L04_allometry PDF - Principles in Ecology
Document Details
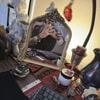
Uploaded by rafawar1000
Florida Atlantic University
Tags
Summary
This document presents lecture notes on ecology, focusing on topics like photosynthesis and heterotrophic processes, particularly in the context of energy consumption and the study of organismal adaptations for food consumption. The document also briefly introduces allometry, and covers concepts in metabolic rate and scaling.
Full Transcript
Principles in Ecology PCB 4043 Energy + Allometry Prof. Fahimipour Topics: Scaling, metabolism, energy [email protected] D: 437 DW | B: 206 Sanson Photosynthetic Pathways The C4 pathway uses more ATP, but greater efficiency gives these plants an advantage over C...
Principles in Ecology PCB 4043 Energy + Allometry Prof. Fahimipour Topics: Scaling, metabolism, energy [email protected] D: 437 DW | B: 206 Sanson Photosynthetic Pathways The C4 pathway uses more ATP, but greater efficiency gives these plants an advantage over C3 plants at high temperatures. Water use efficiency: transpiration losses are minimized—PEPcase can take up CO2 when stomates are not fully open. If photosynthetic rates determine ecological success, climatic patterns should predict regions where C4 plants will dominate. There is a close correlation between temperature and the proportion of C4 species in the community. Photosynthetic Pathways Crassulacean acid metabolism (CAM) minimizes water loss by opening stomates at night when it’s cooler and humidity is higher, and closing them during the day. – Night: CAM plants take up CO2 using PEPcase to make a 4- carbon organic acid and store it in vacuoles. – Day: the molecule is broken down to supply CO2 for the Calvin cycle. High CO2 concentrations reduce photorespiration. Figure 5.15 C3, C4, and CAM Photosynthesis Compared Figure 5.16 Crassulacean Acid Metabolism Photosynthetic Pathways CAM plants are often succulent, with thick, fleshy leaves or stems; common in arid and saline environments. Some occur in the humid tropics—mostly epiphytes that grow on tree branches and have less access to water. Also found in some aquatic plants such as quillwort (Isoetes). – The rate of CO2 diffusion into water is slow; CAM may facilitate CO2 uptake at low CO2 concentrations in aquatic environments. Figure 5.17 Examples of Plants with the CAM Photosynthetic Pathway Photosynthetic Pathways Some plants can switch between C3 and CAM (facultative CAM). – When water is abundant, they use the C3 pathway, which allows more carbon gain. – If conditions become arid or saline, they switch to CAM. It is irreversible in some species but not in others. Heterotrophy Focus on Understanding how the chemical makeup of a food item determines the benefit it provides to the consumer eating it. Explain how morphological and behavioral adaptations enable heterotrophs to obtain food more efficiently. Describe how increasing complexity in the digestive systems of heterotrophs makes the assimilation of energy and nutrients more efficient. Heterotrophs: things that eat stuff Heterotrophs consume energy-rich organic compounds (food) and convert them into usable chemical energy (ATP). Energy gain depends on the chemistry of the food and the effort needed to find and ingest the food. – Soil microorganisms that feed on detritus invest little energy to find food, but it has low energy content. – A cheetah hunting a gazelle invests a lot of energy to find, chase, and kill its prey, but it gets an energy-rich meal. Heterotrophy Constituents of food have varying energy content and ease of assimilation. – Fiber: compounds such as cellulose; most heterotrophs can’t break it down (at least without microbial help). – Most energy is in carbohydrates, proteins, and especially fats. Amino acids also provide nitrogen. – Secondary compounds: not a good energy source, can be toxic. (AI-generate image) Figure 5.18 Categorical Breakdown of Food Chemistry Heterotrophy Animal cells are generally more energy-rich than plant, fungal, and bacterial cells, which tend to have more fiber. Herbivores generally must eat more biomass to get the same benefit that carnivores do, but carnivores may spend more energy finding food. Heterotrophy Archaea, bacteria, and fungi excrete enzymes into the environment to break down organic matter: they digest their food outside their bodies. They have adapted to a wide variety of food sources and produce a wide variety of enzymes to break them down. – This ability is exploited for bioremediation. – Fuels, pesticides, sewage, etc. are cleaned up by microorganisms that can break down the chemicals, e.g., after the Deepwater Horizon oil spill. Heterotrophy Evolution of mobility was probably associated with the need to find food sources and avoid being eaten by others. Animals display tremendous diversity in specialized feeding adaptations, reflecting the