Lecture 4: CBF & Pathophysiology of Stroke PDF
Document Details
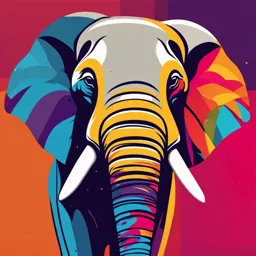
Uploaded by UnabashedLogic
Tags
Summary
This document discusses cerebral blood flow (CBF) and the pathophysiology of stroke. It covers topics such as cerebral metabolic rate of oxygen (CMRO2), blood flow to the brain, and energy metabolism in the brain.
Full Transcript
Lecture 4: CBF & pathophysiology of stroke Cerebral blood supply: ● Cerebral metabolic rate of O₂ (CMRO2) 55ml/kg/min (40-55%) → O₂ that is extracted from the blood ● Minimal CMRO2 for the function 33 ml/kg/min ● Below 22 ml/kg/min integrity of the neurons is affected → can lead to stroke ○ If energ...
Lecture 4: CBF & pathophysiology of stroke Cerebral blood supply: ● Cerebral metabolic rate of O₂ (CMRO2) 55ml/kg/min (40-55%) → O₂ that is extracted from the blood ● Minimal CMRO2 for the function 33 ml/kg/min ● Below 22 ml/kg/min integrity of the neurons is affected → can lead to stroke ○ If energy < 33 → function is affected → dizziness, concentration & memory problems ○ If energy < 22 → structural & cell death → stroke ● BF to the brain is: ○ 60% functional → normal electrical activity (measured by EEG) ○ 40% integrity → to keep the neurons alive “maintenance” ■ Even while sleeping 40% of oxygen is still being used to ← keep the brain alive ■ The neurons are suppressed here, not dead ○ Hypoperfusion of brain → functional problems will appear first ● The difference between the brain & high energy demanding tissues: ○ brain is in the skull, like closed box → ↑ in volume → brain presses on the skull ○ other tissues have high demand (more/less homogenous) ■ ex: If the heart ↑ metabolism → then it will ↑ throughout the cardiac muscles (not one segment of the heart) ○ in the brain it is totally different → ↑ level is required but it then depends on the activity of the selected region of to cortex, this area can demand more blood than other areas ○ Brain is 1.5 Kg, the average is about 500ml, so: ■ BF= 1.5 × 500ml = 750 mL to 1L goes to the brain ○ Content of O₂ in blood ~200 ml & tissue absorbs 25% ○ But when we consider 1.5 go thro the brain = then it is ↑, 1 L of blood go to 1.5 kg in the brain → O2 extraction will not 55 ml/kg/min ■ O₂ absorption will be ~ 40 to 50% of the blood instead of 25% (> 55ml/kg/min) ○ all of this depends whether it is in the cortex (gray m.) or white matter ○ If 30-40 HR → it requires 40 ml/kg/min of blood ○ If it goes to 70-80 HR → it requires 50-60 ml/kg/min, which is very similar to the avg of brain ○ But if it goes to 120-140 HR → the demand will go below 100 ml/Kg/min ○ different regions in the brain require diff. BF & diff. Demand Brain depends on a constant supply of O2 & sugars. The ↑ O2 consumption is to provide energy that is used to reverse the ion influxes that underlie synaptic potentials and AP: ● Energy metabolism in the brain. Dotted lines indicate rxns that occur during ischemia. The dotted line across the oxidative phosphorylation rxn indicates that this reaction is blocked during ischemia. ● This figure shows energy supply, usage & BF regulation in the brain: ATP is generated from glycolysis & mtc OP in neurons & glia. ATP is mainly consumed (red arrows) by ion pumping in neurons, to maintain the ion gradients underlying synaptic & AP, following Na+ entry thro iGluR & voltage-gated Na+ channels (NaV) ● It is also used in glia for Na+-coupled nt uptake by excitatory amino acid transporters (EAAT) & for metabolic processing (shown for conversion of glutamate to glutamine) & on maintaining the cells’ resting potentials ● If not enough energy (ATP) → can’t pump properly, ↑ glutamate accumulates in ECF = ↑ Ca+2 con. in a cell = triggers apoptosis ● Why FFA cannot be used by brain? Cannot cross BBB, so what does the brain use? glucose ● Glucose → glycolysis → pyruvic acid → enters creb cycle when o2 present ● What if no o2? Pyruvate convert to lactate → produces 2 ATP in contrast to 38 ATP from creb ● Why does the brain need alot of ATP? ○ Neurons: for the pumps to maintain membrane potential ○ Astrocytes: