Lecture 6 Visual, Auditory & Sensory System PDF
Document Details
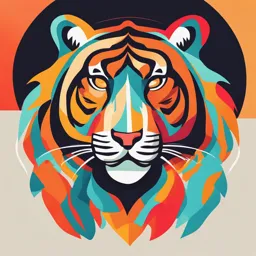
Uploaded by MultiPurposeTrust610
HELP University
Tags
Summary
This document covers different aspects of the visual system, including the anatomy of the eye, photoreceptor cells (rods and cones), and different theories of color vision. It describes the visual pathway, explaining how visual information is transmitted from photoreceptors to the brain.
Full Transcript
Biological Psychology Thirteenth Edition Chapter 5 Vision © 2019 Cengage. All rights reserved. Introduction Sensation: stimuli detection in environment Perception: brain interpretation of sensory input Light Passes through the f...
Biological Psychology Thirteenth Edition Chapter 5 Vision © 2019 Cengage. All rights reserved. Introduction Sensation: stimuli detection in environment Perception: brain interpretation of sensory input Light Passes through the following Cornea - transparent outer layer (continuation of the Sclara) Aqueous humor - fluid produced and reabsorbed between lens and cornea Iris diaphram - pigmented muscle which can change the size of the opening pupil Lens - transparent structure that serves to bend light rays to focus them on the receptors vitreous humor - jelly like structure between lens and retina retina - interior lining at back of eye which contains the receptor units (contains rods & cones, bipolar cells, ganglion cells, amacrine and horizontal cells) NOTE: The function of the eye is to direct the physical energy for vision to the receptors in the retina and focus light Ocular Anatomy En route to the retina, light successively travels through: 1) the cornea 2) the aqueous humor of the anterior chamber 3) the pupil 4) the lens 5) the vitreous humor Retina Light strikes photoreceptors only after passing through sensory neurons, except at the central retinal region (fovea) where acuity is best. The Fovea Is the central portion of the retina and allows for acute and detailed vision – Packed tightly with receptors – Nearly free of ganglion axons and blood vessels Each receptor (almost exclusively cones) in the fovea attaches to a single bipolar cell and a single ganglion cell. Each cone in the fovea has a direct line to the brain which allows the registering of the exact location of input Our vision is dominated by what we see in the fovea © 2019 Cengage. All rights reserved. RETINA Light passes through the pupil and is focused by the lens onto the retina at the back of the eye The retina consists of three layers of cells Ganglion cell layer Bipolar layer Photoreceptor layer: receptors in this layer transduce light The ganglion cell layer is the outermost layer and the photoreceptor layer is the innermost layer In order to reach the photoreceptor layer, light actually passes through the outer two layers of the retina Photoreceptor Cells > Bipolar Retina Cell Arrangement Cells > Ganglion Cells Photoreceptors: Rods and cones, sensitive to light Bipolar Cell: Transfer signal from photoreceptor cells Ganglion Cell: Axons join to form the optic nerve that exits through the back of the eye Horizontal cell Regulates the signal that emerges from several rods and cones. Amacrine cell Reaches across several bipolar cells to regulate signals directed at retinal ganglion cells. Control the ability of the ganglion cells to respond to shapes, movements, or other specific aspects of visual stimuli Visual Receptors: Rods and Cones (1 of 2) The vertebrate retina consists of two kinds of receptors – Rods: most abundant in the periphery of the eye and respond to faint light (120 million per retina) – Cones: most abundant in and around the fovea (6 million per retina) Essential for color vision and more useful in bright light © 2019 Cengage. All rights reserved. Photoreceptors Rods Sensitivity: Rods are highly sensitive to light, making them ideal for vision in low-light conditions. Structure: contain a large amount of a pigment called rhodopsin. Function: Rods are primarily responsible for scotopic vision, which is vision in dim light. They do not provide color vision, but they are very sensitive to motion. Cones Sensitivity: Cones are less sensitive to light than rods, but they are essential for color vision and vision in bright conditions. Structure: Cones are shorter and thicker than rods and contain different pigments that are sensitive to different wavelengths of light. Function: There are three types of cones: red, green, and blue. Each type of cone is most sensitive to a specific wavelength of light, allowing us to perceive different colors. Feature Rods Cones Sensitivity High Low Color vision No Yes Structure Long, cylindrical Shorter, thicker Pigment Rhodopsin Different pigments for red, green, and blue Function Scotopic vision Photopic vision Retina Visual information is transmitted from photoreceptors to bipolar neurons and ganglion neurons before exiting the eye via the optic nerve (II cranial nerve).. Anatomy of the Visual System Photoreceptors Opsin A class of protein that, together with retinal, constitutes the photopigments. Retinal A chemical synthesized from vitamin A; joins with an opsin