lecture 14 studyguide start of test material for test 3- ecology.pdf
Document Details
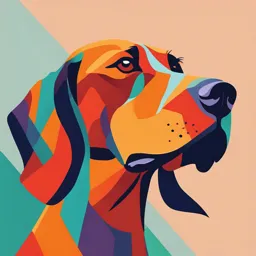
Uploaded by LuxuryOrphism
Tags
Full Transcript
Predation is a biological interaction in which one organism, known as the predator, consumes another organism, known as the prey, for food. Predation plays a significant role in ecological systems and has various forms, including herbivory, carnivory, and cannibalism. 1.Herbivory: Herbivory is a typ...
Predation is a biological interaction in which one organism, known as the predator, consumes another organism, known as the prey, for food. Predation plays a significant role in ecological systems and has various forms, including herbivory, carnivory, and cannibalism. 1.Herbivory: Herbivory is a type of predation where the predator, known as the herbivore, feeds primarily on plants or plant materials. Herbivores consume parts of plants such as leaves, stems, fruits, seeds, or roots. Examples of herbivores include deer, cows, rabbits, and many insects like caterpillars and grasshoppers. Herbivory is essential for maintaining the balance of plant populations within ecosystems and can influence plant evolution through selective pressure. 2.Carnivory: Carnivory is another form of predation in which the predator, known as the carnivore, feeds primarily on other animals. Carnivores can range from small predators like spiders and insects to larger ones like lions, wolves, and sharks. Carnivores have various adaptations such as sharp teeth, claws, and keen senses to capture, kill, and consume their prey efficiently. Carnivory helps regulate prey populations, control herbivore numbers, and shape community structures in ecosystems. 3.Cannibalism: Cannibalism is a form of predation in which an organism consumes members of its own species. This behavior can occur in various organisms, including 1 insects, fish, amphibians, birds, and mammals. Cannibalism may happen for various reasons such as food scarcity, competition, or as a reproductive strategy. In some cases, cannibalism occurs in certain life stages, such as larvae consuming other larvae or juveniles consuming weaker members of their own species. Cannibalism can have ecological implications by influencing population dynamics and genetic traits within a species. Overall, predation in its various forms (herbivory, carnivory, and cannibalism) is a fundamental aspect of ecological interactions, playing a crucial role in shaping ecosystems and maintaining biodiversity. 1 The world appears green primarily due to the presence of chlorophyll, a green pigment found in plants, algae, and cyanobacteria. Chlorophyll is crucial for photosynthesis, the process by which plants and other photosynthetic organisms convert light energy into chemical energy, ultimately producing glucose and oxygen from carbon dioxide and water. Here's why the world is green: 1.Photosynthesis: Chlorophyll absorbs light energy from the sun, particularly in the blue and red regions of the spectrum, while reflecting green light. This reflected green light gives plants their characteristic green color. Since chlorophyll is the predominant pigment in most plants, the overall effect is that the world appears green. 2.Abundance of Plants: Plants are the most widespread photosynthetic organisms on Earth, covering vast areas of land and inhabiting various ecosystems. Because of their abundance, the green color of plants dominates the landscape. 3.Evolutionary Advantage: The green coloration of plants has evolutionary advantages. It allows them to efficiently capture light energy for photosynthesis, which is essential for their growth, survival, and reproduction. 4.Biodiversity: While chlorophyll is the primary pigment responsible for the green coloration of plants, other pigments may contribute to variations in color, such as 2 yellow, red, or purple. However, green is the dominant color due to the widespread presence of chlorophyll-containing plants. In summary, the world appears green because of the prevalence of chlorophyll in plants, which reflects green light while absorbing other wavelengths for photosynthesis. This phenomenon, coupled with the abundance of plants across various ecosystems, gives the Earth its characteristic green hue. 2 Certainly! Here's a brief explanation using the Lotka-Volterra (L-V) predator-prey model: In the Lotka-Volterra predator-prey model, which is a set of differential equations describing the dynamics of predator-prey interactions, the populations of predators (Npred) and prey (Nprey) are regulated by factors such as birth rates (bpred and bprey) and death rates (dpred and dprey). These rates determine how the populations change over time. The equations governing the L-V predator-prey model are as follows: For prey (Nprey): dNpreydt=rNprey−cNpredNpreydtdNprey=rNprey−cNpredNpreyFor predators (Npred): dNpreddt=acNpredNprey−mNpreddtdNpred=acNpredNprey−mNpredHere, r represents the