Lecture 4-Structural Organization of Membrane Proteins PDF
Document Details
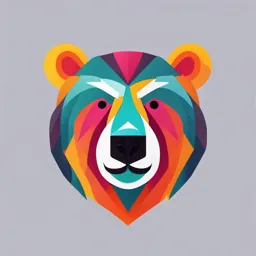
Uploaded by YouthfulGothicArt
2024
Tags
Summary
This lecture covers the structural organization of membrane proteins, including the different types of membrane proteins and their various interactions with lipids and other molecules. Different types of structures such as beta barrels are explained. A key topic is the way amino acid polarity and other factors dictate how these proteins work.
Full Transcript
BIOC*4580 Winter 2024 Lecture 4 B&T Fig. 2.4 Peripheral membrane protein cont. Some proteins are anchored to the membrane by helices that are associated with the surface of the membrane These proteins tend to be anchored in the membrane by amphipathic helices - helices that are hydrophobic on the si...
BIOC*4580 Winter 2024 Lecture 4 B&T Fig. 2.4 Peripheral membrane protein cont. Some proteins are anchored to the membrane by helices that are associated with the surface of the membrane These proteins tend to be anchored in the membrane by amphipathic helices - helices that are hydrophobic on the side buried in the membrane, and hydrophilic on the side facing the cytosol Alternatively, they may interact by a large patch of exposed hydrophobic residues on the surface, often surrounded by basic residues Amphipathic Helix Hydrophobic Hydrophobic Patch in MurG residues View from membrane Basic residues Helical wheel representation of an α helix An α-helix is a regular repetitive pattern with 3.6 amino acids per turn. As amino acids wind around the vertical axis of the helix, certain amino acid residues will fall on the same side of the helix (residues 2, 3 & 6 etc., and 1, 4, 5 etc., in the structure shown) If residues 2, 3 & 6 etc., were all hydrophobic, and 1, 4, 5 etc., were hydrophilic, the helix would have a hydrophilic face and a hydrophobic face This type of a helix is known as an amphipathic helix. Determine the distribution of amino acids along a helical segment given the sequence below: Leu– Gln–Arg–Phe–Val–Thr–Asp–Val–Asn–Ser–His–Leu–Lys–Ser– Val–Asp–Gln–Arg– 1 1 Leu– Gln–Arg–Phe–Val–Thr–Asp–Val–Asn–Ser–His–Leu–Lys–Ser–Val–Asp–Gln–Arg– D R H F S V V R S L L Q V T K Q N D 11 Covalently Attached Lipids Anchor or Direct Some Membrane Proteins Proteins can be anchored to the membrane via long chain fatty acids, isoprenoids, sterols, or glycosylated derivatives of phosphatidylinositol (GPI). To anchor proteins more tightly to the membrane, most proteins use more than one lipid anchor and some use other interactions such as ionic interactions between positively charged amino acids and negatively charged phospholipid head groups. 151- KKKKKRFSFKKSFKLSGFSFKKNKK-175 (The clusters of K and R provide an anchor) GPI-anchored proteins are found in the outer leaflets of membrane rafts Attachment of lipids to proteins sometimes have a more specific role. GPI-anchored lipids are exclusively found on the outer leaflets while farnesyl or geranyl-geranyl linked proteins (as well as palmitoylated) are found exclusively on the inner leaflet. Attaching lipids to a newly synthesized protein therefore targets the protein to a specific location. For some lipid linked proteins, the lipid attachment process is reversible – Amphitropic Proteins b-strands The b-strand conformation is close to fully extended There are no hydrogen bonds within a given b-strand Instead, hydrogen bonds occur between adjacent strands of a sheet b-strands therefore never occur singly, but always as part of a sheet Each residue in a b-strand extends 3.5 Å Anti-parallel b-sheet Hydrogen bonds at right angle to the strands Side chains alternate in pointing up & down Side chains (purple) line up along ridges Only anti-parallel b-strands used to span membrane b-barrels – the alternative architecture for membrane proteins b-barrels are extended anti-parallel b-sheets that wrap around so that the last strand hydrogen bonds with the first b-barrel proteins have no unpaired hydrogen bonding main chain groups - therefore can be used as membrane proteins these are found as such in bacterial outer membranes and mitochondria β-barrel proteins (bacterial outer membranes (OMP) and mitochondria) ▪ About 8 residues will span the bilayer as β-strand ▪ 8 or more β-strands must exist so sheet can bend around to close and form a cylindrical β-barrel ▪ Amino acid side chains project alternately into and out of the barrel ▪ Inward for hydrophilic; outward for hydrophobic Strand properties