to form a photopigment. Rhodopsin A particular opsin found in rods. The pigment, called 15 iodopsin or rhodopsin, consists of large proteins called opsin and retinal (a derivative of vitamin A). Visual Transduction L membrane NA+ channels are open -> glutamate is released which depolarizes the membrane Light(photons) strikes rhodopsin and splits the opsin and retinal apart-> The retinal exists in the 11-cis-retinal form when in the dark, and stimulation by light causes its structure to change to all-trans-retinal. Activates transducin (G protein)-> Activates cGMP phosphodiesterase-> cGMP Phosphodiesterase transforms cGMP to 5′-GMP, which causes Na+ channels (which had been held open by the cGMP) to close. Reduces cGMP -> closes NA+ channels Visual Pathway Ganglion cell axons form the optic nerve The optic chiasm (base of the hypothalamus) is the place where the two optic nerves leaving the eye meet Information from the right visual field (now on the left side of the brain) travels in the left optic tract. Information from the left visual field travels in the right optic tract. Each optic tract terminates in the lateral geniculate nucleus (LGN) in the thalamus. Sends axons to other parts of the thalamus and to the visual areas of the occipital cortex About 90% of the axons in the optic nerve go to the lateral geniculate nucleus in the thalamus. Another population sends information to the superior colliculus in the midbrain, which assists in controlling eye movements as well as other motor responses. The region that receives information directly from the LGN is called the primary visual cortex, (also called V1 and Visual Pathways Information from each visual field crosses over at the optic chiasm and projects to the opposite side of the primary visual cortex Visual association cortex Along with this increasing complexity of neural representation may come a level of specialization of processing into two distinct pathways: the dorsal stream and the ventral stream (the Two Streams hypothesis, The dorsal stream, commonly referred to as the "where" or “how” stream, is involved in spatial attention (covert and overt), and communicates with regions that control eye movements and hand movements. The ventral stream, commonly referred as the "what" stream, is involved in the recognition, identification and categorization of visual stimuli Ventral stream damage: can see where objects are but cannot identify them Dorsal stream damage: can identify objects but not know where they are Color Processing Theory Color Vision Visible light is a portion of the electromagnetic spectrum The perception of color is dependent upon the wavelength of the light “Visible” wavelengths are dependent upon the species’ receptors Humans perceive wavelengths between 400 and 700 nanometers (nm) © 2019 Cengage. All rights reserved. Trichromatic Theory Red cones: Most sensitive to red light (long wavelengths). Green cones: Most sensitive to green light (medium wavelengths). Blue cones: Most sensitive to blue light (short wavelengths). 1. Light Stimulation: When light enters the eye, it stimulates the cone photoreceptor cells. 2. Signal Generation: Each type of cone cell generates a signal based on the intensity of light it receives. 3. Brain Interpretation: The brain interprets the combined signals from the three types of cones to perceive different colors. © 2019 Cengage. All rights reserved. The Opponent-Process Theory Red and green: The perception of red inhibits the perception of green, and vice versa. Blue and yellow: The perception of blue inhibits the perception of yellow, and vice versa. Black and white: The perception of black inhibits the perception of white, and vice versa. Afterimages: A key phenomenon supporting this theory is the appearance of afterimages. For example, if you stare at a red object for an extended period and then look at a white surface, you may see a green afterimage. This is because the red cones in your retina become fatigued, and the green cones become relatively more active. © 2019 Cengage. All rights reserved. Limitations of Color Vision Theories Both the opponent-process and trichromatic theory have limitations – Color constancy, the ability to recognize color despite changes in lighting, is not easily explained by these theories Retinex theory suggests the cortex compares information from various parts of the retina to determine the brightness and color for each area © 2019 Cengage. All rights reserved. Color Vision Deficiency An impairment in perceiving color differences – Gene responsible is contained on the X chromosome – Caused by either the lack of a type of cone or a cone that has abnormal properties – Most common form is difficulty distinguishing between red and green Results from the long- and medium-wavelength cones having the same photopigment © 2019 Cengage. All rights reserved. Receptive fields Receptive fields are the regions of the visual field that, when stimulated, cause a change in the firing rate of a neuron. In the retina, these neurons include photoreceptor cells, bipolar cells, and ganglion cells. On-Center and Off-Center Cells On-center cells: These