intrinsic growth rate of the prey population, c represents the predation rate, a represents the efficiency of prey conversion to predator offspring, and m represents the death rate of predators. These equations illustrate how changes in predator and prey populations are regulated by interactions between them. For instance, an increase in the prey population (Nprey) may lead to a corresponding increase in the predator population (Npred) due to more food availability, which in turn may lead to a decrease in the prey population as predation increases. Conversely, a decrease in prey population 3 may lead to a decrease in predator population due to reduced food availability. Thus, the birth and death rates of both predators and prey are regulated by the dynamics of the predator-prey interaction, as described by the Lotka-Volterra equations. 3 The Lotka-Volterra equations, also known as the predator-prey equations, are a pair of differential equations that describe the dynamics of biological systems where two species interact: a predator and its prey. These equations were developed independently by Alfred J. Lotka and Vito Volterra in the early 20th century. The equations are a cornerstone of mathematical ecology and help us understand the dynamics of predator-prey relationships in ecosystems. The basic Lotka-Volterra predator-prey model consists of two equations: 1.For the prey (Nprey): dNpreydt=rNprey−cNpredNpreydtdNprey=rNprey−cNpredNpreyFor the predators (Npred): dNpreddt=acNpredNprey−mNpreddtdNpred=acNpredNprey−mNpredIn these equations: 2.NpreyNprey and NpredNpred represent the population sizes of the prey and predators, respectively, at time tt.rr is the intrinsic growth rate of the prey population in the absence of predation.cc is the capture efficiency or predation rate, which determines how many prey are captured by each predator.aa is the conversion efficiency, representing how efficiently prey are converted into predator offspring.mm is the mortality rate of the predator population.The first equation represents the change in the prey population over time. The term rNpreyrNprey represents the prey's intrinsic growth, while cNpredNpreycNpredNprey represents the predation 4 rate, where the number of prey consumed is proportional to both the predator and prey populations.The second equation represents the change in the predator population over time. The term acNpredNpreyacNpredNprey represents the birth rate of predators, which depends on both the predator and prey populations, while mNpredmNpred represents the predator mortality rate.These equations form a coupled system, where the populations of predators and prey interact with each other. The dynamics of the system can lead to cyclic fluctuations in population sizes, where increases in prey populations lead to increases in predator populations, which in turn lead to decreases in prey populations, and so on. This cyclical behavior is a characteristic feature of many predator-prey interactions in nature. 4 5 6 7 8 9 10 11 Certainly! Let's delve into each of the points you've mentioned: 1.Symbiosis, Parasitism, Mutualism Definitions, and Types of Interactions: 1. Symbiosis: Symbiosis refers to a close and long-term interaction between two different species living together. It can be beneficial, harmful, or neutral for one or both species involved. 2. Parasitism: Parasitism is a type of symbiotic relationship in which one organism, the parasite, benefits at the expense of another organism, the host. Parasites derive nourishment and shelter from the host, often causing harm or disease in the process. 3. Mutualism: Mutualism is another type of symbiotic relationship in which both organisms involved benefit from the interaction. Both species gain something from the relationship, whether it's food, protection, or other resources. 4. Types of Interactions: Within these categories, interactions can vary. For example, parasites can be ectoparasites (living on the outside of the host) or endoparasites (living inside the host's body). Mutualistic relationships can range from obligate mutualism, where both species are entirely dependent on each other, to facultative mutualism, where the relationship is beneficial but not essential for survival. 12 2. Parasite Transmission, Hosts, and Life Cycle: 1. Parasite Transmission: Parasites can be transmitted to hosts through various means, including direct contact, ingestion of contaminated food or water, insect vectors, and environmental exposure. 2. Hosts: Hosts are organisms that harbor parasites and provide them with a habitat and resources for survival and reproduction. Hosts can be diverse, ranging from individual animals or plants to entire populations or communities. 3. Life Cycle: Parasites often have complex life cycles involving multiple stages and hosts. For example, some parasites require intermediate hosts to complete their life cycle before infecting their final host, while others have direct life cycles with only one host species involved. 