Strands are anti-parallel, organized from N-to Cterminus in a simple circle b-strands generally sit at a 45° angle to the membrane All b-barrel membrane proteins have an even number of b-strands, reflecting the requirement that they be inserted pairwise One known exception – the mitochondrial protein VDAC Amino acid sequences of amphipathic beta strands alternate in polarity Amino acids in the membrane spanning section alternate between hydrophobic and hydrophilic residues Since side chains in a beta strand project alternately on either side, all hydrophobic residues fall on one side and the hydrophilic residues fall on the other side of the beta strand. The hydrophobic side face the exterior and the hydrophilic side face the interior of the barrel. Interior residues Bilayer facing residues Loop residues Wimley, Curr Opin Struct Biol 2003 General features of OMP structures (e.g. porin) Antiparallel β sheet Even number of strands Strands do not cross each other Extracellular loops are often long Periplasmic loops are very short, often β-turns N and C-termini always periplasmic and next to each other Channel size and contents OmpX – 8 strands OmpF – 18 strands FepA – 22 strands Examples with 8 – 26 strands are known Barrels with 8-10 strands have a solid core filled with hydrophilic residues (e.g. OmpX), and no channel Intermediate sized b-barrels with 16 – 18 strands have aqueous pores, generally with inserted loops (e.g. OmpF) Large barrels with 22–26 b-strands generally have a plug domain (orange) that inserts into and closes (most) of the channel VDAC – voltage-dependent anion channel Most abundant OM protein in eukaryotic mitochondria Barrel made up of an odd number of beta strands b1 parallel to b19 – only known case Acts as an ion channel; also plays a role in apoptosis Helix at N-terminus inserts into pore Monika Bayrhuber et al. PNAS 2008;105:15370-15375 Membrane Proteins have a Domain Structure hydrophilic external and internal domains hydrophobic transmembrane domain polypeptide chain folds independently in these regions There are some generalizations regarding amino acid position Charged residues (Lys, Arg, Glu, Asp) are found almost exclusively in the aqueous phases Hydrophobic residues occur frequently in the hydrophobic interior Positive-inside rule: Lys, His, Arg occur more commonly on the cytoplasmic face Trp and Tyr residues often found at the interface between the lipid and water for both types of proteins – Able to interact simultaneous with the lipid and the water phases Rotational symmetry in membrane proteins If the structure is identical after rotating around a fixed point, it has rotational symmetry Membrane proteins are normally inserted into the membrane in only one orientation (there are exceptions to this) Therefore, rotational symmetry axes are usually orthogonal to the plane of the membrane Oligomeric membrane proteins tend to be organized in rings Dual topology proteins Individual proteins are placed within the membrane in both orientations – these types of proteins are rare These can dimerize with the 2-fold axis in the plane of the membrane C C C N N N EmrE – 2B5D N C Fluoride channel – 5A41 Pseudosymmetry Pseudo-symmetry refers to use of two or more structurally similar chains with distinct sequences within a given oligomer (e.g., Acetylcholine receptor is built from 5 chains, 4 different subunit types) Many membrane proteins are built as head to tail fusions of two copies of the same chain–likely due to evolutionary gene duplication and fusion (seen with many membrane proteins) Repeats remain approximately symmetric e.g. Lac permease Quasi-symmetry b a a b b a A proteins could have multiple copies of the same protein with each subunit adopting a different conformation This is known as Quasi-symmetry e.g., The F1 component of the ATP synthase enzyme has 3 β subunit with each adopting a different conformation at a given time (loose, open and tight conformations). The 20 naturally occurring amino acids Nonpolar, aliphatic R groups The hydrophobic effect stabilizes protein structure Aromatic R Groups – Can contributes to hydrophobic effect Polar, Uncharged R Groups – R groups can form H bonds. Cys can form disulfide bonds Negatively Charged R Groups Positively Charged R Groups Cys has a positive hydropathy index. However, the textbook classifies it as polar due to its SH group’s ability to act as a weak acid and be involved in weak hydrogen bonds. We’ve classified it as moderately non-polar before (BIOC*2580)