cells increase their firing rate when light is shone onto the center of their receptive field. They decrease their firing rate when light is shone onto the periphery of their receptive field. Off-center cells: These cells increase their firing rate when light is shone onto the periphery of their receptive field. They decrease their firing rate when light is shone onto the center of their receptive field. Edge Detection: Receptive fields are involved in edge detection, which is the ability to identify the boundaries between objects. On-center and off-center cells can detect edges by responding to changes in light intensity across their receptive fields. Contrast Enhancement: Receptive fields contribute to contrast enhancement, which is the process of increasing the perceived difference between light and dark areas in an image. Motion Detection: Receptive fields are also involved in motion detection. The temporal properties of receptive fields, such as their response to changes in light intensity over time, can help to detect moving objects. Pattern Recognition: Receptive fields can be combined to form more complex patterns, such as lines, edges, and corners. These patterns can be used to recognize objects and scenes. Primate Receptive Fields Ganglion cells of primates generally fall into three categories – Parvocellular neurons – Magnocellular neurons – Koniocellular neurons © 2019 Cengage. All rights reserved. Magnocellular Koniocellular Neurons Parvocellular Neurons Neurons Cell bodies Small Large Small Receptive fields Small Large Mostly small, but variable Retinal location In and near fovea Throughout the retina Throughout the retina Color sensitive? Yes No Some are Respond to Detailed shape Movement and broad Varied outlines of shape Visual Fields in the Visual Cortex Hubel & Wiesel (1959) – record activity from cat occipital cortex They quickly realized that the cell was responding to the edge of the slide. It had a bar-shaped receptive field, rather than a circular receptive field like cells in the retina and lateral geniculate. Hubel and Weisel (1959, 1998) distinguished various types of cells in the visual cortex – Simple cells – Complex cells – End-stopped/hypercomplex cells https://www.youtube.com/watch?v=0ugcw7wOZBg Simple, complex, and hypercomplex cells are types of neurons found in the primary visual cortex (V1) of the brain. They play crucial roles in visual processing, particularly in feature extraction and object recognition. Simple Cells Receptive Fields: Simple cells have elongated, rectangular receptive fields with distinct on and off regions. Position Sensitivity: Simple cells are also sensitive to the position of a stimulus within their receptive field. Complex Cells Receptive Fields: Complex cells have larger receptive fields than simple cells and are less sensitive to the exact position of a stimulus. Orientation and Movement Sensitivity: Complex cells are both orientation-selective and movement-sensitive. They respond to a stimulus of a particular orientation, regardless of its position within their receptive field, and can also detect motion in a particular direction. Hypercomplex Cells Length Tuning: Hypercomplex cells are tuned to specific lengths of a stimulus. Some hypercomplex cells respond best to short stimuli, while others respond best to long stimuli. Applications of Simple, Complex, and Hypercomplex Cells These types of cells play crucial roles in various visual tasks, including: Object Recognition: Simple, complex, and hypercomplex cells can extract features from visual stimuli, such as edges, corners, and lines. These features can then be combined to recognize objects. Motion Perception: Complex and hypercomplex cells are involved in motion perception, allowing us to detect and track moving objects. Spatial Vision: These cells contribute to our ability to perceive the spatial layout of the world, including depth, distance, and size. Detailed Analysis of Shape Receptive fields become larger and more specialized as visual information goes from simple cells to the complex cells and then to other brain areas The inferior temporal cortex contains cells that respond selectively to complex shapes but are insensitive to distinctions that are critical to other cells Cells in this cortex respond to identifiable objects Image: https://psychology.stackexchange.com/questions/132 56/do-direct-cortical-pathways-exist-in-the-visual- system-or-do-they-all-go-via-th Motion Blindness The inability to determine the direction, speed and whether objects are moving – Likely caused by damage in area MT(middle temporal cortex) Some people are blind except for the ability to detect which direction something is moving – Area MT probably gets some visual input despite significant damage to area V1 © 2019 Cengage. All rights reserved. Stereoscopic Depth Perception A method of perceiving distance in which the brain compares slightly different inputs from the two eyes – Relies on retinal disparity or the discrepancy between what the left and the right eye sees – The ability of cortical neurons to adjust their connections to detect retinal disparity is shaped through