3.Consequences and Benefits of Symbioses: 1. Consequences: The consequences of symbiotic relationships can vary widely depending on the type of interaction. Parasitism can lead to harm or disease in the host, impacting its health, reproduction, and survival. Mutualism can provide benefits such as improved nutrient acquisition, protection from predators, or enhanced reproductive success. However, there can also be costs associated with mutualistic interactions, such as the energy or resources invested in maintaining the relationship. 2. Benefits of Symbioses- particularly mutualistic relationships, can offer various benefits to the organisms involved. These benefits may include increased fitness, access to resources that would otherwise be unavailable, enhanced defense against predators or pathogens, and opportunities for coevolution and specialization. Understanding the dynamics of symbiotic interactions, including parasitism and mutualism, is essential for comprehending the complexity of ecological systems and the interconnectedness of species within them. 12 Micro- and macroparasites are two categories used to classify parasites based on their size, life cycle, and mode of transmission. Here's a breakdown of each: 1.Micro-parasites: 1. Size: Micro-parasites are typically microscopic, meaning they are too small to be seen with the naked eye. Examples include bacteria, viruses, and protozoa. 2. Life Cycle: Micro-parasites often have direct life cycles, meaning they can complete their entire life cycle within a single host species. They may also have indirect life cycles, involving multiple hosts or vectors. 3. Mode of Transmission: Micro-parasites are usually transmitted directly between hosts, often through contact, ingestion, or inhalation of infectious particles. They can also be transmitted indirectly through vectors like mosquitoes or ticks. 2.Macroparasites: 1. Size: Macroparasites are larger organisms that can be seen with the naked eye. Examples include worms (helminths), fleas, ticks, and lice. 2. Life Cycle: Macroparasites typically have more complex life cycles compared to micro-parasites. Many macroparasites have indirect life cycles involving intermediate hosts or vectors. For example, parasitic 13 worms often require intermediate hosts like snails or insects to complete their life cycle before infecting the final host. 3. Mode of Transmission: Macroparasites often require a vector or intermediate host for transmission. For example, ticks transmit Lyme disease-causing bacteria, while mosquitoes transmit malaria parasites. Direct transmission can also occur through contact with infected individuals or contaminated environments. Both micro- and macroparasites can have significant impacts on host populations and ecosystems. Micro-parasites like viruses and bacteria are often associated with acute infections that spread rapidly through host populations, leading to outbreaks or epidemics. Macroparasites, on the other hand, may cause chronic infections and have slower transmission dynamics. Understanding the differences between micro- and macroparasites is crucial for developing effective strategies for parasite control and management in both human and animal populations. 13 Hemi- and holoparasites are two categories of parasitic plants based on their degree of dependence on their host plants. Here's an explanation of each: 1.Hemiparasites: 1. Degree of Dependence: Hemiparasites are partially parasitic plants that have some ability to photosynthesize and produce their own organic compounds but still rely on their host plants for some nutrients or water. 2. Structure: Hemiparasites typically have specialized structures called haustoria, which penetrate the host plant's vascular system to extract water, nutrients, or both. These haustoria facilitate the transfer of resources from the host to the parasite. 3. Examples: Some common examples of hemiparasitic plants include mistletoes (such as species in the genus Viscum) and dodder (Cuscuta spp.). 2.Holoparasites: 1. Degree of Dependence: Holoparasites are completely parasitic plants that lack chlorophyll and are entirely dependent on their host plants for water, nutrients, and organic compounds. 2. Structure: Unlike hemiparasites, holoparasites do not engage in photosynthesis and lack chlorophyll. Instead, they rely entirely on 14 specialized structures like haustoria to extract resources from their host plants. 3. Examples: Common examples of holoparasitic plants include broomrapes (Orobanche spp.) and Indian paintbrushes (Castilleja spp.). Both hemi- and holoparasites can have significant ecological impacts on their host plants and ecosystems. They can weaken host plants by competing for resources, reducing their growth and reproductive success, and making them more susceptible to environmental stresses or pathogens. Additionally, parasitic plants play important roles in nutrient cycling and ecosystem dynamics, particularly in certain habitats where they are abundant. Understanding the differences between hemi- and holoparasites is essential for studying their ecological interactions and developing strategies for their management in agricultural and natural ecosystems. 