Lecture 6 - Reciprocating Engines and Fuel Economy PDF
Document Details
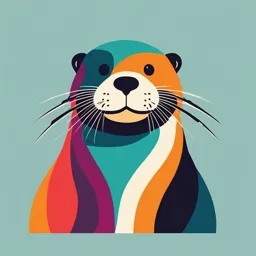
Uploaded by BountifulAgate2489
Cal State LA
2020
Mario Medina
Tags
Summary
This lecture covers reciprocating engine cycles, focusing on Otto and Diesel cycles, and discussions of efficiency. The document also touches on cold start emissions and related topics.
Full Transcript
Cold start emissions https://www.ucair.org/blog/what-is-a-cold-start/ Planet of the Humans discussion https://padlet.com/medinac493/mtwkoyyz2uy7vv2d Reciprocating Engine Cycles ME 4180 – Energy Systems and Sustainability Prof. Mario Medina Department of Mechanical E...
Cold start emissions https://www.ucair.org/blog/what-is-a-cold-start/ Planet of the Humans discussion https://padlet.com/medinac493/mtwkoyyz2uy7vv2d Reciprocating Engine Cycles ME 4180 – Energy Systems and Sustainability Prof. Mario Medina Department of Mechanical Engineering Sept. 21, 2020 Agenda and Outline Objective Agenda Thermodynamic theory for ICE: Otto and Diesel Engine efficiencies Real engine cycles Advance engine strategies Fuel economy Speaking language of engines Gasoline engines = spark ignited (SI); modeled by Otto cycle Fuel injected early in cycle, fuel and air are inducted together by piston motion Premixed fuel/air system Diesel engines = compression ignited (CI); modeled by Diesel cycle Only air is inducted and compressed Fuel injected at end of compression stroke (top dead center = TDC) Engines are characterized by fuel properties © Moran and Shapiro, Fundamentals of Engineering Thermodynamics Cycle analysis: Otto Consists of 4 processes: 12 Isentropic compression (intake/compression Air standard analysis assumes: stroke) 1. Closed system = control mass 23 Const. volume heat addition (combustion) 2. Working fluid = air (ideal gas) 3. ideal cycle (Sgen = 0) 34 Isentropic expansion (power stroke) 4. neglect changes in kinetic and potential 41 Const. volume heat removal (exhaust stroke) energy 5. Only form of work is expansion/compression work Qin Qin 6. Otto = constant volume heat addition 2Q3 = ∆U = maircv ∆T = maircv(T3-T2) 7. Specific heats (Cp, Cv) are constant Qout Qout Moran and Shapiro, Fundamentals of Engineering Thermodynamics, 4th Edition, Instructors Resource, © John Wiley & Sons, 2000. Cycle analysis: Otto Consists of 4 processes: Wcycle 12 Isentropic compression (intake/compression ηcycle = stroke) Qin 23 Const. volume heat addition (combustion) 34 Isentropic expansion (power stroke) 41 Const. volume heat removal (exhaust stroke) T1 1 ηcycle 1− = = 1− k −1 Qin Qin T 2 CR Qout Qout Moran and Shapiro, Fundamentals of Engineering Thermodynamics, 4th Edition, Instructors Resource, © John Wiley & Sons, 2000. Otto cycle efficiency As CR ∞, η 1 CR = 10 η ≅ 60% Diminishing returns (%) Many practical limitations to increase CR Manufacturing Material strength (mechanical/thermal stress) Knock (auto-ignition) ηOtto = 60.2% (CR = 10) TL 300 ηCarnot = 1− ≈ 1− = 86% TH 2200 Cycle analysis: Diesel Consists of 4 processes: Qin 12 Isentropic compression (intake/compression stroke) 23 Const. pressure heat addition(combustion) 34 Isentropic expansion (power stroke) Qout 41 Const. volume heat removal (exhaust stroke) V1 Wcycle W2 + 2 W3 +3 W4 C v (T4 − T1 ) CR = ≈ 15 − 20 4 Q1 V2 ηcycle = = 1 1− = 1− = Qin 2 Q3 2 Q3 Cp (T3 − T2 ) V4 ER = < CR 1 ( CR / ER ) − 1 k 1 T1 (T4 /T1 − 1) 1 V3 1− = 1− = k T2 (T3 /T2 − 1) CR k ( CR / ER ) − 1 k −1 CR = compression ratio, ER = expansion ratio First law efficiency So it’s all about compression ratio… Otto eff. > diesel eff. SI engine eff. > CI engine eff. … but its not always apples to apples Different fuels and different processes Otto vs Diesel Otto