u need to know first that astrocytes remove glutamate from ISF by bringing it along with Na (2nd transporter). So we need to maintain low Na inside astrocytes, to do this we need a pump to always pump Na outside the astrocyte, thats why we need ATP The astrocyte-neuron lactate shuttle (ANLS) model: ● Lactate exits astrocyte via monocarboxylic acid transporter 1 & enter the neuron via monocarboxylic acid transporter 2 ● In the astrocyte glutamate is converted to glutamine and transported to be resynthesized (recycling process) Unique features of the cerebral vasculature: ● The large cerebral arteries contribute significantly to the CVR & therefore contribute to the regulation of CBF ● The cells making up the vessels interact with other cell types including neurons, astrocytes, pericytes, & glial cells (neurovascular unit - NVU) ● Regulation of regional BF is feed-forward, rather than feed-back. ● What is the aim of having different types of vessels? ○ To maintain flow & pressure regardless of changes in MAP ● In a normal physiological state, total BF to the brain is remarkably constant due in part to contribution of large arteries to vascular resistance. In addition, parenchymal arterioles have considerable basal tone & also contribute significantly to VR in the brain. The ↑ ● ● ● ● ● metabolic demand of neuronal tissue requires tight coordination between neuronal activity & BF within the brain parenchyma, known as functional hyperemia. However, in order for flow to ↑ to areas within the brain that demand it, upstream vessels must dilate in order to avoid reductions in downstream microvascular pressure. Therefore, coordinated flow responses occur in the brain, likely due to conducted or flow-mediated vasodilation from distal to proximal arterial segments & to myogenic mechanisms that ↑ flow in response to ↓ pressure Pial artery is controlled by → PNS → SNS ○ Plays a major role in autoregulation ○ have perivascular nerve ○ Have ↑ SMC which regulates BF ○ ↑ activity of cortex → vasodilation of nearby vessels → goes to pial artery → ↑ CBF Intra-cerebral arteriole have ○ Intrinsic neuron (get innervated from neurons in brain) ○ Tiny SMC layer ○ Glial end foot Capillary have ○ Intrinsic neuron ○ Pericyte (innervated from local neuron) ○ Glial end foot Penetrating arteries controlled by CNS Arterioles and small branches controlled by → local neurons Functional features of the cerebral circulation: ● Penetrating arterioles act as a bottleneck to protect the smaller capillaries, how? ○ Unlike the surface arteries & pial arterioles, the penetrating arterioles have very few branches so the R in them is high, this high R buffer the P changes between the brain & the body, so if BP increases suddenly the brain won't be affected. ○ If increased BP we want to dilate the vessels, & opposite is true ○ Thus, they control the BF to very discrete regions of the cortex & act as a bottleneck to BF between the surface of the brain & capillary bed. The parenchymal arterioles are located in the parenchyma surrounded by astrocytic end feet. ● Pial arteries receive extrinsic innervation from the PNS. ● What's the aim of innervation? Match resistance of the BV to perfusion pressure ○ If low BP → SNS detects it → tells pial artery to dilate ○ If high BP → pial artery to constrict ○ SCG= superior cervical ganglion decreases vessel tone = dilate vessel ○ SPG = sphenopalatine increases vessel tone = constrict vessel = more R ○ TG = trigeminal increase vessel tone = constrict vessel = more R ○ Contrary to that, arteries within the brain parenchyma, or the microcirculation, receive intrinsic inniveration; these nerves originate in the CNS. Cortical microvessels receive norepinephrine, serotonin, ach, or GABAergic afferents from subcortical neurons from the locus coeruleus, raphe nucleus, basal forebrain, or local cortical interneurons. ● Inset: neurovascular unit: set of cells that are used to match perfusion (vascular) to neuronal activity ○ It involves astrocytes, neurons, vascular smooth muscle cells, endothelial cells and others ● SNS = in