14 Endo- and ectoparasites are two categories of parasites based on their location in relation to their host's body. Here's an explanation of each: 1.Endoparasites: 1. Location: Endoparasites are parasites that live inside the body of their host. They may inhabit various organs or tissues, such as the digestive tract, bloodstream, muscles, or organs. 2. Examples: Examples of endoparasites include various types of worms (helminths), such as intestinal parasites like roundworms, tapeworms, and flukes. Additionally, certain protozoa and some types of fungi and bacteria can also be endoparasites. 2.Ectoparasites: 1. Location: Ectoparasites are parasites that live on the external surface of their host's body. They typically attach to the skin, feathers, fur, or scales of their host. 2. Examples: Common examples of ectoparasites include fleas, ticks, lice, mites, and certain species of flies. These parasites can cause irritation, itching, and discomfort to their hosts and may also transmit diseases. Both endo- and ectoparasites can have significant impacts on the health and wellbeing of their hosts. They can cause a variety of symptoms and health problems, 15 ranging from mild irritation to severe illness or even death, depending on factors such as parasite load, host susceptibility, and the presence of co-infections or secondary complications. Understanding the differences between endo- and ectoparasites is important for diagnosing and treating parasitic infections effectively. Treatment approaches may vary depending on the type and location of the parasite, as well as the specific characteristics of the host and the environment. Additionally, control measures for preventing parasitic infections may target different stages of the parasite's life cycle or focus on interrupting transmission pathways, depending on whether the parasite is an endo- or ectoparasite. 15 Parasites with complex life cycles often require multiple hosts to complete their life cycle stages. These hosts are categorized based on the roles they play in the parasite's development. The two primary types of hosts in these complex life cycles are: 1.Definitive Host: 1. The definitive host is the host in which the parasite reaches sexual maturity and reproduces. 2. In the definitive host, the parasite typically undergoes sexual reproduction, producing offspring or reproductive stages that are capable of infecting other hosts. 3. The definitive host is often the host in which the parasite causes the most harm or pathology. 4. Examples of definitive hosts include humans, domestic animals, or other vertebrates, depending on the parasite species. 2.Intermediate Host: 1. The intermediate host is an additional host required by the parasite to undergo certain developmental stages but is not where sexual reproduction occurs. 2. In the intermediate host, the parasite may undergo asexual reproduction, 16 development into a different life stage, or other necessary transformations. 3. The intermediate host may play a crucial role in the transmission of the parasite to the definitive host. 4. Examples of intermediate hosts include various invertebrates, such as snails, insects, or crustaceans, as well as other vertebrates, depending on the parasite species. Parasites with complex life cycles often alternate between definitive and intermediate hosts to complete their life cycle stages. For example, many parasitic worms (helminths) have complex life cycles involving intermediate hosts such as snails or insects, where larval stages develop, and definitive hosts such as mammals, where sexual reproduction occurs. Similarly, many protozoan parasites, such as those causing malaria or toxoplasmosis, have complex life cycles involving both vertebrate and invertebrate hosts. Understanding these complex life cycles is crucial for developing strategies to control parasitic infections and interrupt transmission pathways. 16 Investing in defense mechanisms against parasites is a common strategy employed by host organisms to mitigate the negative consequences of parasitic infections. Here are examples of specific host defense mechanisms exhibited by various plant species in response to parasitic interactions: 1.Spruce Cone Gall: 1. Host Plant: Spruce trees 2. Defense Mechanism: When infected by the spruce cone gall adelgid (Adelges cooleyi), spruce trees often produce galls in response to the parasitic invasion. These galls are abnormal growths formed by the plant in reaction to the presence of the parasite. They serve as physical barriers that encapsulate and isolate the parasitic adelgids, preventing their spread and reducing the damage inflicted on the host plant. 