Lec 18-21 Physics PDF
Document Details
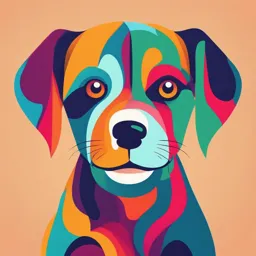
Uploaded by EasiestHarmony86
OneClass
Tags
Summary
These lecture notes cover Physics topics in relation to medical imaging, including location distortion, intentional techniques, factors affecting image quality, exposure, radiation detection, and measurement, as well as radiation detectors.
Full Transcript
Lec 18-21 Physics Location (Position) Distortion Location distortion: - The misrepresentation of an object's position on the image relative to other objects due to variations in the OID and the use of oblique X-ray beams How It Occurs: - Object at Larger OID- When object is located at a larger OID...
Lec 18-21 Physics Location (Position) Distortion Location distortion: - The misrepresentation of an object's position on the image relative to other objects due to variations in the OID and the use of oblique X-ray beams How It Occurs: - Object at Larger OID- When object is located at a larger OID, it means that the object is farther away from the image receptor. - Projection of the Object- The X-ray beam diverges from the X-ray source to the object, and it continues to diverge from the object to the image receptor. - Effect of Divergence: As the X-ray beam diverges, it results in the projection of the object at a position that is further from the central ray than the object with a smaller OID. - Misleading Perception: This divergence effect makes the object with a larger OID appear to be more peripheral or farther from the center of the image than it actually is- it creates the illusion that the object is in a different position relative to other objects in the image. - Impact on Image Accuracy: Location distortion can impact the accuracy of the diagnostic image, as it can lead to misinterpretation of the spatial relationships between objects within the image. Minimize Distortion: - Properly orient object to reduce obliquity (he X-ray beams will be as close to perpendicular to the image receptor as possible) Technique to Intentionally Distort Intentional radiographic distortion techniques are employed in medical imaging to intentionally alter the appearance of structures in X-ray images, primarily to improve the visibility of specific anatomical features. 1. 2. 3. 4. Location Distortion: Objects are repositioned or oriented differently to remove superimposition, allowing for better differentiation between structures that would otherwise overlap. Magnification: Objects are mapped onto a larger area of the image receptor, making their fine details more apparent. This technique is useful for high-resolution imaging to identify small or subtle structures or abnormalities. Geometric Techniques: Changes in X-ray beam angles may introduce controlled distortion to visualize complex structures or assess fractures from multiple angles. Post-processing: Distortion may be introduced or adjusted during digital image post-processing to enhance specific features. Prime Factors to Optimize Image Quality Factors Affecting X-ray Emission: 1. mAs): Directly controls the quantity of X-rays produced. More mAs increases the number of photons reaching the image receptor, resulting in more signal. Less mAs is better for reducing heat production at the anode and the radiation absorbed by the patient. 2. kVp: Influences subject contrast and the percentage of X-rays transmitted. Lower kVp enhances subject contrast, while higher kVp increases signal and reduces patient absorption. Distance (SID - Source-to-Image Distance): Affects geometric unsharpness and magnification distortion. A larger SID minimizes these distortions, whereas a smaller SID reduces the quantity of radiation produced and heat at the anode. Focal Spot Size: Smaller focal spots are better for minimizing geometric unsharpness, while larger focal spots reduce heat concentration on the anode target. Changing Variables: Altering mAs increases photon and energy fluence in both primary and remnant beams. It also affects signal-to-noise ratio (SNR) and differential attenuation. Increasing kVp results in increased photon and energy fluence in both beams, with an impact on SNR. Raising the SID generally increases photon and energy fluence, affecting SNR and differential attenuation. Scatter Radiation: Increased kVp, larger field size, and greater patient thickness contribute to higher scatter radiation. Focal Spot Size Impact: Changing the focal spot size primarily affects geometric unsharpness and heat distribution without directly altering photon fluence or energy fluence in either beam. Exposure - Exposure is proportional to the square of the kilovoltage peak (kVp), which means that it follows the KV² relationship- especially within the diagnostic range of 30 to 150 kVp. - This relationship indicates that variations in kVp have a significant impact on exposure, with higher kVp levels resulting in an exponential increase in exposure due to the increase in the energy fluence of the X-ray beam. 15% rule: - An increase of 15% in kVp means mAs is double Radiation Detection & Measurement - Detection and measurement All detectors require the interaction of radiation with matter, detector has to attenuate and interact with some of the photons - Energy is deposited in matter by ionization and excitation - Most energy deposited in matter is converted to thermal energy - Detectors need signal amplification as energy deposited from single interaction is very small If most of energy deposited is heat, why don't patients feel hot? - Takes lots of photons for that to occur Radiation Detectors 1. 