experience © 2019 Cengage. All rights reserved. Strabismus A condition in which the eyes do not point in the same direction – Usually develops in childhood – Also known as “lazy eye” If two eyes carry unrelated messages, cortical cell strengthens connections with only one eye Development of stereoscopic depth perception is impaired © 2019 Cengage. All rights reserved. Visual Agnosia The inability to recognize objects despite satisfactory vision – Caused by damage to the pattern pathway usually in the temporal cortex Able to point to visual objects and slowly describe them but fail to recognize what they are. © 2019 Cengage. All rights reserved. Recognizing Faces Fusiform Gyrus, especially in the right hemisphere, responds strongly to faces than to other objects Face recognition occurs relatively soon after birth Facial recognition continues to develop gradually into adolescence Prosopagnosia The impaired ability to recognize faces – Occurs after damage to the fusiform gyrus of the inferior temporal cortex – The fusiform gyrus responds much more strongly to faces than anything else When people with prosopagnosia can read, so visual acuity is not the problem. They recognize people’s voices, so their problem is not memory. people with prosopagnosia look at a face, they can describe each element of a face, such as brown eyes, big ears, a small nose, and so forth, but they do not recognize the face as a whole. © 2019 Cengage. All rights reserved. Auditory Hearing alerts us to many types of useful information Auditory signals are sensed as periodic compressions of air, water, or other media Humans experience hearing by detecting sound waves Sound waves are periodic compressions of air, water, or other media Sound waves vary in amplitude and frequency Physics of Sound Structures of the Ear—The Outer Ear Anatomists distinguish the outer ear, the middle ear, and the inner ear The outer ear includes the pinna, the structure of flesh and cartilage attached to each side of the head Responsible for: – Altering the reflection of sound waves into the middle ear from the outer ear – Helping us to locate the source of a sound MIDDLE EAR Ossicular Chain The middle ear is connected and transmits sound to the inner ear via the ossicular chain. The ossicular chain consists of the three smallest bones in the body: the malleus, incus, and stapes. The malleus is attached to the tympanic membrane. The incus is between the malleus and the stapes. The footplate of the stapes inserts into the oval window of the inner ear. These muscles contract to protect the inner ear by reducing the intensity of sound transmission to the inner ear from external sounds and vocal transmission. Structures of the Ear—The Inner Ear The inner ear contains a snail shaped structure called the cochlea – Contains three fluid-filled tunnels – Hair cells are auditory receptors that lie between the basilar membrane and the tectorial membrane in the cochlea – When displaced by vibrations in the fluid of the cochlea, they excite the cells of the auditory nerve by opening ion channels Cochlea The hearing part of the inner ear is the cochlea. The cochlea is spiral-shaped, similar to the shape of a snail. The cochlea is composed of three fluid-filled chambers that extend the length of the structure. The two outer chambers are filled with a fluid called perilymph. Perilymph acts as a cushioning agent for the delicate structures that occupy the center chamber. It is important to note that perilymph is connected to the cerebrospinal fluid that surrounds the brain and the spinal column. The third fluid filled chamber is the center chamber, called the cochlear duct. The cochlear duct secretes a fluid called endolymph, which fills this chamber. Cochlea The cochlear duct contains the Basilar membrane upon which lies the Organ of Corti. The Organ of Corti is a sensory organ essential to hearing. It consists of approximately 30,000 finger-like projections of cilia that are arranged in rows. These cilia are referred to as hair cells. Each hair cell is connected to a nerve fiber that relays various impulses to the cochlear branch of the VIIIth cranial nerve or auditory nerve. The "pitch" of the impulse relayed is dependent upon which areas of the basilar membrane, and hence, which portions of the Organ of Corti are stimulated. The apical portion of the basilar membrane (the most curled area of the cochlea) transfers lower frequency impulses. Auditory transduction As the stapes vibrates the oval window, the perilymph sloshes back and forth, vibrating the round window in a complementary rhythm. The membranous labyrinth is caught between the two, and bounces up and down with all this sloshing. The membranous labyrinth of the cochlea encloses the endolymph- filled scala media. The two compartments of the bony labyrinth, which house the perilymph, are called the scalae vestibuli and tympani. Within the scala media is the receptor organ, the organ of Corti. It rests on part of the membranous labyrinth, the basilar membrane. When the traveling wave reaches a particular portion of the basilar membrane, those haircells move up and down with the basilar