Lecture 2: Structural Organization of Membrane Proteins II PDF
Document Details
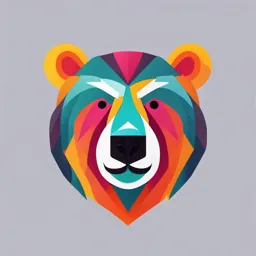
Uploaded by YouthfulGothicArt
Tags
Related
Summary
This document provides a lecture on the structural organization of membrane proteins. It covers topics like alpha-helices, transmembrane proteins, and how different amino acid types are involved in protein interaction with the cell membrane. It also details how protein structure impacts membrane thickness.
Full Transcript
The Helix helix = simplest arrangement, maximum number of hydrogen bonds ▪ backbone winds around an imaginary longitudinal axis ▪ R groups extend out from the backbone ▪ 3.6 residues per each turn; ∼5.4 Å ▪ C=O of each amino acid H-bonds with NH of the amino acid 4 residues down from it (C=O of...
The Helix helix = simplest arrangement, maximum number of hydrogen bonds ▪ backbone winds around an imaginary longitudinal axis ▪ R groups extend out from the backbone ▪ 3.6 residues per each turn; ∼5.4 Å ▪ C=O of each amino acid H-bonds with NH of the amino acid 4 residues down from it (C=O of #1 with NH of # 5; C=O of #2 with NH of # 6 etc.,) What is the length of an -helix that consists of 20 amino acids? A. B. C. D. 72 Å 30 Å 15 Å 20 Å 30 Å is the depth of a regular biological membrane The atoms in the centre of an -helix are in van der Waals distance from each other The helix is NOT hollow and there is no hole in the centre It is very tightly packed – the atoms in the centre are in van der Waals distance from each other Solutes cannot be transported through a helix. There is NO hole in the centre like shown here The middle is very tightly packed Helix dipoles In an -helix, the peptide bonds all point with the carbonyl groups towards the C-terminus Since each peptide bond has a dipole moment, these reinforce one another The result is a large net dipole moment with the dcharge at the C-terminus, and the d+ at the N-terminus Negatively charged amino acids are often found near the N terminus positively charged amino acids often found near the C terminus In a chloride channel, an α helix is found to be part of the specificity filter. Which part of the helix is likely to interact with the chloride ion? A. C terminal end B. N terminal end C. The mid part of the helix which is embedded in the membrane D. Either C or N terminus The Bacterial K+ channel The δ- of the helix dipole (the C terminal end of the helix) stabilizes K+ ions and act as a specificity filter. Single spanning helix The simplest integral membrane proteins use a single -helix to span the membrane. N- and C-termini are outside of the membrane, and often form extended soluble domains Since each amino acid is 1.5 Å apart from each other, ~ 20-25 amino acids can span a biological membrane that is ~30 Å thick Tilting the helix would require more residues Human Erythrocyte Glycophorin Single Spanning Helix - Side Chains The section of helix traversing the membrane is made up of hydrophobic amino acids (e.g., residues 75-93 in glycophorin.) Weakly hydrophilic residues tolerated in small numbers (e.g. Thr, Ser) Regions that interact with head groups of the phospholipids have more hydrophilic residues Aromatics (esp. Trp and Tyr) favoured in this interfacial region Human Erythrocyte Glycophorin Multi-spanning Trans-membrane proteins Multi-spanning TM proteins have 2 or more TM helices 4, 7 (GPCRs) and 12 (transporters) TM segment proteins are especially abundant (more than 13 is rare) The central region of the protein spanning the membrane is almost exclusively hydrophobic Aromatic residues (esp. Tyr and Trp) are concentrated at the solution/membrane interface Bacteriorhodpsin (7 TM) Lac permease (12 TM) Residue abundances in TM helices Small to midsized hydrophobic are most abundant (L/I/A/F/G/V together ~60%) S and T (small, weakly hydrophilic) are 7% each Other hydrophilic residues are rare (< 3%) Charged residues are all very rare (~1%) Liu et al Genome Biol. 2002 TMD of multipass membrane proteins can contain hydrophilic amino acids Hydrophilic side chains can be hidden from the lipid bilayer by having them face the interior of the bundle and interact with hydrophilic groups from the other strands. TMDs involved in transport, ligand binding, catalysis often contain polar or charged amino acids. Predicted energy of membrane insertion The β adrenergic receptor shown in its linear and folded states (Hegde & Keenan, Nature Reviews, 2022) The TMD of the glucose transporter has a channel lined with polar residues In the structure shown, the polar amino acids (coloured blue) of each helix line a hydrophilic transmembrane pore through which glucose in transported The hydrophobic side (coloured yellow) of each helix interacts with the membrane stabilizing the structure of the protein. Lehninger 8e Figure 11.32, Page 388 Lipid annuli (boundary lipids) are common in membrane proteins Annular lipids – form a bilayer shell (annulus) around the protein Oriented similar to the arrangement of the bilayer Head groups -interact with polar amino acids at the inner and outer membranewater interfaces Tails– interact with the non- polar residues This well-ordered lipid layer is analogous to the layer of well-ordered water molecules binding the surface of soluble proteins Specific lipids are often integral to the structure and function of the protein Proteins locally modify membrane thickness Mitra, PNAS The thickness of the membrane depends on the proteins embedded Proteins with a wide hydrophobic band will repack lipids so that the hydrophobic side chains are covered by lipid tails Proteins with a narrow hydrophobic band will encourage lipids to take on less extended conformations Single TM proteins, however, will tilt to accommodate the preferred default membrane thickness Tyr and Trp are found at the interface between lipid and water in many TM proteins The side chains of Tyr and Trp have both hydrophilic and hydrophobic character can interact with the hydrophobic lipids of the membrane and the aqueous phase on either side of the membrane at the same time Serve as membrane interface anchors Charged residues (Arg, Lys, Glu, Asp) are found almost exclusively in the aqueous phase Positively charged Lys and Arg residues tend to be concentrated on the cytoplasmic side of the membrane- Positive Inside Rule Summary of Secondary Structure of Membrane Spanning Segments All known structures exist as either one or more α-helices or as βbarrels α-helical proteins: – About 18-20 residues are needed to span the membrane – Bitopic - single α -helix, all amino acids nonpolar/hydrophobic – (Monotopic does NOT span the membrane) – Polytopic - α -helices arranged in a bundle – amino acids hydrophobic on average, but may include charged/polar residues Membrane helices in multi-spanning membrane proteins are often irregular Helices in multi-spanning membrane proteins are often not completely straight They often bend gently They incorporate irregularities including: – Over-wound regions – Under-wound regions – Kinks (often around proline residues) – Regions of local unfolding These irregularities help helices interact with one another over more of their length, or fulfill specific functional roles Common Secondary Structures Have Characteristic Dihedral Angles dihedral angles φ (phi) and ψ (psi) associated with each residue A dihedral angle: Angle at the completely described secondary structure intersection of two planes. The planes are defined by bond vectors in the backbone. 3 successive bond vectors with the central vector common to both describe two planes Ramachandran plots: – visualize all φ and ψ angles – test quality of three-dimensional protein structures Secondary Structure Conformations are Defined by φ and ψ Values Dark Blue: Conformations with no steric overlap - α helices and β sheets (allowed completely) Medium Blue: Atoms are closer together. Slight clash Light Blue: conformations allowed less frequently White: Conformations not allowed due to steric clashes Over wound 310 helices fall near the top right-hand corner of the α helical region (medium blue)– So, at the edge of the allowed regions and therefore less stable 310 Helix