cycle (const. vol. heat addition) delivers more power Blarigan and Goldsborough, 2003 than Diesel cycle (const. P heat addition) Gasoline engine is constrained by fuel properties = lower CR Typical CR SI gasoline CR = 10:1 to 12:1 DI diesel CR = 15:1 to 20:1 Materials limited by peak pressures and maximum rates of pressure rise Real engine P-v diagram Moran and Shapiro, Fundamentals of Engineering Thermodynamics, 4th Edition, Instructors Resource, © John Wiley & Sons, 2000. Throttling losses SI engine: both fuel and air are throttled to control load (power) CI engine: load is controlled by fuel injection pulse width Throttling air takes work – a lot, up to 20% of power out Area is net work required; eliminate C.R. Ferguson , Internal Combustion Engines: Applied Thermosciences, John Wiley and Sons, © 1986 pumping work increase efficiency In-cylinder images of SI engine Retarding spark timing Duration of combustion E.V. I.V. Start of combustion Chemilluminescence during Otto cycle combustion – Zigler, B. T., et al.., “An Imaging Study of Compression Ignition Phenomena of Iso-Octane, Indolene, and Gasoline piston view imaging, indolene fuel Fuels in a Single-Cylinder Research Engine,” ASME Journal ** see supplemental material for engine details. of Engineering for Gas Turbines and Power, 2007 Can we improve ICE efficiency? HCCI–TC LEAN-SI-TC ADV–TC TC = turbocharging NA = naturally aspirated (unboosted) etab_v_bmep_AIR_TC50_EIVC_Throt 50 HCCI ADV. SI ADV-NA COMB BMEP = brake mean effective pressure 6 (power) 40 3 5 η_brake (%) 4 2.0 2.5 3.0 ηbrake = overall thermal efficiency = (shaft 2 1.5 P_in (bar) work out)/(fuel energy content in) 1.0 1 30 SI–TC (Φ=1) HCCI = homogeneous charge SI–NA, Throttled (Φ=1) compression ignition CR = 12; RPM = 2000 20 0 10 20 30 40 Why improve gasoline engine? BMEP (bar) Lavoie, G. A., Assanis, D., and Ortiz-Soto, E. (2011) Thermodynamic Sweet Spot under Highly Dilute and Boosted Gasoline Engine Conditions, Presented at the SAE 2011 High Efficiency IC engines Symposium, Detroit, Michigan, April 10-11, 2011. Fuel economy benefits Advanced combustion and boosting CASE Combustion Mode Air Handling Size City/Hwy (mpg) FE GAIN with downsizing independently 1 Stoich-SI NA 3.3 L 25.4 BASE increase fuel economy 2 3 Advanced HCCI NA 3.3 L 31.3 23% TC 3.3 L 31.2 23% 4 Stoich-SI TC 1.4 L 34.5 36% Combine both strategies for best 5 Lean-SI TC 1.4 L 36.7 44% 6 Advanced TC 1.4 L 40.3 58% results Combined City/Hwy Fuel Economy 50 Fuel Economy (MPG) GAIN OVER BASE + 58% 40 + 44% + 36% + 23% + 23% Fuel economy gains of 58% are 30 + 0% possible 20 10 1 2 3 4 5 6 0 Stoich-SI Advanced HCCI Stoich-SI Lean-SI Advanced NA NA TC TC TC TC 3.3L 3.3 L 3.3 L 1.4 L 1.4 L 1.4 L Lavoie, G. A., Assanis, D., and Ortiz-Soto, E. (2011) Thermodynamic Sweet Spot under Highly Dilute and Boosted Gasoline Engine Conditions, Presented at the SAE 2011 High Efficiency IC engines Symposium, Detroit, Michigan, April 10-11, 2011. Advanced ICE strategies Next gen of SI/gasoline engines use two methods to increase efficiency: 1) Direct injection of fuel into cylinder and increasing intake pressure (i.e. boosting); both methods have been demonstrated successfully and are in production 2) Low temperature strategies; only demonstrated at limited conditions, no vehicle prototype Gasoline direct injection Advantages GDI has a “gamma” advantage, compressing mostly air (injecting fuel late for stratified charge), increasing cycle efficiency through the ratio of the specific heats (γ = k = cp/cv). (Recall ηOtto = 1 -1/CR(k-1), so you want a high k where kair=1.4, kCO2 = 1.289, kCO = 1.4, kHe=kAr=1.67, kburned = 1.2-1.27, kgasoline+air = 1.25-1.35 compressing air is better than