membrane. Flowing through the fluid in inner ear, the cilia are pushed in the opposite direction of the motion of the hair cell. This flowing motion is called a shearing force. The hair cells are not all the same length. The kinocilium is the longest and the hair cells get shorter as they go away from the kinocilium. Since it is the tallest, the kinocilum catches the most of the fluid and is pushed the hardest. It is similar to the tallest trees in the woods catching the most of a breeze. As a result of this motion, the cilia pull apart pulling the tip links tight. Central auditory pathways: The auditory nerve carries the signal into the brainstem and synapses in the cochlear nucleus. From the cochlear nucleus, auditory information is split into at least two streams, much like the visual pathways are split into motion and form processing. Auditory nerve fibers going to the ventral cochlear nucleus synapse on their target cells with giant, hand-like terminals. The ventral cochlear nucleus cells then project to a collection of nuclei in the medulla called the superior olive. In the superior olive, the minute differences in the timing and loudness of the sound in each ear are compared, and from this you can determine the direction the sound came from. The superior olive then projects up to the inferior colliculus via a fiber tract called the lateral lemniscus. The second stream of information starts in the dorsal cochlear nucleus. Unlike the exquisitely time-sensitive localization pathway, this stream analyzes the quality of sound. Central auditory pathways: The dorsal cochlear nucleus, with fairly complex circuitry, picks apart the tiny frequency differences which make "bet" sound different from "bat" and "debt". This pathway projects directly to the inferior colliculus, also via the lateral lemniscus. The consequence of this is that lesions anywhere along the pathway usually have no obvious effect on hearing. Deafness is essentially only caused by damage to the middle ear, cochlea, or auditory nerve. From the inferior colliculus, both streams of information proceed to sensory thalamus. The auditory nucleus of thalamus is the medial geniculate nucleus. The medial geniculate projects to primary auditory cortex, located on the banks of the temporal lobes. PITCH PERCEPTION : Place theory our ability to perceive pitch is based on the specific location where sound waves cause maximum vibration along the basilar membrane of the cochlea in the inner ear. According to this theory: Different parts of the basilar membrane respond to different frequencies of sound. High-frequency sounds stimulate the base (narrow, stiff part) of the cochlea. Low-frequency sounds stimulate the apex (wider, more flexible part) of the cochlea. The brain interprets the frequency of a sound by identifying the specific location on the membrane where the vibration occurs most intensely. PITCH PERCEPTION : Frequency theory frequency of a sound wave is directly encoded by the rate at which neurons in the auditory system fire. According to this theory, the auditory nerve fibers fire action potentials (nerve impulses) at a rate corresponding to the frequency of the sound wave. For example, if a sound wave has a frequency of 100 Hz, the auditory neurons fire 100 times per second. This theory works well for explaining how we perceive low-frequency sounds (typically below 1,000 Hz) because at lower frequencies, the firing rate of neurons can keep up with the sound wave’s frequency. However, frequency theory has limitations at higher frequencies, where neurons cannot fire fast enough to match the high rate of the sound wave directly. Sound Localization Depends upon comparing the responses of the two ears Three cues: – Sound shadow – Time of arrival – Phase difference Humans localize low frequency sound by phase difference and high frequency sound by loudness differences Three Mechanisms of Sound Localization High-frequency sounds (2000–3000 Hz) create a “sound shadow” Difference in time of arrival at the two ears most useful for localizing sounds with sudden onset Phase difference between the ears provides cues to sound localization with frequencies up to 1500 Hz Individual Differences “Amusia”: the impaired detection of frequency changes (tone deafness) – Around 4 percent of people experience amusia Associated with thicker than average auditory cortex in the right hemisphere, but fewer connections from auditory cortex to frontal cortex Variations in Sensitivity to Pitch Absolute pitch (“perfect pitch”) is the ability to hear a note and identify it – Genetic predisposition may contribute to it – The main determinant is early and extensive musical training More common among people who speak tonal languages, such as Vietnamese and Mandarin Chinese Hearing Loss Two categories of hearing impairment – Conductive or middle ear deafness – Nerve deafness or inner ear deafness Conductive/Middle Ear Deafness Occurs if bones of the middle ear fail to transmit sound waves properly to the cochlea Can be caused by disease, infections, or tumorous bone growth Normal cochlea and auditory nerve allow people to hear their own voice