2. 3. 4. 5. 6. All Image Receptors: They are present in traditional film-based radiography, as well as in modern digital radiography systems. Intensifying Screens: In traditional film-based radiography, intensifying screens containing radiation detectors are used to convert X-ray energy into visible light. This visible light, in turn, exposes the film, resulting in the creation of radiographic images. Computed Radiography (CR) Screens: In CR systems, a type of digital radiography, radiation detectors are used to capture the energy released when X-rays interact with a phosphor plate. This energy is then converted into a digital image. Digital Radiography (DR) Detector Panels: DR systems utilize flat-panel detectors containing radiation detectors to directly capture X-rays and convert them into digital images. These detectors offer real-time imaging capabilities and are commonly used in modern radiography. Input Intensifying Screens for Fluoroscopy: Fluoroscopy, which provides real-time X-ray imaging, also uses radiation detectors in the form of intensifying screens to capture and convert X-ray energy into visible light for continuous imaging. CT Detectors: Multiple X-ray detectors arranged in a circular or arc-shaped array are used to capture X-ray data from multiple angles. These detectors are fundamental in creating cross-sectional and three-dimensional images of the body. Ideal Properties of Detector 1. - 2. - - Linear Response to Radiation Quantities: When a radiation detector exhibits a linear response across a range of radiation quantities, it means that the output signal it generates is directly proportional to the amount of radiation it receives. I If the radiation intensity (quantity) doubles, the output signal from the detector will also double. This linear relationship allows for accurate and predictable measurements of radiation exposure. Linear Response Across Photon Energies: A linear response across a range of photon energies indicates that the detector output signal remains directly proportional to the energy of the incoming photons. Different types of radiation detectors are sensitive to photons with varying energy levels. A linear response ensures that the detector's sensitivity is consistent across this range, accurately capturing the characteristics of the radiation beam. Ideal Properties of Detector Wide Dynamic Range: - Can respond to both small and large quantities of radiation (lots of attenuation and little attenuation) - Measure high energy and low energy Stable Response: - Need it to be consistent, does not change over time, or in different environment Methods of Detecting Radiation Scintillon (light emission) - When a material absorbs x-ray photon, or absorbs radiation, and emits light in response - Comes off in every single direction - DR plates, CR, CT, TLD all use Electron (charge) emission - Ionization chamber, metal object filled with gas, whenever x-rays pass through, they cause ionization events (ionize gas molecules) - Positive charge goes to negative, and can be calculated and measured - Cobia meters, and AEC chambers use Methods of Detecting Radiation Scintillon (light emission) - TLD- personal dose detection, emit light proportional to how much radiation absorbed - CT detectors and flat panel detectors, when radiation hits, they immediately give off light - Fluoro- image intensifier converts x-ray photons into light photons 1. Conversion of Light to Electrical Signal: - Scintillators are typically integrated into radiation detection devices, such as photomultiplier tubes (PMTs) or photodiodes. - These devices can capture the emitted light and convert it into an electrical signal, which can then be further processed and analyzed. 