BIOL 366 Lecture 14: Post Transcriptional Regulation PDF
Document Details
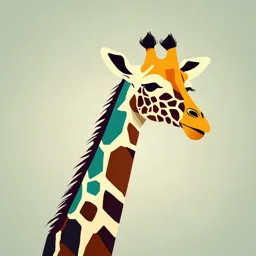
Uploaded by NeatGrace
Tags
Related
Summary
This document details a lecture on post-transcriptional regulation of gene expression in bacteria. It covers various mechanisms, including riboswitches and translational feedback, which allow for rapid protein synthesis control in response to molecular signals. The presentation features diagrams and examples to illustrate the concepts.
Full Transcript
BIOL 366 Lecture 14 Post transcriptional regulation of gene expression Readings: Text Section: 20.2.1 – 20.2.2 and Articles (on library reserve): 1. Antibiotic Resistance: Current Perspectives. Authors: Anushya Petchiappan and Dipankar Chatterji. Source: ACS Omega 2017, 2, 7400-7409. 2. The ribB F...
BIOL 366 Lecture 14 Post transcriptional regulation of gene expression Readings: Text Section: 20.2.1 – 20.2.2 and Articles (on library reserve): 1. Antibiotic Resistance: Current Perspectives. Authors: Anushya Petchiappan and Dipankar Chatterji. Source: ACS Omega 2017, 2, 7400-7409. 2. The ribB FMN riboswitch from Escherichia coli operates at the transcriptional and translational level and regulates riboflavin biosynthesis. Authors: Danielle Pedrolli1, Simone Langer, Birgit Hob, Julia Schwarz, Masayuki Hashimoto and Matthias Mack. Source: FEBS Journal 282 (2015) 3230–3242. doi:10.1111/febs.13226 Key terms: riboswitch, translational repressor, stringent response, stringent factor. Text (Chapter 20) problems: 10, 11, 13, 14, 15, 17, 18. 1 Review of Last lecture: Last lecture we discussed “transcriptional” control of gene expression in bacteria, and looked at: - lac operon; positive and negative regulation with different proteins - ara operon: positive and negative regulation with same proteins - trp operon: Attenuation - Today we will look at some post transcription regulatory mechanism 2 Posttranscriptional control of control of gene expression enables: • Rapid up- or down-regulation of protein synthesis in response to molecular signals. 3 Regulation via translational feedback: Example: The r-protein operons • In bacteria, increased demand for protein synthesis is met by increasing the number of ribosomes. – Ribosomal proteins can make up to 45% of total cellular proteins in rapidly growing cells • Bacteria coordinate the synthesis of the ribosomal components ➢ r-proteins ➢ r-RNAs FIGURE 18-3 4 Regulation via translational feedback: The r-protein operons • The r-proteins are encoded by 52 genes, present in ~20 operons (1-11 genes, each) • One r-protein in each operon functions as a translational repressor – The repressor can bind rRNA (preferred) – The repressor can also bind the operon’s mRNA and blocks its translation Fig 20-18: One r-protein operon 5 Regulation of an r-protein operon via translational feedback mechanism • When [r-protein] is low relative to [rRNA]: ➢ The translational repressor (L4) bind rRNA only ➢ L4 is NOT available to bind r-protein mRNA ➢ Translation proceeds Fig 20-18 6 Regulation of an r-protein operon via translational feedback mechanism • When [r-protein] is higher than [rRNA] (no need for more r-protein) ➢ L4 proteins saturate rRNA ➢ Some L4 protein bind mRNA and repress translation Fig 20-18 7 Some RNA species have regulatory elements/regions known as Riboswitches. Riboswitches: • Exist within the 5′ untranslated region (5′UTR) of mRNA • Are found in bacteria and some eukaryotes (typically not mammals) • Are most common in bacteria. • Include: ➢ a regulatory region ➢ a [small molecule / metabolite]–binding element Fig. 20-14 8 Riboswitches can function at the level of transcription • Transcription proceeds when mRNA is not bound to metabolite • Specific metabolite binds the molecule–binding element • Binding of metabolite (the ligand) changes the shape of the entire RNA molecule • Transcription is terminated Fig. 20-14 9 Riboswitches can function at the level of translation No specific metabolite: • Translation proceeds Specific metabolite present: • Metabolite binds and alter mRNA structure • Sequesters the ShineDalgarno sequence • Halts translation 10 Example: The thiamine pyrophosphate (TPP)–binding riboswitch AKA THI box or THI element (Note: TPP is the active form of thiamine AKA vitamin B1) The THI box / element: • Is located in the 5′UTR region of mRNAs involved in vitamin B1 biosynthesis • Controls gene expression by inhibiting translation when [vitamin B1] is high • Is one of the few riboswitches found across most organisms (archaea, some eukaryotes, and bacteria) 11 Mode of action of the TPP riboswitch When TPP is present (upper diagram) – TPP binds the riboswitch – The riboswitch changes conformation – The Shine-Dalgarno sequence is sequestered – Translation is prevented When TPP is NOT present (lower diagram) – Translation is on 12 Riboswitches can affect mRNA stability; e.g., the glmS riboswitch. Note: The glmS gene encodes an enzyme that catalyzes the reaction: Fructose 6-phosphate + glutamine → glucosamine 6-phosphate • Glucosamine 6-phosphate (GlcN6-P) controls the expression of the glmS mRNA • On binding of GlcN6-P, the glmS pre-mRNA degrades itself (i.e., it is a ribozyme) 13 Riboswitches can discriminate between chemically related molecules Discrimination can be based on: • Atomic charge • Stereochemistry • The presence or absence of particular functional groups Example: The glmS riboswitch. Fig. 20-17: Structure of (GlcN6-P), the metabolite for the glmS riboswitch, and a few of its analogs. 