diversity of foods they consume. Heterotrophy All insects have several paired appendages used to capture, handle, and consume food. – Variation in these mouthparts reflects feeding specializations. Birds also have variation in mouthparts (bills). – Variation in bill morphology reflects adaptations to optimize food gathering and minimize competition among groups of birds. Figure 5.20 Variations on a Theme: Insect Mouthparts Figure 5.21 Variations on a Theme: Bird Bills Heterotrophy Heterotrophs must transform food into simpler molecules: proteins, carbohydrates, and fats are broken down into amino acids, simple sugars, and fatty acids. Small protozoans ingest food particles that are digested in special organelles. Multicellular animals have specialized tissues for absorption, digestion, transport, and excretion. An animal’s diet influences digestive adaptations. Heterotrophy Herbivores consume plants with large amounts of fiber. Their digestive tracts are longer than those of carnivores, which increases food processing time and surface area for absorption. Some re-ingest their feces (coprophagy) to enhance digestion Close associations with microbes who break down plant matter Figure 5.23 Herbivores Have Long Digestive Systems Heterotrophy Most herbivores have bacterial symbionts that enhance digestion (but see some Caterpillars). Ruminants have a specialized stomach compartment (rumen) in which large populations of bacteria facilitate breakdown of cellulose. Ruminants also exhibit rumination (cud chewing)—regurgitation of material from a forestomach for additional chewing. Allows them to eat food quickly and reduce exposure to predators, then more thoroughly chew and digest their food at a later time. Allometry A metabolic network Metabolism Impacts Organismal Ecology Metabolism: the total energy expenditure for an organism's physiological processes Unveiling Kleiber’s Law and Scaling Now let’s look at one of the most famous biological relationships… the depiction of metabolic rate, measured as heat produced per day in kilocalories, as a function of species’ body mass (kg), known as the Kleiber Law Unveiling Kleiber’s Law and Scaling Max Kleiber in the 1930s discovered that metabolic rate scales in a very particular way with organismal body size. First, notice that the scales of the x- and y-axis are log- scaled… meaning each successive quadrant is 10x the size of the previous quadrant. With this log-spacing, the metabolic rate of species is linearly scaled with body mass. Question: As a quick refresher, what does it mean when we look at a log-log plot? Origins of metabolic scaling Why would metabolic rate B be expected to scale with body mass M, and what scaling would be expected? (Remember - when we are discussing types of scaling, we are really talking about the value of b in the equation for metabolic rate B = aMb Building Intuition There are two things to keep in mind… if we imagine a spherical organism increasing in size, the surface area and volume of a sphere scale differently: Building Intuition There are two things to keep in mind… if we imagine a spherical organism increasing in size, the surface area and volume of a sphere scale differently: Building Intuition The HEAT produced by metabolism is produced in proportion to an organism’s volume (because the number of cells is in proportion to volume and each cell produces heat). However the heat must be DISSIPATED across the organism’s surface area. Building Intuition BUT we know this wouldn’t work because the organism wouldn’t be able to dissipate metabolic energy as heat, which would cause it to messily explode. The metabolism has to be turned down, it cannot scale with volume. So we can suppose that metabolic rate B should be proportional to mass M tuned down by an exponent of 2/3: B∝M2/3 Cheating 3d with fractals We’ve spent the last few minutes convincing ourselves that a 2/3 scaling is a reasonable expectation based on the geometry of 3D organisms. But there is a better solution than a 2/3 scaling. The reasoning behind a 3/4 scaling is that the primary limitation is the DISTRIBUTION of materials (nutrients, gasses, waste) throughout our bodies, all of which is facilitated by distribution channels that exhibit fractal branching geometries. Strangely, these fractal shapes have a dimensionality closer to 4D rather than 3D. The mechanisms themselves are complex, and involve advanced mathematical and physical reasoning. If you dig that kind of thing, check out this paper. Population sizes - If we go out in nature and begin measuring things, it is often surprising how many qualities of ecological systems exhibit scaling relationships that appear to relate, and sometimes be the same as, the 3/4 power law. - While the 3/4 power law for metabolism has some very well-defined theory that may explain the underlying mechanism, many other power-scale ecological relationships remain mysterious.