2. Phosphors and Light Emission: - Scintillators often use phosphor materials, which are crystalline inorganic salts. - When ionizing radiation interacts with these phosphors, they exhibit three key processes related to light emission: ➔ Absorption: The phosphor material absorbs the energy from the incoming radiation. ➔ Excitation: This absorbed energy excites electrons within the material. ➔ Emission: As the excited electrons return to their ground state, they release energy in the form of visible or ultraviolet light. Luminescence, Fluorescence, & Phosphorescence Luminescence: - Light is given off at some point in time - Wavelength is characteristic of the material (colour) - Can be subdivided based on the timing of when light is given off Fluorescence: - Light emission is IMMEDIATE, within 10^-8 second of stimulation - The electrons in material are excited (energy has been absorbed, it is excited) - Electrons are in elevated higher energy state, away from nucleus, they give off excess energy in form of light and return to natural energy state - Cesium iodide- most common crystal (fluro) Phosphorescence: - Delayed emission of light- beyond 10^-8 seconds - Can be stored, for days, and even months (TLD, CR imaging plate) - The energy is stored/ stuck in localized state - Excess energy comes in, deposited by photons, absorbed by electrons, electrons are raised to higher energy state and stuck there - Lithium fluoride- common Detectors Gas Filled: - Ionization chamber - Some form of gas between two plates or electrodes - When radiation passes through chamber, every ion that flows to electrode will generate electrical signal Solid-State: - Semiconductor detectors, also known as solid-state detectors, are radiation detectors made from pure silicon or germanium crystals with controlled impurities - These detectors operate as diodes, having two terminals - When ionizing radiation interacts with the crystal, it generates electron-hole pairs, which create electrical charges that can be measured as a signal. - Known for their exceptional energy resolution, making them suitable for applications like gamma-ray spectroscopy. GM; - Count interactions, used in nuclear med Spectrometers: - Provides info of energy distribution - Used in therapy to analyze therapeutic beam Dosimeter: - Measures the energy deposited (KERMA) - TLD, dosimeter, screens when estimating Detector Systems Detector and Circuitry Integration: - A detector system combines the actual radiation detector (which could be a scintillator, semiconductor, or Geiger-Mueller tube, among others) with the electronic circuitry Signal Amplification: - The signal produced by the radiation detector is quite weak; to make it more usable and distinguishable from background noise, detector systems include signal amplification circuits. - These circuits boost the strength of the signal, making it more robust for further processing. Signal Processing: - After amplification, the signal may undergo various types of processing, depending on the application. - Signal processing can involve filtering, noise reduction, or shaping the signal to ensure that the relevant data is accurately extracted and that irrelevant information is discarded. Data Storage: - The data generated by the detector system for future reference, analysis, or reporting. - Often incorporate data storage components or interfaces to external data storage devices. Data Analysis and Display: - Beyond data storage, some detector systems may include components for data analysis and display. - This could involve real-time data analysis or the presentation of results to the user in a readable format, such as on a screen or in a printout. Modes of Operation 1. Pulse Mode GM Counters (Geiger-Mueller Counters): Count and measure ionizing radiation interactions, in the form of individual pulses. Each ionization event generates a discrete electrical pulse, which is counted and analyzed in real-time. Scintillation Detectors in Nuclear Medicine: Scintillation detectors used in nuclear medicine also operate in pulse mode. When gamma or other radiation interacts with the scintillating material, it produces individual light pulses, which are then converted into electrical pulses for analysis. Spectrometers: Spectrometers, which are used to measure the energy distribution of ionizing radiation, often employ pulse mode. Each detected interaction results in a pulse, and the energy associated with each pulse is recorded and analyzed to create a spectrum of radiation energies GM Counters: Contain gases with specific properties that are designed for their ionization characteristics when exposed to ionizing radiation. The interaction of ionizing radiation with the gas in GM counters produces millions of ion pairs, creating a strong and easily detectable electrical pulse. The signal generated by GM detectors typically requires little amplification due to the significant ionization effect produced by each interaction. Downside: A period where detector is unable to register new interactions after initial interaction Modes of Operation Pulse mode: 1. Paralyzable Unresponsive for certain period of time, detector cannot register any subsequent interactions Multiple interactions back to back are treated as one- leads to undercounting 2. Non-paralyzable Does not become unresponsive after an interaction Often used where a high event rate is expected, and accurate data acquisition is critical. Spectroscopy: Study and analysis of spectrum, represent varying energy levels They operate