compressing fuel and air) Air throttling losses are eliminated; increasing cycle efficiency. Evaporative cooling allows a higher compression ratio – increasing cycle efficiency Burn fuel lean charges (40:1 air-fuel charges; up to 65:1!; compared to 14.7:1 for φ = 1), thus decreasing temperatures and decreasing emissions Load is controlled through quantity of fuel added to the engine, not through air Can achieve more precise control over fuel quantities and therefore further improve fuel economy Challenges GDI engines require robust high-pressure fuel injectors that can endure the in-cylinder conditions At higher loads, spontaneous pre-ignition can occur “Superknock” Alternative cycles – Atkinson cycle The intake valve open during a portion of compression stroke reducing the effective compression ratio of the engine (12) The combustion (i.e. heat addition) is considered a combination of Otto and diesel steps (like a dual cycle). The expansion stroke is greater than the compression stroke. Advantages: 1. Less work required during compression, higher cycle efficiencies 2. Can use higher compression ratios (the CR of a conventional Otto/SI engine is knock limited), increasing cycle efficiency. 3. Longer power stroke, extracting more energy from exhaust gases. Disadvantages: Smaller volume of air in-cylinder (less charge) lower power (this yields a lower power density engine) (Can be addressed using a turbo- or supercharger to boost pressure. This is called a Miller cycle) The Atkinson cycle has been used in production hybrid power trains (e.g. Toyota Prius and Camry, Ford Escape/Mazda Tribute), where high fuel efficiency is desirable and the power penalty is not as large a concern. Engine emissions How much CO2 reduction is achieved if SI engine efficiency improved by 25%? C8H18 + 12.5(O2 + 3.76N2) → 8CO2 + … ∆(Increase in η) = ∆(Reduction in mCO2) Direct correlation between fuel and CO2, so 25% reduction in CO2 Criteria air pollutant emissions PER VEHICLE MILE TRAVELED have decreased dramatically in the past 30-40 years, but the total vehicle miles traveled has increased Fuel economy is not just about the engine; strong function of powertrain efficiency Definitions of heat engine efficiency First law efficiency: 1 Otto cycle – gasoline – SI engine ηOtto = 1 − k −1 CR CRSI = 8-12, ηOtto = 56-63% 1 1 (CR / ER) k − 1 V4 Diesel cycle – CI engine η Diesel 1− = k −1 , ER = CR k (CR / ER) − 1 V3 CRDiesel= 15-20, ηDiesel= 62-66% (using ER = 0.6CR) In principle, for equivalent CR the Otto cycle is more efficient (i.e. CR = 20, , ηOtto = 70%) In practice, Diesel cycle are more efficient because they can use higher CR (fueling constraints) Definitions of vehicle efficiency Thermodynamic efficiency: Wcycle 1st Law: η1st = Qin Wactual Wactual η2 nd = 2nd Law: = (often for flow devices) Wmax Wisentropic Definitions of vehicle efficiency Practical efficiencies: Based on the energy content of the fuel and work out of engine Wactual m f : mass of fuel ηf = m f QqHV HV QHV : heating value of fuel [kJ/kg f ] qHV Based on power required to propel the vehicle W prop ( Drag + Friction ) V = ηp = m f QqHV m f QqHV drag coeff. frontal area 1 2 = µ= Friction Wvehicle µ mvehicle g Drag = CD ρ air Af V rolling friction coeff. 