clearly Can be corrected by surgery or hearing aids that amplify the stimulus Nerve or Inner-Ear Deafness Results from damage to the cochlea, the hair cells, or the auditory nerve Can vary in degree Can be confined to one part of the cochlea – People can hear only certain frequencies Can be inherited or caused by prenatal problems or early childhood disorders Tinnitus Frequent or constant ringing in the ears – Experienced by many people with nerve deafness Sometimes occurs after damage to the cochlea – Axons representing other part of the body innervate parts of the brain previously responsive to sound – Similar to the mechanisms of phantom limb Tinnitus is often associated with: age-related hearing loss inner ear damage caused by repeated exposure to loud noises an earwax build-up a middle ear infection Ménière's disease – a condition that also causes hearing loss and vertigo (a spinning sensation) otosclerosis – an inherited condition where an abnormal bone growth in the middle ear causes hearing loss Vestibular Sensation The vestibular sense: system that detects the position and movement of the head – Directs compensatory movements of the eye and helps to maintain balance The vestibular organ is in the ear and is adjacent to the cochlea https://www.youtube.com/watch?v=a_rwZBQeu14&list= PLkKvotUGCyLdTabTWbX2b5ZVdHYaSW-73&index=1 0 Vestibular Organ Comprises otolith organ and three semicircular canals Otolith Organs Function: Detect linear acceleration and gravity. Types: Utricle and saccule. Role in balance: Help maintain balance when standing or walking by providing information about the body's orientation with respect to gravity. Semicircular Canals Function: Detect angular acceleration (rotational movement of the head). Role in balance: Help stabilize gaze during head movements and maintain balance when turning or spinning. SemiCircular Canals Three canals: The semicircular canals are arranged in three different planes: horizontal, anterior (superior), and posterior. Ampulla: At the base of each canal is a dilated region called the ampulla, which contains sensory hair cells. Endolymph: The canals are filled with a fluid called endolymph, which moves in response to head movement. Cupula: The hair cells are embedded in a gelatinous structure called the cupula, which extends into the endolymph. Process of Sensing Head Movement: 1. Fluid movement: When the head moves, the endolymph within the semicircular canals also moves. This movement is due to the inertia of the fluid, which resists changes in motion. 2. Cupula deflection: The movement of the endolymph causes the cupula to bend, which in turn bends the hair cells embedded within it. 3. Neural signal: The bending of the hair cells triggers the release of neurotransmitters, which generate electrical signals that are sent to the brain. 4. Brain interpretation: The brain interprets these signals to determine the direction and speed of head movement. Figure 7.15 66 The Receptive Organ of the Semicircular Canals Otolith Organs: Both the utricle and saccule are otolith organs, meaning they contain small, calcium carbonate crystals called otoliths. These otoliths are embedded in a gelatinous membrane. Hair Cells: Within the gelatinous membrane are hair cells, specialized sensory cells that detect movement. The hair cells have stereocilia, which are tiny, hair-like projections that extend into the gelatinous membrane. Orientation: The utricle is horizontally oriented, while the saccule is vertically oriented. This orientation allows them to detect acceleration in different planes. Process of Detecting Linear Acceleration: 1. Otolith Movement: When the head undergoes linear acceleration, the otoliths within the gelatinous membrane shift due to their inertia. This movement causes the gelatinous membrane to bend. 2. Hair Cell Bending: The bending of the gelatinous membrane causes the stereocilia of the hair cells to bend. This bending of the stereocilia opens or closes ion channels in the hair cells. 3. Neural Signal: The change in ion channels leads to a change in the electrical potential of the hair cells. This change in electrical potential generates a neural signal that is transmitted to the brain via the vestibular nerve. Figure 7.16 68 The Receptive Tissue of the Vestibular Sacs: the Utricle and the Saccule Somatosensory Receptors Touch receptors may be: – Simple bare neuron ending – A modified dendrite (Merkel disks) – An elaborated neuron ending – A bare ending surrounded by non-neural cells that modify its function Stimulation opens sodium channels to trigger an action potential Figure 7.17 70 Cutaneous Receptors Anatomy of the Skin and Its Receptive Organs There are two categories of thermal receptors: those that respond to warmth and those that respond to coolness. Cold sensors in the skin are located just beneath the epidermis, and warmth sensors are located more deeply in the skin. Information from cold sensors is conveyed to the CNS by thinly myelinated Aδ fibers, and information from warmth sensors is conveyed by unmyelinated fibers 71 Peripheral Receptors: These are specialized nerve endings located throughout the