2.Oak Succulent Gall: 1. Host Plant: Oak trees 2. Defense Mechanism: The oak succulent gall is formed in response to parasitic wasps, such as the gall wasp (Cynips spp.), which induce abnormal growths on the leaves or stems of oak trees. These galls provide shelter and nutrition for the developing wasp larvae. In some cases, the host plant may invest resources in producing toxic or repellent 17 compounds within the gall tissues to deter further parasitic infestation or to limit the growth and development of the parasitic larvae. 3. Oak Bullet Gall: 1. Host Plant: Oak trees 2. Defense Mechanism: Similar to the oak succulent gall, the oak bullet gall is induced by parasitic wasps, such as the oak gall wasp (Disholcaspis spp.). The gall provides a protected environment for the developing wasp larvae. In response to parasitic infestation, the host plant may allocate resources towards strengthening the gall structure or producing defensive compounds that inhibit the growth and development of the parasitic larvae, thereby reducing the overall impact of the infestation on the host plant. 4.Goldenrod Ball Gall: 1. Host Plant: Goldenrod plants 2. Defense Mechanism: Goldenrod ball galls are induced by parasitic flies, such as the goldenrod gall fly (Eurosta solidaginis). These galls are formed on the stems of goldenrod plants and serve as shelters for the developing fly larvae. Host plants may invest resources in producing secondary metabolites or physical barriers within the gall tissues to deter parasitic infestation or limit the fitness of the parasitic larvae, thus reducing the negative consequences of the parasitic interaction on the host plant. In summary, host plants employ various defense mechanisms, including the formation of galls and the production of defensive compounds, to counteract the negative effects of parasitic infections and limit the success of the parasites. These defense strategies can help mitigate the impact of parasitic interactions on host fitness and reproductive success. 17 Parasitic infections can decrease host fitness by: 1.Reducing reproductive output. 2.Impeding growth and development. 3.Increasing mortality rates. 4.Suppressing immune function. 5.Altering behavior. 6.Incurring energetic costs. These consequences can have profound effects on host populations and ecosystems, underscoring the importance of understanding and mitigating parasitic interactions. 18 Parasites manipulate host behavior to their advantage: 1.Altered foraging: Changes in feeding habits to favor parasite transmission. 2.Manipulated reproduction: Parasites alter mating behavior to increase their spread. 3.Modified movement: Hosts may wander more to increase parasite dispersal. 4.Induced predator avoidance: Parasites make hosts avoid predators to prolong their own survival. 5.Social manipulation: Parasites alter social interactions to enhance transmission. 6.Circadian rhythm disruption: Parasites disrupt sleep patterns, affecting host health. Understanding these changes helps in managing parasitic interactions. 19 Mutualistic relationships, like mycorrhizal associations and rhizobia-legume symbiosis, benefit both partners by improving nutrient acquisition: 1.Mycorrhizal Associations: 1. Benefit for Plants: Fungi colonize plant roots, extending their reach into the soil for water and nutrients, especially phosphorus and nitrogen. 2. Benefit for Fungi: Plants supply fungi with sugars and carbohydrates produced through photosynthesis. 3. Outcome: Both partners gain vital resources they wouldn't access alone, leading to healthier growth for both. 2.Rhizobia-Legume Symbiosis: 1. Benefit for Legumes: Bacteria convert atmospheric nitrogen into a usable form for plants within root nodules. 2. Benefit for Rhizobia: Legumes provide bacteria with sugars and energy sources. 3. Outcome: Both partners gain nitrogen, crucial for plant growth, improving the fertility of the soil and the plant's health. In both cases, mutualism enhances nutrient acquisition for both partners, fostering healthier plants and benefiting ecosystem productivity. 20 Mutualistic interactions provide nutritional benefits to both partners: 1.Pollination Mutualism: 1. Benefit for Plants: Pollinators aid in reproduction by transferring pollen. 2. Benefit for Pollinators: Pollinators receive nectar or pollen as food. 3. Outcome: Plants reproduce, while pollinators obtain essential nutrients. 2.Gut Microbiota Mutualism: 1. Benefit for Hosts: Gut microbes aid in digestion and nutrient absorption. 2. Benefit for Microbes: Microbes receive nutrients and a habitat. 3. Outcome: Hosts improve digestion, while microbes thrive. 3.Ant-Mycorrhizal Mutualism: 1. Benefit for Plants: Ants defend plants; plants provide food. 2. Benefit for Ants: Ants receive food and shelter. 3. Outcome: Plants get protection and nutrients; ants get food and shelter. 4.Cleaning Mutualisms: 1. Benefit for Hosts: Cleaners remove parasites; hosts gain hygiene. 