Detection of X-rays PDF
Document Details
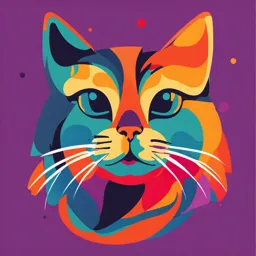
Uploaded by ProsperousLead
Fatima College of Health Sciences
Tags
Summary
This lecture provides a summary of X-ray detection mechanisms and different detector types, including ionization and scintillation detectors. The document also discusses learning outcomes, prescribed text, and key terms related to X-ray detection.
Full Transcript
Detection of X-rays Slide 1 fchs.ac.ae Learning Outcomes At the conclusion of this lecture, associated tutorial and practical session (if relevant), you will be able to: State the general principles of photon detection Provide examples of the main x-ray detector types Define...
Detection of X-rays Slide 1 fchs.ac.ae Learning Outcomes At the conclusion of this lecture, associated tutorial and practical session (if relevant), you will be able to: State the general principles of photon detection Provide examples of the main x-ray detector types Define detector efficiency List the various ionization-type detectors List various luminescence-type detector materials Describe the function of a scintillator Explain the purpose of a photomultiplier tube Slide 2 fchs.ac.ae Prescribed Text This lecture is not drawn specifically from Bushong. Much of this lecture is drawn from: Bushberg, J.T., Seibert, J.A. Leidholdt Jr, E.M. and Boone, J.M., The Essential Physics of Medical Imaging, 4th edition, Lippincott, Williams and Wilkins, Philadelphia, 2020, pp. 633-677. Images in this PPT lecture presentation are selected from this text unless otherwise noted. Slide 3 fchs.ac.ae Detection of x-ray Photons X-ray photons are detected when they impart all or part of their energy to the detector material Radiation energy is totally or partially absorbed by the detector material For ionizing electromagnetic radiation, x-rays and -rays, photon energy is E = hc/ = hf Where: E is photon energy (Joules), λ is the photon's wavelength (metres), c is the speed of light in vacuum - 299792458 metres per second h is the Planck constant - 6.62607015 × 10−34 (m2kgs−1) Slide 4 fchs.ac.ae Interaction Mechanisms The absorption of photon energy is by two mechanisms: Excitation Electrons may be excited, not only to higher energy states in atoms (as you have already studied) but also to higher energy states in molecules or in crystals Ionization Instead of being raised to an excited state, electrons may be completely removed from atoms or molecules, creating ions in the process Both of these processes may produce chemical changes or cause the emission of (usually) visible light or electrical charge induced in the circuit. Slide 5 fchs.ac.ae Amplification Because of the small magnitude of the photon energy, signal amplification is almost always required Methods of amplification vary, depending on the type of mechanism being used for photon detection, and can be electrical or chemical Various methods – for example, The photomultiplier tube The image intensifier These will be discussed briefly under each relevant method Slide 6 fchs.ac.ae Flux and Intensity Flux, F, is the number of photons per unit time per unit cross-sectional area, expressed as photons per second per cm2 Intensity, I, is power per unit area, units watt/cm2 but usually expressed as joule/s/cm2 Intensity is integrated (summed) across the energy spectrum; Because the counting area is usually constant, flux is proportional to count rate, and intensity is proportional to count rate times mean (average) photon energy If the mean beam energy remains constant, then intensity and flux are directly proportional Slide 7 fchs.ac.ae Main Detector Types The main possibilities are: Fluorescence: the incoming photon is absorbed and lower energy radiation is produced. This occurs in film screens and scintillator-type detectors. Energy trapping: as in computed radiography (CR) image plates, thermoluminescent dosimeters (TLD’s). Ionization chambers and related devices: like Geiger-Müller tubes and proportional counters Digital radiography systems electrical charge induced in the circuit. Slide 8 fchs.ac.ae Some Useful Definitions Luminescence – emission of light by a material; and can take many forms such as: Fluorescence – the emission of light by a material that has absorbed light or other form of electromagnetic radiation Phosphorescence – similar to fluorescence, but the light emission is delayed in time Thermoluminescence – the later emission of light is due to heating of the irradiated material Optically stimulated luminescence – the later emission of light following optically irradiating for previously irradiated material Chemiluminescence – light emission after chemical reaction Slide 9 fchs.ac.ae Some Useful Definitions The terms Phosphor and Scintillator are often used interchangeably to represent luminescent materials In radiography, phosphors and scintillators are used to (immediately) convert x-ray energy into light energy Ionization detectors: Geiger-Muller detectors (gas-filled detector) Proportional counters (gas-filled detector) LED-type (solid state; producing electron-hole pairs) These all convert photon energy into ion pairs. Slide 10 fchs.ac.ae Two Forms of Detector Systems 1. Detect each photon: Defined as a detection system operating in pulse-mode; Here each photon is detected individually Problem may be that some photons are ‘missed’ while the previous photon event is being ‘processed’, which Leads to the idea of ‘dead time’ 2. Detect the beam energy: Defined as a detection system operating in current-mode Output is a measure of the energy in the incident beam These systems have a time constant Output is integrated (or smoothed) over time Slide 11 fchs.ac.ae Detector Types Fluorescence detectors use a phosphor scintillator to convert x-ray photons to optical photons. For gamma spectroscopy, a common scintillator material is NaI. Different physical forms are possible for the scintillator, including deposition of the material onto a substrate. Slide 12 fchs.ac.ae Detector Characteristics All detectors have a characteristic curve which relates the detector output response to the input dose or exposure For any detector, a certain minimum input dose is required before you will get any output; this minimum input is called the ‘offset’ Above this threshold, a curve (that may or may not be linear) describes the relationship between input and output The H & D curve is nearly linear in the diagnostic range [H&D = “Hurter and Driffield”, study from 1890 of visible film characteristics, relevant to film radiography – see later lecture] Slide 13 fchs.ac.ae Characteristics If linear, which is very desirable, the characteristic curve will have a constant gradient, called the sensitivity sensitivity = output/input Film is not linear, but Modern computer-based and digital detection systems are linear, over a certain range For zero input, a minimum output level – the offset – will be detected Above a certain maximum input, there will be no appreciable increase in output; this is called saturation Noise will be present in any system Slide 14 fchs.ac.ae The latitude or dynamic range refers to the range of intensities to which the device responds saturation (usually 3 or more orders of magnitude) Dynamic range (or latitude) refers to the range of intensity levels or signal values that an imaging device can detect and accurately represent. This range determines how well the device can capture details in both the brightest and darkest areas of an image without saturating. For example, if the device's dynamic range covers 3 or more orders of magnitude, it can effectively display a wide range of brightness levels, providing clearer images with better contrast and detail across varied tissue densities. Slide 15 fchs.ac.ae fchs.ac.ae fchs.ac.ae 1-Digital Detectors In order to understand signal processing we need to learn about the detectors. Photo Stimulable Phosphor Plates Photoconductive materials. Detector consists of a receptor material (e.g. BaF(H)Eu), and a set of signal readout and conversion electronics. fchs.ac.ae Best described by three elements: Capture element: that in which the x-ray is captured. Coupling element: that which transfers the x-ray generated signal to the collection element. Collection element: photodiode, a charge-coupled device (CCD), or a thin-film transistor (TFT) fchs.ac.ae Direct systems convert X-rays into an electric charge by means of a photoconductor such as amorphous selenium. In indirect systems, X-rays are converted into visible light by means of an intermediate scintillator layer such as cesium iodide. The light photons are then converted to an electric charge by a photodiode. Indirect uses phosphor, direct uses a photoconductor. 20 fchs.ac.ae Slide 21 fchs.ac.ae Slide 22 fchs.ac.ae Slide 23 fchs.ac.ae Diode Detectors The photon energy (often light) is absorbed in the semi- conductor material, and Produces electron-hole pairs The circuit collects this charge directly or as a current Usually used in current mode Can be used in conjunction with phosphor to turn x-ray energy into light energy Slide 24 fchs.ac.ae 2-Ionization Chambers This is one type of gas-filled detector The energy of incoming photon ionizes gas atoms Heavy gases like Xe with higher may be used to increase the stopping power An older Hitachi CT scanner has a xenon detector array operating at a gas pressure of approximately 20 atmospheres There is a voltage between the plates of an ionization chamber; the electric field collects the electrons at the anode and cations (ionized atoms) at the cathode Slide 25 fchs.ac.ae Air Ionization Chambers An ionization chamber containing only air is used to measure incident radiation intensity in the form of exposure, E (C/kg), and this may also be converted to dose. The Diamentor is an example of an air ionizsation chamber. This is an example of a dose-area product meter, and this quantity (dose area) is independent of distance from the focal spot (assumed to be a point source of radiation) Ionization chambers work in current-mode, and can measure dose, or the dose-rate Slide 26 fchs.ac.ae Proportional Counters The (most useful) proportional region, P, follows the ionization region, I Charge collected is proportional to the applied voltage Amplification depends on electric field within the detector R = recombination region This instrument has a potential I = ionization region P = proportional region dead-time GM = Geiger-Muller region CD = continuous discharge region Bushong Figure 36-7, page 558 Slide 27 fchs.ac.ae Geiger-Müller (G-M) Counters These instruments have a significantly higher voltage across the tube than do ionization or proportional counters A special gas is used Most particles