body that detect sensory stimuli. They include: Mechanoreceptors: Sensitive to mechanical deformation, such as touch, pressure, and vibration. Thermoreceptors: Sensitive to temperature changes. Nociceptors: Sensitive to pain. Proprioceptors: Sensitive to joint position and muscle tension. Sensory Neurons: They are classified based on their size and myelination: A-alpha fibers: Large, myelinated fibers that transmit proprioceptive information and touch sensation. A-beta fibers: Smaller, myelinated fibers that transmit touch and pressure sensation. A-delta fibers: Smaller, myelinated fibers that transmit fast pain and temperature sensation. C fibers: Unmyelinated fibers that transmit slow pain, temperature, and itch sensation. Somatosensory Receptors and Probable Functions Receptor Location Responds to Free nerve Ending Any skin area Pain and temperature Hair-follicle receptors Hair-covered skin Movement of hairs Meissner’s corpuscles Hairless areas Movement across the Skin Vibration or sudden Pacinian corpuscles Any skin area Touch Merkel’s disks Any skin area Static touch Ruffini endings Any skin area Skin stretch Mostly Krause and bulbs hairless areas Uncertain Dorsal Column-Medial Lemniscus Pathway: This pathway carries information about fine touch, vibration, and proprioception. The neurons enter the spinal cord through the dorsal root and ascend in the dorsal column. In the medulla oblongata, these fibers cross over to the medial lemniscus in the midbrain to the thalamus. Spinothalamic Tract: This pathway carries information about pain and temperature. The neurons enter the spinal cord through the dorsal root and ascend in the spinothalamic tract to the thalamus. Trigeminal Pathway: This pathway carries sensory information from the face, head, mouth, and nasal cavity. The neurons enter the brainstem through the trigeminal nerve ascend to the thalamus. The thalamus is a relay station for sensory information. It receives sensory signals from the spinal cord and brainstem and relays them to the cerebral cortex. This nucleus projects to the primary somatosensory cortex. Spinal Pathways for Touch and Pain The Somatosensory Cortex Various aspects of body sensations remain separate all the way to the cortex – Various areas of the somatosensory thalamus send impulses to different areas of the somatosensory cortex located in the parietal lobe – Different sub areas of the somatosensory cortex respond to different areas of the body – Damage to the somatosensory cortex can result in the impairment of body perceptions Pain The experience evoked by a harmful stimulus and directs one’s attention toward a danger – Pain sensation begins with the least specialized of all receptors (bare nerve endings) – Some pain receptors also respond to acids, heat, or cold Emotional associations of pain – Activate a path that goes through the reticular formation of the medulla – And then to several of the central nuclei of the thalamus, the amygdala, hippocampus, prefrontal cortex, and cingulate cortex Experimenters monitored people’s brain activity and found hurt feelings activate similar pathways as physical pain (cingulate cortex) Relieving Pain Opioid mechanisms are systems that are sensitive to opioid drugs and similar chemicals Opiates bind to receptors found mostly in the spinal cord and the periaqueductal gray area of the midbrain Endorphins: group of chemicals that attach to the same brain receptors as morphine – Different types of endorphins for different types of pain Gate Theory Open Gate: When receptors are activated, they send signals along A-δ and C fibers to the spinal cord. If the gate is open, these signals are transmitted to the brain, resulting in the perception of pain. Closed Gate: The gate can be closed by several factors: Non-painful stimuli: Activities like rubbing or massaging the affected area can stimulate A-β fibers (touch and pressure fibers). These fibers can activate inhibitory interneurons in the spinal cord, which close the gate and reduce pain perception. Descending inhibitory pathways: The brain can send signals down to the spinal cord to close the gate, reducing pain perception. This can occur through various mechanisms, such as the release of endorphins (natural pain-relievers). Emotional factors: Psychological factors, such as stress, anxiety, or mood, can also influence the perception of pain by affecting the activity of the pain gate. These other areas that provide input can close the “gates” by releasing endorphins and decrease pain perception Taste Receptors Receptors for taste are modified skin cells Taste receptors have excitable membranes that release neurotransmitters to excite neighboring neurons Taste receptors are replaced every 10–14 days Papillae are structures on the surface of the tongue that contain the taste buds Each papillae may contain up to ten or more taste buds Each taste bud contains approximately 50 receptors Most taste buds are located along the outside edge of the tongue in humans The Organs of Taste Taste buds: These are sensory organs located on the tongue, soft palate, and epiglottis. They contain taste cells that are sensitive to