2. Benefit for Cleaners: Cleaners get food and protection. 3. Outcome: Hosts get cleaned; cleaners get food and safety. In each case, mutualism promotes nutrition and benefits both partners. 21 Mutualistic interactions provide defense and cleaning benefits to both partners: 1.Cleaning Mutualisms: 1. Benefit for Hosts: Larger organisms, such as fish or mammals, receive cleaning services from smaller organisms, like cleaner fish or birds, which remove parasites, dead tissue, or algae. 2. Benefit for Cleaners: Cleaners gain access to a food source and protection from predators while performing the cleaning service. 3. Outcome: Hosts benefit from parasite removal and improved hygiene, while cleaners obtain nutrition and protection. 2.Ant-Plant Mutualisms: 1. Benefit for Plants: Certain plant species form mutualistic relationships with ants, providing them with food bodies or specialized structures, such as extrafloral nectaries. 2. Benefit for Ants: Ants defend the plants against herbivores and pathogens, and in return, they receive food and shelter. 3. Outcome: Plants benefit from reduced herbivory and enhanced protection, while ants obtain nutrition and a nesting site. In both cases, mutualistic interactions contribute to defense and cleaning, enhancing the survival and well-being of the participating organisms. 22 22 Mutualistic interactions provide benefits in pollination and seed dispersal: 1.Pollination Mutualisms: 1. Benefit for Plants: Pollinators aid in reproduction by transferring pollen between flowers, facilitating fertilization and seed production. 2. Benefit for Pollinators: Pollinators receive nectar or pollen from flowers as food. 3. Outcome: Plants reproduce successfully, while pollinators obtain essential nutrients for their diet, ensuring their survival and allowing them to continue the pollination process. 2.Seed Dispersal Mutualisms: 1. Benefit for Plants: Animals, such as birds, mammals, or insects, disperse seeds away from the parent plant, promoting the establishment of new individuals. 2. Benefit for Dispersers: Animals receive food rewards, such as fruits or seeds, as they consume or transport the seeds. 3. Outcome: Plants expand their range and increase genetic diversity, while dispersers obtain nutrition and contribute to ecosystem regeneration. These mutualistic interactions play vital roles in the reproductive success and dispersal of plant species, contributing to the diversity and resilience of ecosystems. 23 The relationship between crayfish and annelid worms provides an interesting case study that blurs the line between parasitism and mutualism. Here's how: 1.Parasitic Aspect: 1. Some annelid worms, such as branchiobdellidans, are known to attach to the exoskeleton of crayfish. These worms feed on the mucus and tissues of the crayfish, potentially causing harm or discomfort to the host. 2. From the crayfish's perspective, this interaction resembles parasitism, as the worms derive nourishment from the crayfish's body without providing any apparent benefit in return. The presence of worms may lead to reduced fitness or health for the crayfish, especially if the infestation is severe. 2.Mutualistic Aspect: 1. However, recent research suggests that the relationship between crayfish and annelid worms may not be entirely parasitic. Some studies have found that branchiobdellidans may provide benefits to crayfish, such as reducing bacterial infections or deterring predators. 2. Branchiobdellidans have been observed consuming bacteria on crayfish exoskeletons, potentially reducing the risk of bacterial infections for the crayfish host. Additionally, the presence of worms may serve as a 24 deterrent to certain predators, as they may avoid consuming crayfish with visible worm infestations. 3. Context Dependence: 1. The nature of the crayfish-annelid relationship may vary depending on factors such as environmental conditions, host health, and the specific species involved. In some cases, the interaction may be more parasitic, while in others, it may exhibit mutualistic aspects. 2. Furthermore, the degree of parasitism or mutualism may fluctuate over time, influenced by factors such as the abundance of worms, host behavior, and ecological dynamics within the crayfish's habitat. Overall, the relationship between crayfish and annelid worms showcases the complexity of interspecies interactions and the challenges of categorizing them strictly as either parasitic or mutualistic. Instead, it highlights the nuanced nature of ecological relationships and the importance of considering context and variability in understanding these interactions. 