are detected The G-M counter is useful for general survey work such as detecting contamination after spillage of radioactive material Radiation must be able to enter the active detector volume, usually through a thin ‘window’ of low-Z material. For detecting alpha particles, the window must be very thin Slide 28 fchs.ac.ae The G-M Counter Can operate in pulse-mode Cannot be used as a spectrometer or a dose-rate meter G-M tubes are cheap to build as they require no electronic amplification The output charge pulse is often fed directly to a speaker or ammeter (current meter) G-M tubes need a large bias voltage The ‘dead time’ can be a shortcoming of the G-M detector May become ‘paralysed’ in high radiation fields, and read zero! Slide 29 fchs.ac.ae 3. Scintillation Detectors We will now consider detectors that utilise scintillation crystals as the detecting medium instead of gas. Scintillation crystals produce light when interacting with radiation and the number of light photons produced is proportional to the energy of the incident x-ray photon. Slide 30 fchs.ac.ae Scintillation Detectors (continued) Sodium iodide is the most common crystal scintillator material. It is usually mixed with a small amount of thallium to enhance the visible light output. This type of detector is denoted by NaI(Tl). The crystal must be optically coupled to a photomultiplier tube (PMT) as shown in Figure 6. The function of the PMT is to convert the light photons from the crystal into an electrical pulse. Slide 31 fchs.ac.ae Scintillation Detectors (continued) Figure 6. Scintillator crystal and photomultiplier tube combination (Bushberg Fig. 17-8). Slide 32 fchs.ac.ae Scintillation Detectors (continued) A scintillation detector operates in a series of steps. These are: 1. The incident radiation excites atoms in the scintillator 2. The excited atoms decay, emitting visible light 3. The light strikes the photocathode of the PMT, releasing electrons 4. The electrons are multiplied in the PMT to produce an output pulse, the height of which is proportional to energy. Slide 33 fchs.ac.ae Scintillation Detectors (continued) The typical operational range for PMTs is an applied voltage of 800 - 1200 V. The gain (or amplification) of a typical PMT can be up to 108. Increasing the voltage between the dynodes of a PMT will result in greater amplification, that is increasing gain. Increasing the voltage beyond an optimal range will result in unacceptably high amplification of noise. The PMT voltage must be highly stabilised to prevent fluctuations in amplification. Slide 34 fchs.ac.ae 4. Solid State (diode) Detectors Diode detectors are semiconductor devices usually made of germanium (Ge) or silicon (Si). Ge detectors must be doped with small amounts of lithium (Li) to enable them to operate. Their principal use is in applications where a high degree of energy resolution in a photon energy spectrum is required. The energy resolution of these detectors is about 8 times better than proportional counters and 20 to 80 times better than NaI(Tl) detectors Slide 35 fchs.ac.ae Solid State (diode) Detectors (continued) Operation The radiation ionises the semiconductor atoms in a manner similar to that in a gas detector. These ionisations produce a charge pulse that is converted to a voltage pulse by a resistor. Because the density of semiconductor materials is 2000 – 5000 times more than gases, they have much better stopping power and are much more efficient detectors for x-rays and γ-rays. Slide 36 fchs.ac.ae Solid State (diode) Detectors (continued) Each ionisation requires a lower energy than that required in gas detectors (about 3eV compared with 35eV). Thus, about 10 times the number of ions are produced relative to gas detectors for a given γ-ray energy. Slide 37 fchs.ac.ae 5. Thermoluminescence Detectors Certain crystalline materials are able to absorb x-ray energy when irradiated and to store it for a considerable time. We can determine the resulting radiation dose by measuring the light output from the material when it is heated through a specific temperature cycle. Such devices are called thermoluminescent dosimeters and are used for personal dose monitoring. You will be required to wear one as you undertake your nuclear medicine studies. Slide 38 fchs.ac.ae Thermoluminescence Detectors (continued) Common TLD compounds include LiF:Mg, CaF2:Dy and CaF2:Mn. The doping elements increase the sensitivity. The heating produces a characteristic glow curve for the thermoluminescent material and both the height of the highest temperature peak and the total area under the curve are directly proportional to the energy deposited in the TLD by ionising radiation. LiF has an atomic number similar to that of soft tissue and so its response is relatively independent of the energies encountered in medical applications of radiation. Slide 39 fchs.ac.ae Summary Check that you can satisfy the learning outcomes for this lecture Go over calculations/exercises undertaken during the lecture Make sure you can define/describe the following terms: Luminescence and related terms Pulse-mode and current-mode detector systems Detector threshold, linear region, and saturation Digital detectors Various types of ionization detectors Scintillator Photomultiplier tube Solid state (diode) detectors Thermoluminescence detectors Slide 40 fchs.ac.ae