the superior cervical ganglion → 𝝰 receptors get activated → vasoconstriction = ↑ BP = pial arterioles constricts to prevent BP ↑ ○ ex: If you're scared, activated SNS → ↑ BP but to protect the brain → pial arterioles constrict ● PNS = causes dilation by parasympathetic ganglion or otic → migraine/ headache = dilation of pial arterioles causing distention of meninges → pain ● If neurons get activated = release NT = causing atrial dilation = reducing resistance = increasing blood that comes from the upper bigger arterioles to them The relations between the intracranial pressure (ICP), MAP & cerebral perfusion pressure (CPP): ● CPP = MAP – ICP *normally ICP is close to 0, so CPP = MAP ● CBF= CPP/CVR ● CBF= (MAP-ICP)/CVR ● CPP ≈ MAP ● CPP ≥80 mmHg, If ≤ 50 mmHg - ischemia & ↓ electrical activity of the brain ● 1st: CPP increases, so CBF increases ● 2nd: Since we want to keep the CBF constant, as CPP increases CVR increase to maintain CBF ● 3rd: The ↑ of CVR is a result of decrease in diameter ● 4th: As a result of the changes mentioned in the previous steps the CBF is maintained → autoregulation The autoregulation: CBF in relation to artery lumen diameter: ● Dotted lines represent the lower and upper limits of CBF autoregulation. Red circles represent the cerebral arteries, and blue line represent the CBF ● ● ● Four mechanisms are proposed for autoregulation: ○ Myogenic: concerns the ability of the vascular smooth muscle to constrict or dilate in response to changes in transmural pressure. It is a feature of any smooth muscle. ○ Neurogenic: occurs thro an extensive nerve supply to cerebral vessels. ex: activation of 𝝰 adrenoceptors shifts the limits of autoregulation to higher pressures (to right) & cerebral vessels respond to this with vasoconstriction ○ Metabolic: chiefly contributes to autoregulation in the microvasculature, where changes in the microenvironment such as for pCO2 & H+ will lead to vasodilation. Imp for parenchymal tissues ○ Endothelial: factors such as NO In fact, a theory suggests that a neuronal control of CBF serves to maintain autoregulation against MAP However, if autoregulation fails in traumatic brain injury, in stroke (mainly in the penumbra & in the tissue affected by stroke, around space occupying lesions; in that case CBF begins to change linearly with MAP The autoregulation: effects of the SNS & α receptors in VSMCs: neuronal control: ● We said that when SNS is activated → pial a. constrict so CBF stays constant ● SNS blocked? P will ↑ at earlier stages bcs no constriction so ↑ CBF → curve shift to left → ↓ lower limit of autoregulation ● SNS stimulated? P will ↓ at later stages bcs constriction so ↓ CBF → curve shift to right → ↑ upper limit of autoregulation ● CAH affects autoregulation, bcz the upper & lower limits of autoregulation are both displaced to higher levels in pts with chronic HTN. ○ So, symptoms of cerebral hypoperfusion can occur at higher MAPs → curve shift to right ● The sympathetic innervation of the cerebral vasculature raises the upper limit of autoregulation & interfering with it, either by pharmacological blockade (alpha blocker) or by cutting nerves, ↓ the lower limit, but in the normal range of pressures neither stimulation nor blockade affects CBF. ● BB have no effect on autoregulation of CBF (past mcq) ● RECAP: ○ Stimulate sympathetic system = shift to right ○ Block sympathetic system (alpha blockers) = shift to left ○ In CAH, the vessels will adjust & the curve shifts to the right. Due to this adjustment, if you ↓ the pressure suddenly (to levels that can be handled by normal patients) they will experience symptoms of hypoperfusion. (So they're less adaptable to lower pressures) Constituents within the skull: slide 12: ● The total of 1600ml ○ brain (80%) → Can increase with edema ○ blood (12%) ○ CSF (8%) → acts as a buffer ■ plays major role in preventing ICP ↑ when CBV or brain volume ↑ → in systemic hypotension ● The skull is thus a rigid fluid filled box ● If the volume thus ↑, the pressure inside will rise considerably unless some fluid is able to escape ○ ↑ in ICV bcz of ex: dilation of bv, mild ischemia, & cytotoxic edema ● If the brain enlarges, some blood or CSF must escape to avoid a rise in pressure ○ So, when you ↑ ICV, the ICP doesn't ↑ initially ○ Bcz CSF will escape → leaves the skull to provide more space for the brain ○ This buffering effect occurs until a certain point, after all the CSF leaves ● Then if this fails, or be unable to occur there will be a rapid ↑ in ICP from the normal range (8-18 mmHg) ○ The ICV will lead to a direct ↑ in ICP ● If the ICP ↑ it may cause multiple things: ○ ↓ CBF ○ Hernia, which may affect the brain stem & causes sudden death ● Vicious cycle of the vasodilation cascade: ○ In hypoperfusion (MI or shock) → ↓ CPP (CPP = MAP - ICP) → arteries dilate to compensate → ↑ CBV → ↑ ICP → & further ↓ CPP → person dies ○ How do you stop this cycle? Stop its cause, which is shock → ↑ SABP (Systemic arterial BP) CBF is regionally heterogeneous; These differences are due to local neuronal activity. Two mechanisms: feedback & feedforward: ● Total CBF is 750 - 1000 ml/min: ○ 350ml/min from each ICA ○ 100-200ml/min from the vertebral artery ● CBF: ○ If you look at the brain holistically, it is constant & homogenous ○ Local regions → heterogeneous & dependent on the activity of the neurons ○ Ex: when you look at a visually stimulating thing the optic cortex would promptly increase ?? Feedback control of CBF: ● BF depends on metabolism: ↓ O₂ & nutrition → ↑ CO₂ & H → vasodilation → ↑ BF to area ● Disadvantage: in imminent danger, you wouldn't want to black out as your visual cortex gets BF back → we want out neurons fully functional at ALL TIMES ● Vasodilators ex: ○ pO2 pCO2, Adenosine ● ● Feedforward control of CBF: ● ● ● ● Release NTs to neurons & astrocytes → vasodilation As soon as glutamate (main NT) is release → vasodilation Vasodilators release from astrocytes: K+, AA, PG, EET Vasodilators release from neurons: NO Normal conditions: CBF is regulated by a feedforward mechanism which is when the axons stimulate astrocytes & neurons to release vasodilators BEFORE changes in blood lvls of O₂, CO₂ or other metabolites Pathological conditions: regulated by a feedback mechanism which occurs AFTER the ↑ O₂, CO₂ or other metabolites Feed forward control: ● Blood is redirected to area in need depending on the activity of the brain & it isn’t equal but regional flow depends on neuronal activity ● ↑ activity at the site of termination of thalamic afferents (layer 4) produces vasodilation in nearby vessels (local vasodilation) that then propagates upstream to pial arteries, ↓ resistance vessels that control neocortical BF (remote vasodilation) ● Pial arterial dilation ↑ CBF in downstream branches, including those that supply the activated territory ● Myogenic adjustments of vessels that supply quiescent areas & activity of interneurons, help to focus the ↑ in flow to activated areas ● The interneuron & the thalamic afferent neurons help in activation of specific areas (by causing vasodilation). ○ In neurons, synaptically released glutamate acts on N-methyl-D-aspartate receptors (NMDAR) to ↑ Ca+2i, → neuronal NO synthase (nNOS) to release NO → activates SM guanylate cyclase. This generates cGMP to dilate vessels. ↑ Ca+2i may also generate arachidonic acid (AA) from phospholipase A2, which is converted by COX2 to prostaglandins (PG) that dilate vessels. ○ Glutamate ↑ Ca+2i in astrocytes by activating metabolic glutamate receptors (mGluR), generating AA & 3 types of metabolite: PG & EETs in astrocytes, which dilate vessels; 20-HETE in smooth muscles, which constricts vessels. A ↑ Ca+2i in astrocyte end feet may release K+, which also dilates vessels. Summary of proposed regulation of cortical microvessels by pyramidal cells, GABA interneurons & astrocytes: ● Subcortical afferents target distinct populations of neurons in cortex, either: ● Directly acting on local microvessels, which are endowed with receptors (geometric forms on the vessel wall) for most nts/neuromediators ● Indirectly via astrocytes that act as intermediaries to both pyramidal