2 Engine and drivetrain efficiency m f QHV Wshaft - heat losses ηengine = ≈ 20-30% - friction losses Welec m f QqHV HV - throttling losses Engine Q loss Wshaft W prop η DT = ≈ 80% W shaft Transmission Q fric ηtotal = ηengineη DT ≈ 15% W prop All vehicles have to overcome drivetrain efficiency – ICE, EV, hydrogen Fuel economy and engine efficiency Fuel economy = MPG (miles per gallon) ∆m f ∆x ∆x m f = for constant speed, = V , t ∆= ∆t ∆t V ∆m f V W prop ∆m f V W prop m f = Recall ηtotal = m f = = ∆x m f QHV ∆x ηtotal QHV ∆x ρ f ηtotal QHV V MPG = MPG ~ ηtotal ∆m f / ρ f W prop ρ f ηengineη DT QHV V ρ f ηengineη DT QHV MPG = 1 2 1 2 CD ρ air Af V + µWveh V CD ρ air Af V + µWveh 2 2 Distribution of energy in vehicle Wind resistance – drag Rolling resistance – friction Engine losses are the largest source U.S Dept. of Energy, Office of Energy Efficiency and Renewable Energy, 2019 Supplemental Material Cycle Process – Otto E.F. Obert Easley at el., DEER 2005 In-cylinder images of SI engine Single-cylinder optical engine research facility [Sandia] Based on 1.25L Ford Zetec-SE 16V cyl. head Flat Ø71.9mm piston with Ø48.5mm window 76.5mm stroke 0.31L E.V. E.V. displacement at CR=10 PFI with Siemens DEKA II Hydraulic dynamometer ETAS LA3 lambda meter I.V. I.V. Imaged with Vision Research Phantom V.7.1 at 320 x 320 pixels at 3000 fps with 310μs exposure Zigler, B. T., Walton, S. M., Assanis, D., Perez, E., Wooldridge, M. S., and Wooldridge, S. T., “An Imaging Study of Compression Ignition Phenomena of Iso-Octane, Indolene, and Gasoline Fuels in a Single-Cylinder Research Engine,” ASME Journal of Engineering for Gas Turbines and Power, in review 2007. Gasoline direct injection (GDI) Gasoline is directly injected into cylinder; spark is used to ignited mixture Stratified charge is created (partial premixed) Production GDI engines realized >15% in fuel efficiency Production GDI The Japanese automakers came out early (1995-2000) with GDI technologies (Honda, Toyota, Nissan and Mitsubishi). Ford introduced the GDI Ecoboost technology in 2009 (Lincoln MKS model, 3.5L 340 hp turbocharged V6, Explorer 4 cylinder 2L 275 hp,20-30% improvement in fuel economy). GM offered 6 GDI engines in model year 2009 in the U.S. (e.g. 2L Ecotec, 2.4L 182 hp, CR 11.4), and 18 worldwide. Hyundai (announced 2009) – 2.4 Theta II GDI, 4 cylinder 2011 Sonata, 200 Hp, 10% improvement in fuel economy, fuel economy in the 30’s mpg GM Terrain, General Motors © Ford Ecoboost , Ford Motor Company © Market penetration..is absolutely critical!!! Circa 2006, the U.S. passenger vehicle fleet was the largest in the world (251 million vehicles) U.S. owners keep their vehicles for ~5 years (depending on national economy and market segment, e.g. SUVs, pick up trucks are kept longer, and other factors) The U.S. market is saturated at about 14 million new vehicles per year High volume sales are required to have a major/rapid impact on CO2 emissions 20,000 18,000 Retail sales [1000s of units] 16,000 14,000 12,000 10,000 8,000 6,000 4,000 Cars Trucks 2,000 Total 0 1965 1970 1975 1980 1985 1990 1995 2000 2005 2010 2015 Year U.S. Department of Transportation U.S. Census Bureau Soot and particulates Soot - Usually used as a synonym for particulate although some people use it to refer to the material formed in the combustion chamber before hydrocarbons have condensed and/or adsorbed on to it. Kittelson, D. B., Engines and Nanoparticles: A Review. EPA Publication No. EPA 454-R-04-002 J. Aerosol Sci., Vol. 29, No.5/6, pp. 575-588, 1998. Advanced ICE strategies Mueller and Upatnieks, DEER., 2005 HCCI - advantages HCCI is a low temperature mode of engine operation HCCI demonstrated emissions performance High efficiencies, approaching diesel engine efficiencies – b/c higher compression ratios can be used Low temperatures minimize NOx emissions Lean and homogenous reaction minimizes particulate emissions Can use a variety of fuels HCCI challenges FTP driving cycle for a typical SI engine Chang, Babajimopoulos, Lavoie, Filipi, Assanis, SAE Paper No. 2006-01-1087, “Analysis of Load and Speed Transitions in an HCCI Ening Using 1-D Cycle Simulation and Thermal Networks”