (below threshold 2; blue) activate a third set of genes Figure 4.17 A gradient of the paracrine factor Activin, a morphogen, causes concentrationdependent expression differences of two genes in unspecified amphibian cells (A) Beads containing no Activin did not elicit expression (i.e., mRNA transcription) of either the Xbra or goosecoid gene. (B) Beads containing 1 nM Activin elicited Xbra expression in nearby cells. (C) Beads containing 4 nM Activin elicited Xbra expression, but only at a distance of several cell diameters from the beads. A region of goosecoid expression is seen near the source bead, however. Thus, it appears that Xbra is induced at particular concentrations of Activin and that goosecoid is induced at higher concentrations. Figure 4.17 A gradient of the paracrine factor Activin, a morphogen, causes concentrationdependent expression differences of two genes in unspecified amphibian cells (D) Interpretation of the Xenopus Activin gradient. High concentrations of Activin activate goosecoid, whereas lower concentrations activate Xbra. A threshold value appears to exist that determines whether a cell will express goosecoid, Xbra, or neither gene. In addition, Xbra inhibits the expression of goosecoid, thereby creating a distinct boundary. This pattern correlates with the number of Activin receptors occupied on individual cells. Figure 4.18 Structure and function of a receptor tyrosine kinase The binding of a paracrine factor (such as Fgf8) by the extracellular portion of the receptor protein activates the dormant tyrosine kinase, followed by phosphorylation of specific tyrosine residues of certain intracellular proteins. Figure 4.19 Fgf8 in the developing chick (Part 1) Fgf8 protein (dark areas) is seen in the distal-most limb bud ectoderm (1), in the somitic mesoderm (the segmented blocks of cells along the anteriorposterior axis; 2), in the pharyngeal arches of the neck (3), at the boundary between the midbrain and hindbrain (4), in the optic vesicle of the developing eye (5), and in the tail (6). Figure 4.20 The widely used RTK signal transduction pathway can be activated by fibroblast growth factor The receptor tyrosine kinase is dimerized by the ligand (a paracrine factor, such as FGF) along with heparan sulfate proteoglycans (HSPG), which together cause the dimerization and autophosphorylation of the RTKs. Figure 4.20 The widely used RTK signal transduction pathway can be activated by fibroblast growth factor [The adaptor protein recognizes the phosphorylated tyrosines on the RTK and activates an intermediate protein, GEF, which activates the Ras G protein by allowing phosphorylation of the GDP-bound Ras. At the same time, the GAP protein stimulates hydrolysis of this phosphate bond, returning Ras to its inactive state. The active Ras activates the Raf protein kinase C, which in turn phosphorylates a series of kinases (such as MEK)] Eventually, the activated kinase ERK alters gene expression in the nucleus of the responding cell by phosphorylating certain transcription factors (which can then enter the nucleus to change the types of genes transcribed) and certain translation factors (which alter the level of protein synthesis). Figure 4.21 A JAK-STAT pathway: casein gene activation The gene for casein is activated during the final (lactogenic) phase of mammary gland development, and its activating signal is the secretion of the hormone prolactin from the anterior pituitary gland. Prolactin causes the dimerization of prolactin receptors in the mammary duct epithelial cells. A particular JAK protein (Jak2) is “hitched” to the cytoplasmic domain of these receptors. When the receptors bind prolactin and dimerize, the JAK proteins phosphorylate each other and the dimerized receptors, activating the dormant kinase activity of the receptors. Figure 4.21 A JAK-STAT pathway: casein gene activation The activated receptors add a phosphate group to a tyrosine residue (Y) of a particular STAT protein, which in this case is Stat5. This addition allows Stat5 to dimerize, be translocated into the nucleus, and bind to particular regions of DNA. GR is the glucocorticoid receptor, OCT1 is a general transcription factor, and TBP is the major promoterbinding protein that anchors RNA polymerase II and is responsible for binding RNA polymerase II In combination with other transcription factors, the Stat5 protein activates transcription of the casein gene. Figure 4.22 A mutation in the gene for FgfR3 causes the