cells & interneurons. Known direct vasoactive mediators released from pyramidal cells & interneurons are prostaglandin E2 (PGE2) & NO & GABA, whereas astrocytes act by releasing EETs Feedback mechanism: PO2 (slide 16) ● CBF is virtually insensitive to ↓ of arterial O2 until PaO2 falls to very ↓ levels → CBF changes “hyperbolic” ○ drops < 70/80mmHg → normal O2 pressure = 100mmHg ● CBF changes in response to CO2 (PH) is much more powerful ● No direct relationship between PCO2 and CBF ● PCO2 change → pH change → changes in CBF (pH is the middle man between PCO2 & CBF) ● pH & CBF do have a linear relationship Effect of hyper & hypocapnia on cerebral autoregulation: ● Hypercapnia: autoregulation curves are in black at normocapnia & red at hypercapnia. Cerebral resistance vessels are illustrated in red/pink. The bold solid blue arrows indicate the dynamic shift of the maximally dilated & constricted cerebral resistance vessels at hypercapnia. The dashed black and blue lines/arrows indicate the lower and upper limits at normocapnia and hypercapnia, respectively. ○ Vasodilation: In severe hypercapnia the autoregulation range would be decreased bcs the smc of the arteries are already dilated regardless of sympathetic stimulation ● Hypocapnia: Caliber of maximally constricted cerebral resistance vessels at hypocapnia is smaller than normocapnia & hypocapnia causes a rightward shift of the upper limit. Autoregulation curves are in black at normocapnia and blue at hypocapnia. Cerebral resistance vessels are illustrated in red/pink. The bold solid blue arrow indicates the dynamic shift of the maximally constricted cerebral resistance vessels at hypocapnia. The dashed black & blue lines/arrows indicate the lower & upper limits at normocapnia & hypocapnia, respectively ○ Vasoconstriction: In severe hypocapnia, autoregulation range would be increased bcs smc of the arteries are already constricted CFB during exercise: ● Changes in CBF is similar to changes in CO2, which suggests that CO2 has a great effect on CBF ● Cardiac output has nothing to do with CBF ● There is some correlation between oxygen extraction fraction and Cerebral metabolic rate of oxygen Hypoperfusion (ex:stroke): (Slide 20 & 21) ● CBF & Metabolic Thresholds. ● With ↓ CPP as occurs distal to a cerebral artery occlusion, intracranial arteries dilate to maintain CBF → autoregulation ● This results in an ↑ CBV ● When vasodilation is maximal, further ↓ CPP → ↓CBF → ↑ in O₂ Extraction Fraction (OEF) to maintain tissue oxygenation ● When OEF is maximal, further ↓ CPP→ ↓ CMRO2 utilization ● Pic: combined effects of residual CBF & duration of ischemia on reversibility of neuronal dysfunction during focal ischemia. ● The gray shaded region outlines the limits of severity & duration of ischemia, distinguishing tissue “not at risk” from functionally impaired tissue ● True or false: As soon as CBF reduces to below 20ml/100g, the function is affected irreversibly ○ Ans: false, time is a factor! When the CBF goes < 20 & stays there for a time the reduction in function is irreversible ● If the tissue got damaged, the OEF will remain low even if BF is restored :) The transient ischemic attack (TIA): ● Brief episode of neurological dysfunction caused by focal brain or retinal ischemia, with clinical symptoms typically lasting < 1 hr & without evidence of acute infarction ● Causes: ○ ↓ flow through a partially occluded vessel or stenosis of a small penetrating vessel ○ Atherosclerosis of carotid & vertebral arteries ○ Embolism: valvular disease, ventricular & atrial thrombi ○ Arterial dissection ○ Arteritis ○ Sympathomimetic drugs (eg, cocaine) ● Use the ABCD to rate the patient form 1-7 according to risk factors (not required to memorize the table) ● TIA's by themselves aren't an issue, we can use them to estimate if a patient is likely to have a stroke ● If patients ABCD stroke is 7, then they 25% chance of having a stroke within 90 days Immediate diffusion-weighted imaging assessed with MRI is the current preferred test for pts with a suspected TIA: ● If the TIA occurs in the