specific tastes. Taste receptors: Each taste cell has taste receptors that bind to specific molecules in food. There are five primary tastes: sweet, sour, salty, bitter, and umami (savory). Signal Transduction Chemical reactions: When taste molecules bind to their respective receptors, a series of chemical reactions occurs within the taste cell. These reactions lead to the opening or closing of ion channels. This generates an electrical signal. Nerve Impulse Transmission Cranial nerves: The electrical signals from the taste buds are transmitted to the brain via three cranial nerves: the facial nerve (CN VII), the glossopharyngeal nerve (CN IX), and the vagus nerve (CN X). Gustatory information from the anterior part of the tongue travels through the chorda tympani, a branch of the facial nerve that passes beneath the eardrum on its way to the brain.. The posterior part of the tongue sends gustatory information through the glossopharyngeal nerve, the palate and epiglottis send gustatory information through the vagus nerve Brainstem: These nerves carry the taste signals to the brainstem, where they synapse with neurons in the nucleus tractus solitarius in the medulla. Relay to Thalamus Thalamus: From the NTS, the taste signals are relayed to the thalamus, a major relay center in the brain. Primary Gustatory Cortex Primary gustatory cortex: The thalamus then projects the taste signals to the primary gustatory cortex, in the cerebral cortex. Taste perception: In the primary gustatory cortex, the brain interprets the taste signals and creates a conscious perception of taste. Gustatory information is also sent to the amygdala, hypothalamus, and basal forebrain. Figure 7.23 Neural 84Pathways of the Gustatory System. Adaptation and Cross-Adaptation Adaptation refers to reduced perception of a stimuli due to the fatigue of receptors (same) Cross-adaptation refers to reduced response to one stimuli after exposure to another Although we describe tastes as sweet, sour, salty, and bitter as the “primary,” evidence suggests a fifth type of glutamate receptor (umami) – MSG – Tastes like unsalted chicken broth Some research suggests that the ability to taste fats is a sixth type of taste – For now, this is being called oleogustus Olfactory Receptors Olfactory cells line the olfactory epithelium in the rear of the nasal passage and are the neurons responsible for smell Olfactory receptors are located on cilia, which extend from the cell body into the mucous surface of the nasal passage Vertebrates have hundreds of olfactory receptors, which are highly responsive to some related chemicals and unresponsive to others Nasal Cavity: Odorant molecules enter the nasal cavity through the nostrils. Olfactory Epithelium: The olfactory epithelium, a specialized tissue located in the upper part of the nasal cavity, contains olfactory receptor cells. Odorant Binding: Odorant molecules bind to specific receptors on the cilia of olfactory receptor cells. Signal Transduction: G-protein Activation: Binding of odorant molecules to receptors activates G-proteins within the olfactory receptor cells. cAMP Production: Activated G-proteins stimulate the production of cyclic adenosine monophosphate (cAMP). Ion Channel Opening: cAMP opens cation channels, allowing sodium and calcium ions to enter the cell, depolarizing it. Action Potential Generation in the olfactory receptor cell. Axon Projection: Olfactory Nerve: Axons of olfactory receptor cells bundle together to form the olfactory nerve. Olfactory Bulb: The olfactory nerve projects to the olfactory bulb, a structure located in the brain. Synaptic Transmission: Glomeruli: In the olfactory bulb, axons of olfactory receptor cells synapse with dendrites of mitral cells Projection to Higher Brain Centers: Olfactory Tract: Mitral project their axons to the olfactory tract from the olfactory bulb to various regions of the brain. Primary Olfactory Cortex: The olfactory tract primarily projects to the primary olfactory cortex, located in the temporal lobe. Higher Brain Centers: From the primary olfactory cortex, signals are further processed and integrated with other sensory modalities in higher brain centers, such as the hippocampus, amygdala, and orbitofrontal cortex. Olfactory Receptors Image Figure 7.27 Connections of Olfactory Receptor Cells with Glomeruli. Each glomerulus receives information from only one type of receptor cell. Olfactory receptor cells of different colors contain different types of receptor molecules. 90 Figure 7.28 Coding of Olfactory Information. A hypothetical explanation of coding of olfactory information shows that different odorant molecules attach to different combinations of receptor molecules. (Activated receptor molecules are shown in blue.) Unique patterns of activation represent particular odorants. Adapted from Malnic, B., Hirono, J, Sato, T., and Buck, L. B. Cell, 1999, 96, 713–723. 91 Midterm Midterm (2/10), Conducted in class Midterm (215 pm – 315 pm) ; Class as usual (330 pm – 5 pm) MCQs (Week 1 – Week 6): Neuron & Synapses – Auditory, Mechanical & Chemical Senses Please bring your pencil https://forms.office.com/r/ 1j0TmDSHPn