premature constitutive activation of the STAT pathway and the production of phosphorylated Stat1 protein Stat1 (transcription factor) activates genes that cause the premature termination of chondrocyte cell division in the growth plates. The result is thanatophoric dysplasia, a condition of failed bone growth that results in the death of the newborn infant because the thoracic cage cannot expand to allow breathing. Figure 4.23 Hedgehog processing and secretion Cleavage and lipid-modifications are essential for hedgehog secretion Membrane-associated Glycoproteins (Heparan Sulfate Proteoglycan; HSPG) can enable complex assembly and transport Figure 4.25 Sonic Hedgehog Head of a cyclopic lamb born of a ewe that ate Veratrum californicum early in pregnancy, caused by ingestion of the alkaloid cyclopamine. In some parts of Idaho, up to 25% of newborn lambs were affected at some ranches during the end of Summer Figure 4.25 Sonic Hedgehog Cell, Vol. 75, 1401-1416, December 31, 1993, Copyright 0 1993 by Cell Press Sonic hedgehog Mediates the Polarizing Activity of the ZPA Robert D. Riddle, Randy L. Johnson, and Cliff Tabin Department of Genetics Harvard Medical School Boston, Massachusetts 02115 Ed Laufer, Summary (A) Sonic hedgehog is shown by in situ hybridization to be expressed in the nervous system (red arrow), gut (blue arrow), and limb bud (black arrow) of a 3-day chick embryo The zone of polarizing activity (ZPA) is a region at the posterior margin of the limb bud that induces mirrorimage duplications when grafted to the anterior of a second limb. We have isolated a vertebrate gene, Sonic hedgehog, related to the Drosophila segment polarity gene hedgehog, which is expressed specifically in the ZPA and in other regions of the embryo, that is capable of polarizing limbs in grafting experiments. Retinoic acid, which can convert anterior limb bud tissue into tissue with polarizing activity, concomitantly induces Sonic hedgehog expression in the anterior limb bud. Implanting cells that express Sonic hedgehog into anterior limb buds is sufficient to cause ZPA-like limb duplications. Like the ZPA, Sonic hedgehog expres- acid is unlikely for ZPA activity ( One of the strong date ZPA morpho retinoic acid, at a tions, induces a gene (the retinoi than that normal 1991). This impli than is required retinoic acid is p lieved that rathe signal, retinoic ZPA. The anterio implant and direc strated to acqui that tissue to an 1983; Wanek e to a ZPA graft d Exogenous retin stream of the ZP One approach fying new signali Figure 4.25 Sonic Hedgehog Sonic hedgehog mutations induce cyclopia (Nature; 1996) Ov=optic vesicle; op= olfactory placode Figure 4.25 Sonic Hedgehog Alkaloids (Cyclopamine/Jervine) from the Veratrum plant inhibits Shh signaling! (Science; 1998) Opt=optic vesicle; olf= olfactory placode; Mx=maxillary; Mn=mandiubluar Figure 4.26 Wnt4 is necessary for kidney development and for female sex determination Wnt4 is necessary for kidney development and for female sex determination. (A) Urogenital rudiment of a wild-type newborn female mouse. (B) Urogenital rudiment of a newborn female mouse with targeted knockout of Wnt4. Kidney fails to develop. In addition, the ovary starts synthesizing testosterone and becomes surrounded by a modified male duct system. Figure 4.27 Notum antagonism of Wnt Lipidation and HSPG (glypican) is also essential for Wnt signaling. Notum creates a negative feedback mechanism for Wnt signaling. Figure 4.28 Wnt signal transduction pathways *Phosphorylation & Ubiquitination are critical for the regulation of Wnt signaling Figure 4.29 Relationships among members of the TGF-β superfamily Figure 4.30 The Smad pathway is activated by TGF-β superfamily ligands Different TGF-beta ligands lead to phosphorylation of different Smads to regulate transcription Figure 4.33 Wnt diffusion is affected by other proteins (12th edition) (A) Diffusion of Wingless (Wg, a Wnt paracrine factor) throughout the developing wing of wildtype Drosophila (above) is enhanced by Swim, a protein that stabilizes Wg and that is made by some of the wing cells. When Swim is not present, as in the mutant below, Wg does not disperse and is confined to the narrow band of Wgexpressing cells. (B) Similarly, Wingless usually activates the Distal-less gene (green) in much of the wild-type wing (above). However, in swim-mutant flies (below), the range of Distal-less expression is confined to those areas near the band of Wg-expressing cells.