in pulse mode The amplitude of each pulse of the detector's output signal is proportional to the energy deposited by the interacting radiation. Modes of Operation 2. Current Mode: The electrical signal generated by each interaction with ionizing radiation is not processed individually but is instead averaged together to form a net current signal. Current Mode in Detectors (e.g., CT Detectors and Ionization Chambers): - The amount of electrical charge collected from each interaction is proportional to the energy deposited by that interaction. - Instead of counting individual events, the accumulated electrical charge represents the total energy deposited in the detector material over a given period of time. Detectors with High Interaction Rates: - Detectors with very high interaction rates are often operated in current mode as individual pulse data from each interaction can be challenging at such high rates- the accumulated current is a more practical measure of radiation exposure. Applications of Current Mode: - Image intensifiers, fluoroscopy, computed tomography (CT) detectors, and Automatic Exposure Control Systems (AECs). - Focus is on measuring the total radiation exposure over time rather than individual interaction events. Detector Efficiency Detector Efficiency: - Ratio of the number of radiation interactions detected by the detector to the number of radiation emissions that occur. Efficiency Calculation: - The efficiency of a detector is calculated using the formula: Efficiency = (Number Detected / Number Emitted) Geometric Efficiency: - This factor is determined by the beam geometry and represents the fraction of emitted radiation that reaches the detector. - It depends on the setup and geometry of the radiation source, as well as the detector's location relative to the source. Intrinsic Efficiency (QDE): - Intrinsic efficiency, or Quantum Detection Efficiency (QDE), is a characteristic of the detector. - It reflects the detector's ability to detect photons effectively and is influenced by factors like the atomic number of the detector material, the energy of the incident photons (kVp), and the thickness of the detector material. Detective Quantum Efficiency (DQE): - In medical imaging, DQE is a descriptor used to quantify the absorption efficiency of a detector. - Factors such as signal-to-noise ratio and contrast. Absorption Coefficient: - The absorption coefficient is a parameter that varies with the energy of the incident radiation. It describes how efficiently a material can absorb radiation at different energy levels. Gas Filled Detector 1. Structure and Operation: Gas-filled detectors consist of a volume of gas sealed between two electrodes. When ionizing radiation passes through the gas, it creates ion pairs by ionizing the gas molecules. 2. Voltage Application: A potential difference, or voltage, is applied between the two electrodes. This voltage creates an electric field within the gas volume. 3. Ionization and Current Flow: As the ion pairs are created by the passage of ionizing radiation, the positive ions are attracted to the negatively charged electrode, and the free electrons are attracted to the positively charged electrode. This movement of charges results in the flow of electric current. 4. Signal Detection: The charges released during the ionization process are detected as an electrical signal that is proportional to the intensity of the incident radiation. The strength of the signal reflects the amount of radiation exposure. 5. Application in AEC: Gas-filled detectors are often used in Automatic Exposure Control (AEC) systems. AEC systems automatically terminate the X-ray exposure when a predetermined amount of radiation has been received, ensuring that the appropriate image density is achieved while minimizing unnecessary radiation exposure to the patient. 6. Gas Type and Properties: The choice of gas used in these detectors can vary, but gases with higher atomic numbers (such as xenon) are often preferred These gases are compressed and pressurized within a small cylinder, with a central electrode in the chamber. Photostimulable Phosphors Optically Stimulated Luminescence Dosimeters (OSLDs) and Computed Radiography (CR) plates are similar to Thermoluminescent Dosimeters (TLDs) 1. Radiation Dosimetry: - TLDs, OSLDs, and CR plates are all used for measuring and quantifying ionizing radiation exposure, making them valuable tools in radiation protection, medical dosimetry, and various applications. 2. Trapping of Electrons: - Like TLDs, OSLDs involve the trapping of electrons in an excited state when exposed to ionizing radiation; In both cases, radiation exposure results in the accumulation of trapped charge. 3. Stimulated Emission of Light: - In TLDs, exposure to heat (thermoluminescence) is used to release the trapped electrons, which results in the emission of visible light, In OSLDs, stimulation is achieved using lasers, causing the trapped electrons to be released and emit light, typically in the form of visible or ultraviolet light. 