24 The statement "Mutualism - Parasitism? Crayfish hosts & their Branchiobdellian annelid worm symbionts" suggests that the relationship between crayfish and their annelid worm symbionts falls within a spectrum between mutualism and parasitism. This spectrum reflects the varying outcomes of symbiotic interactions, which can range from mutually beneficial to detrimental depending on the specific context. In the case of crayfish and branchiobdellidans, the relationship appears to exhibit characteristics of both mutualism and parasitism. On one hand, the worms derive nourishment from the crayfish host, resembling parasitism. However, recent research suggests that the presence of branchiobdellidans may also confer benefits to the crayfish, such as reducing bacterial infections or deterring predators, resembling mutualism. The statement emphasizes that the outcomes of symbiotic interactions are not fixed but rather dynamic and dependent on the ever-changing context of interactions. Factors such as environmental conditions, host health, and the abundance of symbionts can influence the nature of the relationship between crayfish and branchiobdellidans. Therefore, the classification of the interaction as either mutualism or parasitism may vary over time and across different circumstances. This interpretation underscores the complexity of symbiotic relationships and highlights the need to consider the multifaceted nature of interspecies interactions in 25 ecological research. 25 Notes 1.Lotka-Volterra Predator-Prey Equations: Simulating the Lotka-Volterra PredatorPrey equations involves solving a set of coupled ordinary differential equations that describe the dynamics of predator and prey populations over time. These equations capture the interactions between predators and prey, including predation, reproduction, and natural mortality rates. While the concept of the equations is relatively straightforward, their precise solution and interpretation can be complex due to the nonlinear nature of the interactions. Implementing numerical methods to solve these equations accurately and efficiently requires a solid understanding of mathematical modeling techniques and computational skills. Additionally, interpreting the results of simulations involves analyzing population dynamics, oscillations, stability, and the effects of parameters on the dynamics of predator and prey populations. 2.Simulating a Green World with Herbivores and Predators: Simulating a green world with herbivores and predators adds another layer of complexity to the modeling process. In addition to the interactions between predators and prey, factors such as resource availability, competition among herbivores, and the influence of environmental variables on population dynamics must be considered. Modeling such a system accurately requires integrating multiple ecological processes, including nutrient cycling, primary production, and trophic interactions, into the simulation 26 framework. This entails incorporating additional equations and parameters into the model, as well as accounting for spatial heterogeneity and habitat dynamics. Furthermore, validating the simulation results against empirical data and understanding the emergent properties of the simulated ecosystem pose significant challenges. Overall, simulating a green world with herbivores and predators requires a multidisciplinary approach, combining ecological theory, mathematical modeling, and computational methods to capture the complexity of natural ecosystems accurately. 3.Symbiosis: Symbiosis refers to a close and long-term interaction between two different species. It encompasses a wide range of interactions, including mutualism, commensalism, and parasitism. 4.Mutualism - Commensalism - Parasitism - Parasitoidism: 1. Mutualism: Both species benefit from the interaction. 2. Commensalism: One species benefits while the other is neither helped nor harmed. 3. Parasitism: One species benefits at the expense of the other. 4. Parasitoidism: Similar to parasitism but typically ends with the death of the host. 5.Parasite Characteristics: 1. Hemiparasite: Partially reliant on host for nutrients. 2. Holoparasite: Completely reliant on host for nutrients. 3. Ectoparasite: Lives on the surface of the host. 4. Endoparasite: Lives inside the body of the host. 6.Parasite Transmission: 1. Vector: Organism that carries and transmits a parasite to a host. 2. Hosts: Definitive host harbors the adult parasite, while intermediate hosts may harbor larval stages. 7.Effects of Parasite Infection: Parasites can cause harm to their hosts, leading to reduced fitness, disease, or death. However, in some cases, parasitic interactions can also result in mutualistic benefits, such as enhanced nutrient acquisition or defense against pathogens. 8.The Fine Line between Parasitism and Mutualism: Crayfish & Worm Case Study: The relationship between crayfish and annelid worms illustrates the blurred boundary between parasitism and mutualism. While the worms derive nourishment from the crayfish, recent research suggests that they may also provide benefits, such as reducing bacterial infections. This case study highlights the complex nature of symbiotic interactions and the challenges of categorizing them strictly as either mutualistic or parasitic. 26