14 Riboswitches can be very specific to their ligands. Result of agarose gel electrophoresis of ribonuclease-cleaved samples (Fig. 20-17c). The glmS mRNA was incubated with its known effector (glucosamine 6-phosphate) or the other compounds shown in (b). The riboswitch specifically recognizes GlnN6P Fig. 20-17c: Degradation of glmS mRNA triggered by different ligands. 15 Some properties of riboswitch-regulated mRNAs (1) Have unusually long sequences upstream from the translation start site that are necessary to form the riboswitch. (2) The upstream sequence is well-conserved in the same mRNA in different bacterial species, and sometimes in archaea, plants, and fungi. (3) Do not have protein binding partners, consistent with their ability to function directly to regulate gene expression. 16 There are several classes of Riboswitches (RS) • RS’s are named after the ligand they bind • Over 15 classes are known, classified based on ligand type and RS secondary structure. 17 Utility of riboswitches Many bacteria are developing resistance to antibiotics. How? 18 Overview of metabolic pathways targets for some common antibiotics Reference: Antibiotic Resistance: Current Perspectives, by Anushya Petchiappan and Dipankar Chatterji. ACS Omega 2017, 2, 7400-7409. 19 Major mechanisms of antibiotic resistance development. Figure 2a. Resistant bacteria can: - Decrease antibiotic influx - Increase antibiotic efflux 20 Major mechanisms of antibiotic resistance development. Figure 2b. Resistant bacteria – Degrade antibiotic – Modify antibiotic by adding chemical group 21 Major mechanisms of antibiotic resistance development. Figure 2c. Resistant bacteria - Protect antibiotic target - Produce alternative protein to do the function Figure 2. Three main mechanisms of antibiotic resistance development in bacteria. 22 Utility of Riboswitches; the FMN riboswitch Example: The FMN riboswitch. – This riboswitch controls the expression of genes involved in the biosynthesis of riboflavin (vitamin B2). – Riboflavin is a precursor to synthesizing cofactors like FMN. Structures of FMN, roseoflavin antibiotic, and ribocil-C. Note: Roseoflavin and Ribocil-C inhibit synthesis of vitamin B2 and FMN Reference: Antibiotic Resistance: Current Perspectives, by Anushya Petchiappan and Dipankar Chatterji. ACS Omega 2017, 2, 7400-7409. 23 Background on riboflavin (vitamin B2) biosynthesis: In eubacteria (e.g., E. coli & B. subtilis) riboflavin (vitamin B2) is synthesized from GTP and two molecules of ribulose 5-phosphate via a series of enzymatic reactions. The enzymes involved are: i) GTP cyclohydrolase II (RibA) ii) 3,4-dihydroxy2-butanone-4-phosphate synthase (RibB) iii) a riboflavin-specific deaminase/reductase (RibDG) iv) lumazine synthase (RibH) v) Riboflavin synthase (RibE) vi) RibCF These genes are part of a single transcription unit (ribDG-E-AB-H) whose expression is regulated by a FMN riboswitch (ribDG FMN riboswitch) located in the 50 -UTR of the “ribDG-E-AB-H” mRNA. 24 The ribDG-E-AB-H transcriptional unit. • Genes shown as yellow arrows are involved in vitamin B2 biosynthesis. • Genes shown as gray arrows are not involved in vitamin B2 biosynthesis. 25 Utility of Riboswitches; the FMN riboswitch • The FMN riboswitch controls the riboflavin (vitamin B2) biosynthesis pathway • The pathway is essential in the pathogenic bacterium M. tuberculosis, as it lacks riboflavin transport genes. • So inhibitors of FMS synthesis act as antibiotics for this bacterium. Reference: Antibiotic Resistance: Current Perspectives, by Anushya Petchiappan and Dipankar Chatterji. ACS Omega 2017, 2, 7400-7409. 26 Utility of Riboswitches; the FMN riboswitch In the absence of FMN, mRNA is produced and translated. When abundant, FMN binds riboswitch causing premature termination of gene expression. Binding of FMN to its target results in a conformational change and formation of a terminator/sequester loop, thereby abolishing gene expression. Both transcription & translation can be inhibited with this riboswitch. Reference: Antibiotic Resistance: Current Perspectives, by Anushya Petchiappan and Dipankar Chatterji. ACS Omega 2017, 2, 7400-7409. 27 Utility of Riboswitches; the FMN riboswitch Structures of FMN, & 2 analogs roseoflavin and ribocil-C. Note: Roseoflavin and Ribocil-C inhibit synthesis of vitamin B2 and FMN Reference: Antibiotic Resistance: Current Perspectives, by Anushya Petchiappan and Dipankar Chatterji. ACS Omega 2017, 2, 7400-7409. 28