4. Light Emission Proportional to Energy Absorbed: - The intensity of the emitted light in both TLDs and OSLDs is proportional to the energy of the ionizing radiation that was absorbed by the dosimeter. - This property allows for the quantification of radiation exposure based on the measured light emission. Phototimer - The Lucite component of phototimer chamber is coated with a phosphor material, phosphor coating is sensitive to X-ray radiation When x-ray passes through to phosphor, it emits visible light which is then detected by the AEC system which is able to compensate for patient density and set mAs control factor automatically to ensure proper dose is given to patient Signal Conversion 1. - X-rays to Electrical Signal: In this pathway, X-rays that pass through the patient interact with a detector system, which converts the X-ray energy into an electrical signal directly The detector may consist of materials like amorphous silicon, which is sensitive to X-rays. When X-rays interact with the detector material, they generate electrical charge, and this charge is collected as an electrical signal. 2. X-rays to Light to Electrical Signal: In this pathway, X-rays interact with a scintillator material, typically made of phosphor compounds. When X-rays strike the scintillator, they are converted into visible light. This emitted light is then detected and converted into an electrical signal by a separate component, such as a photodetector. Various types of detectors and technologies are used in digital imaging to implement these conversion pathways, including: Photomultiplier Tubes (PMTs): Nuclear medicine and gamma cameras, to convert light into electrical signals. PMTs offer excellent sensitivity and can amplify low-level light signals. Image Intensifiers (II): Image intensifiers are commonly used in fluoroscopy and some radiography applications. They work by converting X-rays into visible light and then amplifying that light signal before converting it into an electrical signal. Charge-Coupled Devices (CCDs): CCDs are used in some digital radiography systems. They convert visible light into an electrical signal and are known for their high image quality and versatility PMT PMTs have two main functions: 1. Conversion of Light to Electrical Signal: - PMTs convert incident ultraviolet (UV) and visible light photons into an electrical signal by releasing photoelectrons when struck by light- initiated at the photocathode 2. Amplification of the Signal: - PMTs amplify the initial electrical signal; this amplification occurs through a series of components known as dynodes, each of which accelerates and multiplies the number of electrons in the signal. Photocathode: - Emits electrons when exposed to light, made from combinations of antimony and cesium compounds - Positioned just inside the glass window Vacuum Tube: - PMTs operate in a vacuum environment to ensure the efficient and reliable movement of electrons within the detector. Multiple Electrodes: - PMTs consist of multiple electrodes, including dynodes and an anode- these electrodes play a crucial role in the electron multiplication process. PMT Electron Amplification: - The voltage applied to the electrodes, which increases in magnitude at successive dynodes, accelerates electrons to higher potentials and generates more electrons. Dynode Amplification: - Each dynode amplifies the number of electrons by a consistent amount. - The increase in the signal of the PMT depends on the number of dynodes and the gain or amplification factor at each dynode. Calculation of PMT Gain: Solid State Detectors Conductivity: Think of semiconductor materials like silicon and germanium as being somewhere between metals (good conductors) and insulators (poor conductors). Doping: Doping is like adding special ingredients to make the semiconductor work as a detector. There are two types: N-type, where there are extra electrons, and P-type, where there are "holes" where electrons can fit. Energy Bands: Imagine semiconductor materials having energy levels like stairs. Electrons can hang out on one set of stairs (valence band), but they need a boost to get to a higher set of stairs (conduction band). There's a gap between the stairs (forbidden energy gap). P-N Junction: A P-N junction is like a one-way street for electrons. When you give it a push (apply a voltage), electrons can go in one direction but not the other. Radiation Detection in P-N Junction: When light (or X-rays) hits the detector, it's like shaking things up. Some electrons get excited and jump to the higher stairs. This creates pairs of electrons and holes, which move to different sides, causing a temporary flow of electricity. These pairs are pulled to opposite sides of the diode, resulting in a temporary flow of current that is proportional to the energy deposited by the photons.