same place many times it may cause vascular dementia ● Multiple bright spots in the cortical territory of right MCA. ● Panel A shows 2 small brain infarctions, Panel B shows 1 small infarct & Panel C shows one small infarct (arrow) Three main types of Ischemic Stroke: ● Thrombosis: ○ Atherosclerosis ○ Other pathological causes ● Embolism: ○ Two most common sources of emboli: ■ Left sided cardiac chambers ■ Artery to artery stroke ○ Generally “smaller” strokes than thrombotic strokes ● Global-Ischemic or Hypotensive Stroke: ○ Any event causing abrupt drop in BP ○ Sites affected by low CBF are located at the end of an arterial territory ○ Watershed area ○ Multiple small occlusions BV occlusion triggers an acute injury phase with excitotoxicity, the failure of ionic homeostasis, cytotoxic edema & free radical formation: ● Our aim is to recover the patients in 4 hours & do thrombectomy to prevent death MINUTES ● Lesion is deprived of O2 and necrosis occurs, the Penumbra is the tissue at risk ○ Energy failure ○ Excitotoxicity ○ Ca & Na overload ○ Cytotoxic edema ○ Free radicals ○ Early BBB injury ○ PARP activation HRS TO DAYS ● Penumbra decreases as the lesion increases ● Apoptosis & neuroinflammation ○ Caspase activation ○ ↑ cytokine & chemokine lvl ○ Leukocyte infiltration ○ Microglial activation ○ ↑ROS & RNS lvls ○ ↑ PG & Leukotriene production ○ MMP activation & vasogenic edema DAYS TO WEEKS ● Penumbra = lesion ● Neuroplasticity ● Angiogenesis & neurogenesis ○ ↑ NSC proliferation, migration & differentiation Intracranial hemorrhage: ● Features of intracerebral hemorrhage: ○ BF to an area is lost (same as ischemic stroke) ○ ↑ ICP → hemorrhage, which are fatal ○ Pronounced toxicity to cells Why? ○ NORMAL: ■ RBC & Hb are taken by macrophages & digested so the heme & iron don't cause toxicity ■ Hemopexin also binds to heme to protect the body from toxicity ■ Hemosiderin also binds iron for protection. ○ HEMORRHAGE ■ ↑ RBC conc, the mc can't keep up → iron & heme build up → cause damage ● Types: → type the adjacent pic to where it belongs ○ Intracerebral hemorrhage (ICH) ■ Classification & frequency based on hematoma location ● Deep (basal ganglia & internal capsule) 35% to 70% ● Lobar (cortical–subcortical areas) 15% to 30% ● Cerebellum, 5% to 10% ● Brain stem, 5% to 10% ○ Subdural hematoma ○ Epidural bleeds ○ Subarachnoid hemorrhage (SAH) Molecular Pathophysiology of Cerebral Hemorrhage: ● Upper image: ○ During ICH, blood is released into the brain matter ○ RBC are cleared from parenchyma by microglia/mc through cell-surface scavenger receptor CD36-mediated phagocytosis ○ Timely clearance of RBC & injured cells prevents them from lyses & spillage of toxic contents into brain parenchyma ○ In hemolysis (occurs after ICH), Hb needs to be removed quickly from the ECS to avoid its cytotoxic effects ○ Haptoglobin (Hp) from blood & synthesized locally by oligodendroglia, binds Hb, forming less toxic Hb–Hp complexes that are endocytosed by microglia/ mc through scavenger receptor CD163 ○ Toxic extracellular free heme generated from Hb can be neutralized by binding to hemopexin ○ Heme–Hx complexes are subsequently removed by phagocytes via CD91 scavenger receptor-mediated endocytosis. ○ In phagocytes, heme is metabolized by heme oxygenase (HO-1) to biliverdin, CO & pro oxidative iron ○ To prevent oxidative cell damage, iron is sequestered within phagocyte by iron-binding proteins (hemosiderin or ferritin) ○ CD36 is expressed under control of PPAR & Hp expression is ↑ with Nrf2 ○ Both Nrf2 & PPAR ↑ expression of antioxidant proteins (ex: catalase or SOD) ● Lower image: ○ ICH activates TF NFB → perpetuates inflammation & oxidative stress → 2ndry brain damage ○ TF PPAR via inhibition of NF-B & induction of antioxidative defense components ↓ inflammation, oxidative stress & protects brain ○ TF Nrf2 acts as an effective regulator of oxidative stress & blood detoxification components ○ In addition, PPAR stimulates phagocytosis mediated hematoma cleanup, thus facilitating removal of hematoma, the source of toxicity & inflammation